Surgeon-driven neurophysiologic monitoring in a spinal surgery population
Introduction
Spinal surgery, including correction of scoliosis and spondylolisthesis, can involve a significant risk of post-operative neurologic compromise as a result of intra-operative insults (1). The risk of neurologic complications following corrective scoliosis surgery has been estimated at between 1.5% and 9% depending on the etiology of the scoliosis (2). The efficacy of intraoperative neuro-monitoring to help overcome complications in pediatric populations undergoing spinal surgery has been established (3).
The intent of intraoperative neurophysiologic monitoring is to detect insults to the spinal cord and/or nerves before irreversible neurologic injury occurs (4). Detection of changes in cord function while correction is still possible may allow avoidance of long-term neurologic sequelae. Spinal cord monitoring is accomplished primarily by the use of somatosensory evoked potentials (SSEPs) and transcranial MEPs (tcMEPs). SSEPs are cortical or subcortical responses to repetitive electrical stimulation of a mixed peripheral nerve. Transcranial MEPs involve transcranial electrical stimulation of appropriate areas of the motor cortex to elicit descending impulses travelling down the corticospinal tract (4).
With both SSEPs and tcMEPs, baseline values are obtained at the beginning of the surgical intervention. Decreased amplitude by more than 10% or decreased latency of greater than 50% are felt to be indicative of potential deficit with SSEP monitoring, while a 75% decrease in CMAP amplitude is significant in tcMEP monitoring (4,5). These criteria, however, have not been universally established, and cutoff values remain controversial. Nonetheless, the use of concurrent SSEP and tcMEP monitoring is becoming best practice (6,7).
A worldwide shortage of adequately trained and qualified personnel, particularly technologists and physiologists, to perform and interpret the results of intraoperative neuro-monitoring (1,4) has led to the development of surgeon-driven neuro-monitoring systems. At the authors’ institution, one such system (NeuroVision®, NuVasive® Inc., San Diego, CA, USA) has been in use for 8 years. This system interfaces directly with the surgeon throughout the operative procedure, providing tcMEP and CMAP waveform information in visible and audible feedback. The fact that the surgeon operates and interprets this neuro-monitoring system means that it can be employed even when a technologist is not available. The purpose of this study was to evaluate the use of this surgeon-driven neuro-monitoring system in a pediatric population undergoing corrective spinal surgery, with the hypothesis that a surgeon-driven system would provide a safe alternative to standard technologist-operated neuro-monitoring system in a spinal surgery population.
Methods
One hundred thirty-five patients undergoing a variety of spinal procedures were monitored intra-operatively using a surgeon-driven neuro-monitoring system over an 80-month period. Patients underwent informed consent if they were less than 50 years old and were scheduled for a planned spinal procedure with potential neurologic complications. Monitoring was used in all cases which technically allowed for Cranial MEP’s. Exclusion criteria were patients with active uncontrolled seizures and muscular dystrophy, prior cranial surgery, skull deformity or metallic implant in the head, implanted stimulator, and any pre-existing conditions that prohibit the effective use of intra-operative motor pathway monitoring (e.g., paraplegia). All cases were monitored by the surgeon.
Surgical procedures
A variety of operative procedures were carried out (Table 1), including posterior segmental instrumentation for scoliosis, decompression and fusion for spondylolisthesis, and single-level vertebral column resection and anterior segmental fusion. In addition, intraoperative traction with Gardner-Wells tongs and femoral skeletal traction were used.
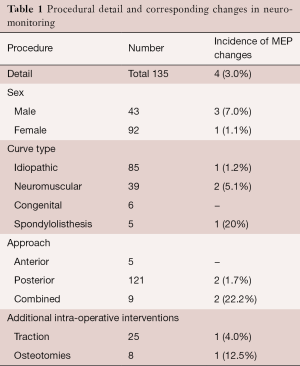
Full table
Monitoring procedures
Figure 1 is a screen capture of the neuro-monitoring system display. It demonstrates tcMEP stimulation thresholds in mA for each myotome monitored, and corresponding CMAP waveforms. Baseline thresholds are continuously displayed with the waveform; subsequent test thresholds are displayed in the adjacent boxes and color-coded to reflect significant changes from baseline (e.g., >100 mA increase = yellow, >200 mA increase = red), and provided to the surgeon-user in this simplified format both visibly and audibly.
The operating surgeon who was trained in the application of the neurophysiologic monitoring system applied transcutaneous stimulation and recording leads after induction. Subsequent to induction of general anaesthesia, bilateral transcranial electrodes were placed by the operative staff anterior to the coronal suture in order to stimulate the motor cortex. Intramuscular leads were placed in both upper and lower extremities, including abductor pollicis (C6,7,8,T1), vastus medialis (L2,3,4), tibialis anterior (L4,5), and gastrocnemius (S1,2). These leads provided both EMG and tcMEP data. Prior to beginning the operative procedure, baseline MEP recordings were obtained to ensure both normal stimulation thresholds. A 75% increase in threshold was considered significant.
An attempt was made to avoid usage of certain anesthetic agents including benzodiazepines, nitrous oxide, and halogenated agents. Care was also taken to avoid hypotension or hypothermia. If any of these factors were present during abnormal tcMEP results, they were corrected.
Throughout the procedure, repeat stimulation was carried out and new values and waveforms were compared to baseline. Particular attention was paid during and after reduction manoeuvres. Significant changes in MEP recordings alerted the surgeon automatically and were documented. If monitoring changes influenced surgical intervention, this was also documented, along with any resolution of monitoring abnormalities due to corrective intervention. These intraoperative findings were compared with the postoperative neurological status of each patient.
Post-operative neurologic deficit was defined as a clinically detectable change in either motor or sensory function compared to pre-operative status, as determined by clinical exam both immediately post-operatively and in follow-up clinic visits. This study was reviewed for ethics compliance by the Queen’s University Health Sciences and Affiliated Teaching Hospitals Research Ethics Board.
Results
Intra-operative adjustments based on neuro-monitoring data were made in four cases or 3% of the cohort. The first occurred in a posterior decompression and fusion of a grade IV L5-S1 spondylolytic spondylolisthesis in a 17-year-old male. During the reduction manoeuvre, tcMEP stimulation threshold in the tibialis anterior lead increased from a baseline of 500 mA to greater than 900 mA. In addition, spontaneous EMG readings of tibialis anterior increased significantly. The reduction was consequently abandoned, with a corresponding normalisation of tcMEP to 560 mA. The patient’s spondylolisthesis was fused in situ without a reduction, and he demonstrated no post-operative neurologic deficit.
The second case involved combined anterior and posterior instrumentation of a 14-year-old male with neurofibromatosis and 90-degree kyphoscoliosis. Intra-operatively, increased tcMEP values were noted in the tibialis anterior leads on two separate occasions, both independent of instrumentation or reduction manoeuvres. His baseline tcMEP value of 260 mA increased to 460 mA during the procedure. Given intra-operative blood loss and relative hypotension (blood pressure 80/50 mmHg) at the time of tcMEP measurement, the increase was felt to be secondary to spinal cord hypoperfusion. One unit of packed red blood cells (PRBCs) was administered, resulting in relative normalisation of tcMEP threshold to 300 mA. At the end of the same procedure, during closure of the incision, tcMEP threshold increased to 420 mA. This was again felt to be secondary to cord hypoperfusion, and a second unit of PRBCs was transfused. Postoperatively, the patient had no new deficit in his neurologic status, which had been significantly impaired pre-operatively due to his deformity and underlying disease.
The third case involved a 44-year-old female with idiopathic scoliosis undergoing posterior instrumentation. Correction was achieved using 40 pounds of traction to the head and legs. During exposure, prior to placement of pedicle screws, tcMEP values increased from a baseline of 460 mA to over 900 mA. Traction was decreased to 20 pounds on the head and legs and tcMEPs normalized to 460 mA. The patient underwent correction and demonstrated no post-operative neurological deficits.
The fourth case involved a posterior instrumentation with intra-operative osteotomies in a 14-year-old male with proteus syndrome. Correction and instrumentation were carried out without concern. While closing, tcMEP in tibialis anterior were lost bilaterally. His planned post-operative course was to remain intubated in ICU for a day, however given the changes in neuro-monitoring, a wake-up test was carried out and the patient had no motor function in his lower extremities. Posterior stabilization rods were subsequently removed and the neurologic function returned to normal by one week post-operatively.
The remaining 130 patients (97.0%) exhibited no significant abnormalities in intra-operative monitoring throughout their respective procedures. There were no documented post-operative neurologic abnormalities that differed from pre-operative baseline in any of the patients in this series.
Discussion
There is increasing evidence that intraoperative neuro-monitoring is safe and effective in both adult and pediatric populations (3). As a result, neuro-monitoring is becoming best practice for pediatric spinal deformity surgery (1). A significant barrier to more widespread use of intraoperative neuro-monitoring is a lack of adequately trained and certified technologists and physiologists to operate neuro-monitoring systems and interpret the results (1,4). One potential solution to this problem is the use of a system which is both operated and interpreted by the surgeon. Such a surgeon-driven system potentially allows for the benefits of intraoperative neuro-monitoring when personnel resources are scarce. To the best of the authors’ knowledge, there have been no reports in the literature reporting on the use of a surgeon-driven neuro-monitoring system in a pediatric spinal deformity population.
In this series, four episodes (3%) in a cohort 135 patients of potential neurologic compromise were identified intra-operatively. No post-operative neurologic deficits were identified, supporting the hypothesis that this surgeon-driven system was safe and effective in this spinal deformity population.
There are several factors that must be taken into consideration when performing intraoperative neuro-monitoring. As described elsewhere, inhalational anaesthetics have a significant effect on SSEP values, and should be avoided when neuro-monitoring is being performed. At high doses, propofol may suppress tcMEPs, and ketamine can be used to lower the propofol dose necessary to achieve adequate anaesthesia. In addition, factors such as hypotension, hypothermia, and decreased haematocrit, carbon dioxide and oxygen may alter neurophysiologic data (8). This was evidenced in this study’s second case, where an increase in tcMEP stimulation threshold was felt to be secondary to hypotension and cord hypoperfusion. Adequate communication between all members of the operative team, including surgeons, anesthetists and nurses is essential.
This study has some limitations. The neuro-monitoring system used measured tcMEPs and CMAP waveforms only, while the combined neuro-monitoring techniques in recent literature also include SSEPs (9). The newer generation system includes SSEP capability as well. The utility of combined neuro-monitoring, even in patients with neuromuscular disorders such as cerebral palsy, has been established (10). In addition, SSEP monitoring may be of benefit for patients with Duchenne muscular dystrophy, where early muscle fatigue prevents usual stimulation with “train of four” impulses and establishment of baseline tcMEP values.
This study is subject to the biases inherent in non-randomized studies. Given that intra-operative neuro-monitoring is best practice at our institution, however, and that its efficacy in predicting and preventing postoperative neurologic deficit has been well established, it was not felt to be ethical to propose a randomised trial which would expose the control population to a higher risk of postoperative deficit. Secondly, the numbers reported are relatively small. Nevertheless, this is the only known report on the safety and efficacy of surgeon-driven intraoperative neuro-monitoring in a spinal deformity population to date.
In conclusion, none of the patients in this cohort experienced postoperative neurologic deficit. Four patients had intra-operative adjustments made to their surgical or anaesthetic technique based on neuro-monitoring results. Neuro-monitoring capabilities allowed the surgeon to perform more aggressive reduction or correction manoeuvres with confidence, knowing that impending spinal cord compromise could be detected while correction was still possible, before deficits became permanent. Although rare, changes in intraoperative monitoring and associated neurologic injuries are catastrophic. Preventing even one paralysis is of value, but not always possible when/where neuro-monitoring resources are unavailable. Surgeon-driven neuro-monitoring was safe and effective for surgery in this spinal deformity population, despite the lack of a technologist or physiologist. This technique should be considered in centres where additional trained personnel are not available for monitoring.
Acknowledgements
The authors would like to thank Heather Grant for her assistance with this manuscript.
Footnote
Conflicts of Interest: The authors have no conflicts of interest to declare.
Ethical Statement: This study was reviewed for ethics compliance by the Queen’s University Health Sciences and Affiliated Teaching Hospitals Research Ethics Board (SURG-181-08), and written informed consent was obtained from all patients.
References
- Norton J, Hedden D. Canadian Paediatric Spinal Deformity Study Group. Neuromonitoring during surgery for paediatric spinal deformity in Canada (2007). Can J Neurol Sci 2009;36:47-50. [Crossref] [PubMed]
- Weiss HR, Goodall D. Rate of complications in scoliosis surgery - a systematic review of the Pub Med literature. Scoliosis 2008;3:9. [Crossref] [PubMed]
- Wilson-Holden TJ, Padberg AM, Lenke LG, et al. Efficacy of intraoperative monitoring for pediatric patients with spinal cord pathology undergoing spinal deformity surgery. Spine (Phila Pa 1976) 1999;24:1685-92. [Crossref] [PubMed]
- Devlin VJ, Schwartz DM. Intraoperative neurophysiologic monitoring during spinal surgery. J Am Acad Orthop Surg 2007;15:549-60. [Crossref] [PubMed]
- Gonzalez AA, Jeyanandarajan D, Hansen C, et al. Intraoperative neurophysiological monitoring during spine surgery: a review. Neurosurg Focus 2009;27:E6. [Crossref] [PubMed]
- Nuwer MR, Dawson EG, Carlson LG, et al. Somatosensory evoked potential spinal cord monitoring reduces neurologic deficits after scoliosis surgery: results of a large multicenter survey. Electroencephalogr Clin Neurophysiol 1995;96:6-11. [Crossref] [PubMed]
- Legatt AD. Current practice of motor evoked potential monitoring: results of a survey. J Clin Neurophysiol. 2002;19:454-60. [Crossref] [PubMed]
- Sloan T. Anesthesia and intraoperative neurophysiological monitoring in children. Childs Nerv Syst 2010;26:227-35. [Crossref] [PubMed]
- Quraishi NA, Lewis SJ, Kelleher MO, et al. Intraoperative multimodality monitoring in adult spinal deformity: analysis of a prospective series of one hundred two cases with independent evaluation. Spine (Phila Pa 1976) 2009;34:1504-12. [Crossref] [PubMed]
- DiCindio S, Theroux M, Shah S, et al. Multimodality monitoring of transcranial electric motor and somatosensory-evoked potentials during surgical correction of spinal deformity in patients with cerebral palsy and other neuromuscular disorders. Spine (Phila Pa 1976) 2003;28:1851-5; discussion 1855-6.
Contributions: (I) Conception and design: All authors; (II) Administrative support: Heather Grant; (III) Provision of study materials or patients: DP Borschneck; (IV) Collection and assembly of data: DP Borschneck; (V) Data analysis and interpretation: All authors; (VI) Manuscript writing: All authors; (VII) Final approval of manuscript: All authors.