Single-level selective dorsal rhizotomy for spastic cerebral palsy
Introduction
Selective dorsal rhizotomy (SDR) has been practiced in many guises for over 100 years (1). Currently it is most commonly performed for the treatment of spastic cerebral palsy (CP) in children. CP affects approximately 2–3 in 1,000 people in developed nations (2-4), but the prevalence and severity of CP in developed nations may be decreasing due to more effective perinatal care (5). By comparison there is an approximately threefold higher incidence in developing nations due to poorer antenatal, perinatal and postnatal care and facilities (2). Bilateral spastic CP is the most common subtype of CP in Europe, accounting for over half of the patients (6).
CP encompasses a wide range of non-progressive neurological disability that originates in the developing foetal or infant brain (7). CP can be classified according to movement disorder type (spastic, dyskinetic, ataxic or mixed) and comorbidities such as dysphagia, sialorrhoea, gastro-oesophageal reflux disease (GORD), epilepsy, and neuropsychological problems may occur (7). Of these, spastic CP is the most common (2) and is further sub-classified in relation to the topographical distribution of the disease (7). Gross motor function in CP is often classified using Gross Motor Function Classification System (GMFCS) (8):
- Level I: walks well in all settings. Balance and speed may be limited compared with children developing normally;
- Level II: walks in most settings, but may have difficulty walking long distances or with balance. May utilise personal or environmental mobility aids to climb stairs;
- Level III: walks with the use of hand-held mobility aids such as K-walkers in most indoor settings. Uses wheeled mobility for longer distance travel;
- Level IV: utilises wheeled mobility aids in most settings (either attendant-propelled or powered) and requires assistance to transfer;
- Level V: transported in wheelchairs in all settings and has limited to no antigravity head, trunk and limb control.
The pathophysiology of CP involves a disturbance of brain development that occurs during pregnancy, around the time of birth, or early infancy. In recent years more genetic conditions have been identified which can result in either brain injury or malformation, such as COL4a mutation and early ischaemic stroke, LIS1 gene and lissencephaly and GPR56 gene and polymicrogyria. The periventricular white matter is particularly susceptible to injury in the prenatal period from 26 to 34 weeks (9). Consequently over 70% of patients with bilateral spastic CP exhibit periventricular leukomalacia (PVL) on MRI (10). Such involvement compromises inputs from the precentral gyrus through the internal capsule to the spinal motor neurons. Abnormal inputs to the vestibular and reticular nuclei or their tracts results in loss of inhibitory influences to the spinal motor neurons, resulting in aberrant reflex arcs. This increases tone; in particular, damage to the vestibulospinal tracts increases extensor tone (9).
The management of spastic CP should be integrated and multidisciplinary with a focus on altering the natural history of the disease (1). It may include a combination of physical and pharmacological therapy, as well as surgical interventions:
- Therapy interventions are first line and include occupational therapy, physiotherapy and speech therapy. Their aim is often to improve functional independence and/or decrease caregiver burden. This includes the provision of orthotics and equipment to aid mobility and function;
- Pharmacological interventions include oral medication (such as diazepam or baclofen) intramuscular botulinum toxin A injections, and phenol nerve blocks. Pharmacological interventions target the spasticity and its complications;
- Surgical interventions include neurosurgical techniques that target the spasticity [intrathecal baclofen (ITB) pump or SDR] and orthopedic surgery to release contractures and manage torsional bony deformities.
Most centres will offer conservative and pharmacological interventions combined with orthopedic surgery as contractures arise. Neurosurgical techniques require specialist referral and are performed in relatively fewer centres. For instance, SDR is currently performed in two centres in Australia and five centres in the UK.
SDR technique
SDR targets the aberrant reflex arcs in spastic CP. Today SDR is commonly performed as a single level procedure at the conus (11) combined with Fasano’s intraoperative electromyography (EMG) technique (1), as shown in Figure 1. In Australia, an open laminoplasty technique is typically used currently. Concerns about the potential impact of extensive spinal surgery led Park to develop his single level technique (11).
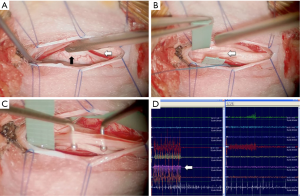
There is very little research comparing the two approaches, but O’Brien and Park have reported the need for less post-operative spinal stabilisation after introducing the single-level technique (12). In a retrospective case series comparing single-level laminectomy with multi-level laminectomy, Ou et al. found that length of hospital stay was the only significant difference between the two approaches (13). They found no differences in pain or time to mobilisation following surgery and also note that there are similar gains in function at one-year post-surgery regardless of whether a single- or multi-level technique is used.
The single-level approach is technically more difficult than the multi-level approach and is described in detail by Park and Johnston (11). The conus and L2 to S2 nerve roots are exposed through a single level laminotomy at the level of the conus, which is identified using ultrasound guidance. Afferent nerve rootlets are systematically stimulated using intraoperative EMG and graded according to how diffuse the response to the stimulation is. Rootlets with responses beyond their myotome distribution are selectively divided, with approximately 60–70% of rootlets undergoing selective division.
Patient assessment for SDR
Patient workup for SDR is variable and has not been widely validated. Selection is typically based on clinical rationale rather than scientific evidence (14). While there is no restriction in age, evidence shows there is likely to be less benefit in patients with bilateral spastic CP undergoing SDR after the age of ten years (15) and patients with intellectual disability are likely to have a poorer prognosis (16). This raises the importance of quality selection algorithms for patients with bilateral spastic CP, the impact of which has been reported in a number of retrospective reviews (17-19).
Standard selection criteria for SDR are based on the Peacock criteria (1):
- History:
- No restriction in age, typically over the age of three;
- At least six months after the last botulinum toxin A injection;
- At least one year after orthopedic surgery;
- Cognitive and emotional ability to cope with intensive rehabilitation process;
- Supportive home environment;
- Access to rehabilitation facilities.
- Examination:
- Spastic diplegia with no significant ataxia or dystonia;
- A degree of ambulation with or without assistive devices, typically GMFCS grade II or III;
- Good trunk control and good lower extremity antigravity strength on clinical examination.
- No significant scoliosis.
- Investigations:
- PVL on MRI with no involvement of the thalamus, basal ganglia or cerebellum;
- Reimers index <40%, ie no significant femoral head subluxation on pelvic radiograph.
The Oswestry selection criteria are more stringent than the Peacock criteria but have a more orthopedic focus than most centres (17). Their selection criteria involved a complex multi-disciplinary assessment that included clinical examination, spine and hip radiographs, gait analysis, EMG, and MRI of the brain and spinal cord. Only 35% of referred children satisfied the Oswestry selection criteria and underwent SDR (17). While patients are generally only considered if they are GMFCS grade II or III, some recent evidence suggests patients with GMFCS grade IV or V could benefit from SDR (20), given appropriate goals for the procedure. Due to the aetiology of CP in children with GMFCS grade IV and V, they are more likely to have a mixed pattern of hypertonia, including a combination of spasticity and dystonia; the latter is not improved with SDR. While stringent selection criteria aim to maximise outcomes, patients who would normally be excluded from the Peacock criteria may still benefit from SDR.
Rehabilitation from SDR
The aim of SDR combined with PT is to maintain function while reducing spasticity, with the combination of treatment almost doubling the gains that PT alone can achieve (21). Formal assessments typically occur at 6, 12 and 24 months post-SDR. This allows the goals of rehabilitation to be modified according to the patient’s gains. As patients regain skills over the course of rehabilitation, therapy transitions towards fitness activities.
Early recovery from single-level laminotomy SDR is usually fast, with patients commencing PT by day 3 or 4 post-SDR (22). Ongoing PT is required for up to 2 years post-SDR, but intensive daily PT is required in the initial 2-3 weeks (22). During this period, reduced sensory feedback limits the ability of patients to maintain movement control rather than post-surgical weakness. By eliminating spasticity SDR unmasks underlying weakness which may sometimes be underestimated by those involved in the child’s care. The rate at which patients recover muscle control depends on their pre-surgical GMFCS level; GMFCS level II patients typically regain some degree of walking within 3 weeks. Orthotics are also used to help patients gain stability and alignment, and additional postural support may be required.
The most rapid gains usually occur within the first 3 to 6 months post-SDR, and therapeutic aims are to develop motor learning combined with musculoskeletal change (22). Over this period, the goals of PT include movement control, resistance strengthening, fitness and endurance. This is achieved through repetitive practice combined with motivational goals, which requires a significant investment from patients and their families. Orthotics will also require modifications over this period in order to optimise these gains.
However, it must be kept in mind that the motor deficit in CP is complex. The reduction in spasticity produced by SDR may unmask other movement disorders such as dystonia and dyskinesia or uncover deficits in strength or selective motor control. Carefully targeted adjuvant medical therapy, such as botulinum toxin injections or trihexyphenidyl may be required to target dystonia. This complexity highlights the need for integrated multidisciplinary care to continue throughout rehabilitation and beyond.
Outcome of SDR
The evidence from randomised control trials suggests that functional gains from SDR are likely to be moderate (1,23). McLaughlin et al.’s meta-analysis of three randomised trials found that GMFM and Ashworth scores both significantly improved following SDR combined with PT compared with PT alone (21). There was also a direct linear correlation between the number of dorsal roots divided and the gains in GMFM. This was an important finding as it explained the poorer outcome in the Seattle group (24), which divided a mean of 25% of the dorsal roots rather than the currently accepted technique of dividing 50–75%. These improvements in GMFM have not always been shown to translate to gains in social participation (23). Worse gross motor function is correlated with poorer outcome (6,7). Nevertheless, where spasticity has been shown by careful examination to confer a significant impediment to their motor progress, children with worse gross motor function still improve once their spasticity has been reduced by SDR (25).
The natural history of CP at GMFCS levels III-V involves decline in GMFM (26) during adolescence, suggesting timing of surgery and understanding the long term outcomes of SDR are important. Evidence of long term outcomes of SDR is emerging. Generally, studies have been positive, with negative outcomes contributing to the evidence base for selection criteria (1). Studies with at least 5 years follow up consistently show that the reductions in spasticity are maintained in the long term (27-31). Peacock’s original cohort has now been followed up for over 20 years and it is evident that the benefits they gained as children are still present (32). Nordmark et al. and Josenby et al. found that patients also had improvements in their Paediatric Evaluation of Disability Inventory (PEDI) scores, notably in the functional skills and mobility domains (27,31). Dudley et al. found that these gains persisted to 15 years post-SDR (29). The multidimensional benefits of SDR were evident from the PEDI score results, where significant gains in self-care and mobility persisted through to early adulthood. Moreover, SDR patients in this study compared with children who did not undergo SDR had a significant reduction in the need for further orthopedic intervention (29). Tedroff et al. on the other hand found that, while spasticity was significantly reduced by SDR at 15–20 years post-SDR, contractures were not prevented and long-term functioning was not improved (28). Novak et al.’s review concluded that these potential benefits should not be the primary goal of SDR (23).
Less satisfactory long-term outcomes have been reported, which contribute to decisions on patient selection. Poorer outcomes have been reported in children with spastic quadriplegia compared to those with spastic diplegia (16). Children over ten years of age have been reported to have better long-term outcomes with multi-level orthopedic surgery than with SDR (15). In one study of children who were mostly GMFCS level IV, there was no significant benefit of SDR combined with physiotherapy compared with physiotherapy alone (33). More recently Ingale et al. have suggested that SDR was more effective at reducing spasticity than ITB in children (GMFCS 4-5) requiring ITB pump replacement (20). ITB pump has other advantages over SDR in that it is reversible and is effective at treating any concurrent dystonia, which is more likely to be found in patients with GMFCS level IV or V. Ingale et al. suggested that SDR could be a viable option for patients with pump infections or who require a battery change (20). There is general agreement that good long-term results can be achieved with young children with spastic diplegia who function at GMFCS level II-III (1).
The evidence supports the use of SDR to address spasticity (23), but it is important to establish realistic goals for a patient undergoing SDR (1). While GMFCS correlates with physical well-being, it does not correlate with QOL measures in patients with CP (34). Patients are however likely to report improvements in activities of daily living following SDR, are likely to recommend SDR to other patients with CP, and are unlikely to report negative impressions of the procedure (35). This has obvious implications for counselling and may require tailoring throughout recovery to suit the changing needs of the patient and their family as well as to reflect the gains of the patient.
Possible operative complications include infection, haemorrhage and leak of cerebrospinal fluid (CSF). Permanent complications are rare (1). Judicious intraoperative EMG monitoring is a widely performed and evidence-based approach and used to mitigate against severe lower limb weakness and incontinence (36). The literature suggests that in the long term, patients may experience increased lordosis and hip subluxation (6,32,37,38), but there is insufficient evidence to attribute the complications to SDR or the natural history of CP (30). However, the single-level laminectomy aims to significantly reduce the likelihood and severity of these complications (11).
Cost-effectiveness of SDR
There are no studies evaluating the health economics of SDR in children with spastic diplegia. Without such studies it is challenging to evaluate the cost-effectiveness of SDR as the various treatment options are difficult to compare. Most children at GMFCS levels II-IV who would be potentially suitable for SDR undergo multiple botulinum toxin injections and at least one episode of multi-level orthopedic surgery. However patients who undergo SDR may have significant reductions in the need for further orthopedic surgery and botulinum toxin A injections (23,39,40).
Based on our experience managing CP, children with spastic diplegia often require botulinum toxin A injections on a regular basis, from approximately 3 years of age onwards. Injections are administered usually under oral sedation followed by intensive physiotherapy and sometimes serial casting. The effect in some is short lived and rarely persists beyond 6 to 9 months. Many children require repeated injections, which also become less effective on repeated administration as children get older. SDR has the potential to replace these cumulative costs and decreasing effect.
Children with spastic diplegia accumulate progressive lower limb skeletal, muscular and joint deformities before reaching skeletal maturity. This frequently necessitates multi-level orthopedic surgery, which often includes soft tissue release surgery in combination with femoral osteotomies and hip reconstruction. It is probable that all patients who undergo SDR would need to have orthopedic procedures at least once before they reach skeletal maturity had SDR not been performed (41). Those undergoing SDR at a young age have the lowest requirement for orthopedic surgery after SDR, which may have a potential cost benefit.
Conclusions
An integrated, multidisciplinary approach is required to effectively treat spastic CP and not all patients are suitable for SDR. SDR combined with intensive PT is an effective treatment for spastic CP. Patients require up to 2 years’ rehabilitation, and the goals of that rehabilitation must adapt to the gains of the patient. Patients are unlikely to improve their GMFCS level but are likely to show a significant reduction in spasticity and improvement in GMFM scores. This reduces their reliance on further surgical interventions and improves their independence in ADL and QOL. However significant improvements in spasticity may unmask underlying dyskinesia, which may in turn require integrated medical therapy. As it is practiced today, SDR has very few orthopedic complications such as lordosis and hip subluxation, but the natural history of CP may have a larger contribution to these adverse outcomes. While the cost-effectiveness of SDR has not been adequately assessed, it is likely that SDR is a cost-effective intervention for CP.
Acknowledgements
None.
Footnote
Conflicts of Interest: The authors have no conflicts of interest to declare.
References
- Aquilina K, Graham D, Wimalasundera N. Selective dorsal rhizotomy: an old treatment re-emerging. Arch Dis Child 2015;100:798-802. [Crossref] [PubMed]
- Odding E, Roebroeck ME, Stam HJ. The epidemiology of cerebral palsy: incidence, impairments and risk factors. Disabil Rehabil 2006;28:183-91. [Crossref] [PubMed]
- Hirtz D, Thurman DJ, Gwinn-Hardy K, et al. How common are the "common" neurologic disorders? Neurology 2007;68:326-37. [Crossref] [PubMed]
- Jacobsson B, Hagberg G. Antenatal risk factors for cerebral palsy. Best Pract Res Clin Obstet Gynaecol 2004;18:425-36. [Crossref] [PubMed]
- Reid SM, Meehan E, McIntyre S, et al. Temporal trends in cerebral palsy by impairment severity and birth gestation. Dev Med Child Neurol 2016;58 Suppl 2:25-35. [Crossref] [PubMed]
- Bolster EA, van Schie PE, Becher JG, et al. Long-term effect of selective dorsal rhizotomy on gross motor function in ambulant children with spastic bilateral cerebral palsy, compared with reference centiles. Dev Med Child Neurol 2013;55:610-6. [Crossref] [PubMed]
- Rosenbaum PL, Walter SD, Hanna SE, et al. Prognosis for gross motor function in cerebral palsy: creation of motor development curves. JAMA 2002;288:1357-63. [Crossref] [PubMed]
- Palisano R, Rosenbaum P, Walter S, et al. Development and reliability of a system to classify gross motor function in children with cerebral palsy. Dev Med Child Neurol 1997;39:214-23. [Crossref] [PubMed]
- Koman LA, Smith BP, Shilt JS. Cerebral palsy. Lancet 2004;363:1619-31. [Crossref] [PubMed]
- Bax M, Tydeman C, Flodmark O. Clinical and MRI correlates of cerebral palsy: the European Cerebral Palsy Study. JAMA 2006;296:1602-8. [Crossref] [PubMed]
- Park TS, Johnston JM. Surgical techniques of selective dorsal rhizotomy for spastic cerebral palsy. Technical note. Neurosurg Focus 2006;21:e7. [Crossref] [PubMed]
- O'Brien DF, Park TS. A review of orthopedic surgeries after selective dorsal rhizotomy. Neurosurg Focus 2006;21:e2. [Crossref] [PubMed]
- Ou C, Kent S, Miller S, et al. Selective dorsal rhizotomy in children: comparison of outcomes after single-level versus multi-level laminectomy technique. Can J Neurosci Nurs 2010;32:17-24. [PubMed]
- Grunt S, Fieggen AG, Vermeulen RJ, et al. Selection criteria for selective dorsal rhizotomy in children with spastic cerebral palsy: a systematic review of the literature. Dev Med Child Neurol 2014;56:302-12. [Crossref] [PubMed]
- MacWilliams BA, Johnson BA, Shuckra AL, et al. Functional decline in children undergoing selective dorsal rhizotomy after age 10. Dev Med Child Neurol 2011;53:717-23. [Crossref] [PubMed]
- Kim HS, Steinbok P, Wickenheiser D. Predictors of poor outcome after selective dorsal rhizotomy in treatment of spastic cerebral palsy. Childs Nerv Syst 2006;22:60-6. [Crossref] [PubMed]
- Cole GF, Farmer SE, Roberts A, et al. Selective dorsal rhizotomy for children with cerebral palsy: the Oswestry experience. Arch Dis Child 2007;92:781-5. [Crossref] [PubMed]
- Morota N. Functional posterior rhizotomy: the Tokyo experience. Childs Nerv Syst 2007;23:1007-14. [Crossref] [PubMed]
- Langerak NG, Lamberts RP, Fieggen AG, et al. Selective dorsal rhizotomy: long-term experience from Cape Town. Childs Nerv Syst 2007;23:1003-6. [Crossref] [PubMed]
- Ingale H, Ughratdar I, Muquit S, et al. Selective dorsal rhizotomy as an alternative to intrathecal baclofen pump replacement in GMFCS grades 4 and 5 children. Childs Nerv Syst 2016;32:321-5. [Crossref] [PubMed]
- McLaughlin J, Bjornson K, Temkin N, et al. Selective dorsal rhizotomy: meta-analysis of three randomized controlled trials. Dev Med Child Neurol 2002;44:17-25. [Crossref] [PubMed]
- Cawker S, Aquilina K. Selective dorsal rhizotomy (the perspective of the neurosurgeon and physiotherapist). Paediatrics and Child Health 2016. [Epub ahead of print]. [Crossref]
- Novak I, McIntyre S, Morgan C, et al. A systematic review of interventions for children with cerebral palsy: state of the evidence. Dev Med Child Neurol 2013;55:885-910. [Crossref] [PubMed]
- McLaughlin JF, Bjornson KF, Astley SJ, et al. Selective dorsal rhizotomy: efficacy and safety in an investigator-masked randomized clinical trial. Dev Med Child Neurol 1998;40:220-32. [Crossref] [PubMed]
- Kan P, Gooch J, Amini A, et al. Surgical treatment of spasticity in children: comparison of selective dorsal rhizotomy and intrathecal baclofen pump implantation. Childs Nerv Syst 2008;24:239-43. [Crossref] [PubMed]
- Hanna SE, Rosenbaum PL, Bartlett DJ, et al. Stability and decline in gross motor function among children and youth with cerebral palsy aged 2 to 21 years. Dev Med Child Neurol 2009;51:295-302. [Crossref] [PubMed]
- Josenby AL, Wagner P, Jarnlo GB, et al. Functional performance in self-care and mobility after selective dorsal rhizotomy: a 10-year practice-based follow-up study. Dev Med Child Neurol 2015;57:286-93. [Crossref] [PubMed]
- Tedroff K, Löwing K, Åström E. A prospective cohort study investigating gross motor function, pain, and health-related quality of life 17 years after selective dorsal rhizotomy in cerebral palsy. Dev Med Child Neurol 2015;57:484-90. [Crossref] [PubMed]
- Dudley RW, Parolin M, Gagnon B, et al. Long-term functional benefits of selective dorsal rhizotomy for spastic cerebral palsy. J Neurosurg Pediatr 2013;12:142-50. [Crossref] [PubMed]
- Grunt S, Becher JG, Vermeulen RJ. Long-term outcome and adverse effects of selective dorsal rhizotomy in children with cerebral palsy: a systematic review. Dev Med Child Neurol 2011;53:490-8. [Crossref] [PubMed]
- Nordmark E, Josenby AL, Lagergren J, et al. Long-term outcomes five years after selective dorsal rhizotomy. BMC Pediatr 2008;8:54. [Crossref] [PubMed]
- Langerak NG, Lamberts RP, Fieggen AG, et al. A prospective gait analysis study in patients with diplegic cerebral palsy 20 years after selective dorsal rhizotomy. J Neurosurg Pediatr 2008;1:180-6. [Crossref] [PubMed]
- Mäenpää H, Salokorpi T, Jaakkola R, et al. Follow-up of children with cerebral palsy after selective posterior rhizotomy with intensive physiotherapy or physiotherapy alone. Neuropediatrics 2003;34:67-71. [Crossref] [PubMed]
- Livingston MH, Rosenbaum PL, Russell DJ, et al. Quality of life among adolescents with cerebral palsy: what does the literature tell us? Dev Med Child Neurol 2007;49:225-31. [Crossref] [PubMed]
- Hurvitz EA, Marciniak CM, Daunter AK, et al. Functional outcomes of childhood dorsal rhizotomy in adults and adolescents with cerebral palsy. J Neurosurg Pediatr 2013;11:380-8. [Crossref] [PubMed]
- Fukuhara T, Nakatsu D, Namba Y, et al. Histological evidence of intraoperative monitoring efficacy in selective dorsal rhizotomy. Childs Nerv Syst 2011;27:1453-8. [Crossref] [PubMed]
- Li Z, Zhu J, Liu X. Deformity of lumbar spine after selective dorsal rhizotomy for spastic cerebral palsy. Microsurgery 2008;28:10-2. [Crossref] [PubMed]
- Johnson MB, Goldstein L, Thomas SS, et al. Spinal deformity after selective dorsal rhizotomy in ambulatory patients with cerebral palsy. J Pediatr Orthop 2004;24:529-36. [Crossref] [PubMed]
- Wong AM, Pei YC, Lui TN, et al. Comparison between botulinum toxin type A injection and selective posterior rhizotomy in improving gait performance in children with cerebral palsy. J Neurosurg 2005;102:385-9. [PubMed]
- Chicoine MR, Park TS, Kaufman BA. Selective dorsal rhizotomy and rates of orthopedic surgery in children with spastic cerebral palsy. J Neurosurg 1997;86:34-9. [Crossref] [PubMed]
- Steinbok P. Outcomes after selective dorsal rhizotomy for spastic cerebral palsy. Childs Nerv Syst 2001;17:1-18. [Crossref] [PubMed]
Contributions: (I) Conception and design: D Graham; (II) Administrative support: None; (III) Provision of study materials or patients: None; (IV) Collection and assembly of data: K Aquilina; (V) Data analysis and interpretation: S Cawker, S Paget, N Wimalasundera; (VI) Manuscript writing: All authors; (VII) Final approval of manuscript: All authors.