Range of motion, sacral screw and rod strain in long posterior spinal constructs: a biomechanical comparison between S2 alar iliac screws with traditional fixation strategies
Introduction
Whilst fusion at the lumbosacral joint provides an extra point of stability in long fusions, often large excessive forces are placed on this joint resulting in significant non-union pseudarthrosis and construct failure for some such patients (1,2). Lumbosacral non-union rate has been shown to increase with fusions from L3 and above down to the sacrum (3). Several options for reducing the motion and screw strain at the lumbosacral junction have been proposed to reduce the risk of construct failure and non-union, including S2 Alar Iliac (S2AI), Galveston technique, or supplemental fixation via the sacroiliac joint (4). Additional anterior column support can be provided via anterior lumbar interbody fusion (ALIF), transforaminal lumbar interbody fusion (TLIF) or posterior lumbar interbody fusion (PLIF) which may increase biomechanical stability (5).
Maintenance of a stable mechanical environment is necessary during the fusion period, together with correct surgical technique and use of bone graft and/or biologics, to avoid non-union or distal failure. Movement can occur through the implants themselves (i.e., rod bending in pedicle screw constructs) or at the bone or implant interface (e.g., screw loosening, cage subsidence). In addition macroscopic failure due to implant pullout or periprosthetic sacral fracture may require revision surgery. Fixation strategies which are able to further reduce lumbosacral screw strain will likely be associated with decreases in pseudarthrosis rates (1,6-8).
To avoid such problems, an ideal technique to supplement a long lumbosacral construct should add little to the duration and technical difficulty of what is already demanding surgery, and if possible should avoid the need to re-position the patient for a different surgical approach. It should also require as little extra surgical dissection as possible, and be widely applicable to all patient groups requiring surgery with long constructs. Biomechanical analysis of S1 screw strain and L5–S1 range of motion (ROM) has shown that L2–S1 pedicle screw (PS) constructs with anterior axial interbody fusion (AxiaLIF) and PS construct with standard iliac screws provide similar L5–S1 stability, and both provide greater reduction in screw strain and L5–S1 ROM than PS construct with anterior lumbar interbody fusion (ALIF) and L2–S1 pedicle screws alone (5).
One method introduced to overcome complications associated with long fusions to the sacrum is sacropelvic fixation via the S2 alar iliac screw (S2AI). The S2AI technique is a new technique (9-12) that, when compared with standard iliac screws, offers reduced soft tissue dissection, lower profile instrumentation, easier alignment with cephalad fixation (S1 and above), and avoids the need for lateral connectors which reduces costs. As opposed to ALIF and AxiaLIF it can be performed through the same posterior approach as the main procedure, and when compared with TLIF/PLIF there is no need to expose and retract neural elements. Currently, the S2AI technique is used in a variety of clinical settings including long fusions involving the sacrum, flat back deformity, correction of pelvic obliquity, high grade spondylolisthesis, and lumbosacral fusion in the context of high grade osteoporosis.
In a previous biomechanical analysis by Fleisher et al. (5), the authors compared three strategies for reducing S1 pedicle screw (PS) strain in long constructs: PS alone, ALIF+PS, iliac screws +PS, and AxiaLIF + PS. The authors found that iliac screws and AxiaLIF had similar stability and reduce S1 screw strains in flexion and extension and axial torsion, when compared to PS alone or PS+ALIF. However, no studies have looked at the effect of S2AI screws on sacral screw or rod strain. In addition, there has been no previous comparison of S2AI screw fixation with TLIF support.
Thus, using a similar study design (5), we sought to examine and compare the biomechanical effects on an L2–S1 pedicle screw construct of adding S2AI screws, AxiaLIF, L5–S1 interbody support via transforaminal lumbar interbody fusion (TLIF), and to examine the effect of the addition of cross connectors to each of these constructs. The hypothesis of this study was that the addition of S2AI screws and cross connectors to the long construct would improve stiffness and reduce S1 screw strain, and that this effect would be similar to, or greater than, the effects of alternative forms of sacropelvic fixation.
Methods
Overview
The ROM and strain on the S1 pedicle screws and the right posterior fusion rod of seven L2-pelvis cadaveric spines were non-destructively assessed using a pure-moment flexibility testing protocol (±7.5 Nm). Specimens were tested intact and then in five different instrumentation states consisting of (I) PS from L2-S1; (II) PS + S2AI; (III) PS + TLIF at L5-S1; (IV) PS + AxiaLIF at L5-S1 and (V) PS + AxiaLIF + S2AI. In addition, each of the five instrumentation conditions was tested with cross connectors placed at L2–3 and below the S1 pedicle screws for the implanted tests. Tests were conducted in flexion-extension, lateral bending and axial torsion with no compressive preload. Motion was tracked using a non-contact camera-based motion measurement system with tracking of the kinetimatic signatures and simultaneous acquisition of strain data (Figure 1).
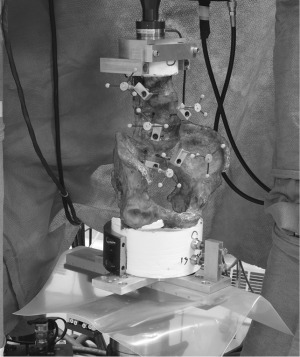
Cadaveric specimens
Seven L1-pelvis lumbar spine specimens ranging in age from 29 to 70 years were obtained (three male, four female) and scanned using DEXA (GE Lunar, iDXA, Piscataway, NJ, USA) to quantify bone density (Table 1). One specimen (#7) was osteoporotic with a T-score of –2.8, one specimen (#5) was osteopenic with a T-score of −2.1 and the remaining specimens had T-scores within the normal range with values of –0.9 to 1.0. Specimens were carefully dissected to preserve all ligamentous structures, and potted (Smooth-Cast 300, Smooth-On, Easton, PA, USA).
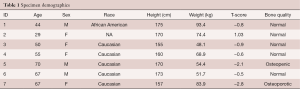
Full table
Surgical procedures and test conditions
All devices were implanted by practicing spine surgeons. Bilateral, strain gauge-instrumented polyaxial pedicle screws (7.5 mm × 55 mm, Mesa screws, K2M, Leesburg, VA, USA) were implanted at S1 and strain data were acquired for all test conditions and directions. Specimens were first instrumented with pedicle screws and rods from L2–S1 (6.5 mm × 35–50 mm at L2-L5, 7.5 mm × 55 mm at S1, Mesa screws, K2M) with S2AI screws (8.5 mm × 80 mm) and cross connectors at L2–3 and between the S1 and S2AI screws (the “S2AI” test condition, Table 2). The cross connectors were removed with the specimens in the load frame and tests were repeated for the S2AI condition. The S2AI screws were then released with the specimen on the load frame and tests were repeated for the pedicle screw condition. While still on the load frame the cross connectors were then re-attached and flexibility testing was again repeated. The specimens were then removed from the load frame and a TLIF was implanted at L5–S1; the cross connectors remained in place during implantation. Flexibility tests were conducted for the TLIF + cross connectors; the cross connectors were removed with the specimen on the machine and tests were repeated. The specimen was removed from the load frame and a trans-sacral approach was then utilized to implant a non-distracting AxiaLIF at L5–S1 (TranS1, Wilmington, NC, USA). The one-level AxiaLIF implants were implanted in the optimal position through the center of each vertebral body as confirmed using bi-planar fluoroscopy. Tests were sequentially repeated on the AxiaLIF, AxiaLIF + cross connectors, AxiaLIF + cross connectors + S2AI and AxiaLIF + S2AI.
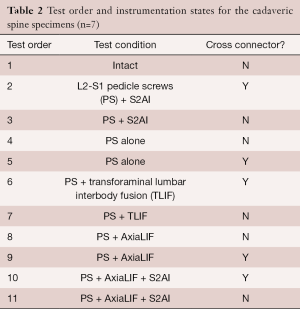
Full table
Flexibility testing
Flexibility tests were conducted to ±7.5 Nm with no preload in flexion-extension, lateral bending and axial torsion. Unconstrained moments were applied to the superior vertebral body using a hydraulically-actuated spinal loading fixture attached to a servo hydraulic load frame (MTS 858 Mini Bionix, MTS Systems, Eden Prairie, MN, USA) with the pelvis mounted to a passive XY slide table. A 6 DOF load cell mounted directly above the specimen was used to control the application of loads and moments. Moments of ±7.5 Nm were applied in the test direction of interest while moments in the remaining directions were maintained at 0 Nm. The loading waveform consisted of three cycles with moments being applied at a rate of 0.5 Nm/sec; data was only collected on the third cycle. Reflective marker triads were rigidly attached to the L2–S1 vertebral bodies and the pelvis. Spinal motion was tracked using a 4-camera non-contact motion measurement system (MX F20 cameras, Vicon, Oxford, UK).
Pedicle screw and rod strain
Pedicle screws were instrumented to directly measure biplanar screw bending by positioning strain gauges in two independent half bridge configurations. The strain gauge instrumented pedicle screws were implanted bilaterally at S1. The construction of these instrumented screws has been previously described (5). Strain gauges were positioned to measure bending moments occurring in the sagittal plane (primarily flexion-extension) and in the transverse plane.
Similarly to the screws, the right side rod was instrumented by positioning strain gauges in two independent half bridge configurations. The gauges were placed 7 cm from the end of the rod and were spaced 90 degrees apart around the rod circumference; flat surfaces were not milled on the rod. The gauges were oriented to measured bending moments occurring in the sagittal plane (primarily flexion-extension) and in the coronal plane (primarily lateral bending).
A dedicated computer equipped with a strain gauge signal conditioning board (Model 5100A Scanner, Vishay Micro-Measurements, Raleigh, NC, USA) was used for strain data acquisition.
Outcome measures
Although reflective markers were attached to five rigid bodies, ROM was only calculated at L5-S1, where the interbody devices were placed. ROM was defined as the difference between the peak positive and peak negative rotations.
The bending strain data were individually analyzed for each screw and the rod to determine the magnitude of the resultant moment. The resultant screw bending moment magnitude was calculated using the Pythagorean Theorem with the bending moments from the two strain channels as inputs. For simplicity, the resultant bending moment was only reported at the peak applied moment for each specimen (e.g., flexion and extension). Bending moment data from the left and right S1 screws were averaged (no statistical differences were present between left vs. right screws).
Statistics
A one-way repeated measures ANOVA was performed on the ROM and rod strain data with Bonferroni post-hoc tests. The screw strain data were non-normal and required transformation prior to statistical analysis. Bivariate analyses within each testing condition was conducted using Wilcoxon tests. Multivariate analysis was conducted using Least Square Means Tests with Bonferroni post-hoc tests. The screw strain data were retransformed for presentation. Statistical differences were declared at P<0.05.
Results
The osteopenic and osteoporotic specimens were excluded from analysis (#5 and #7).
Range of motion
The intact ROM averaged 10.2±3.1°, 6.6±2.0° and 4.4±2.1° in flexion-extension, lateral bending and axial torsion, respectively. The addition of any type of supplemental fixation (pedicle screws, S2AI, TLIF, AxiaLIF) significantly reduced ROM in all test directions by 69–97% compared to the intact condition.
In flexion-extension without cross connectors, ROM was greatest with pedicle screws alone at 1.5±1.0° and decreased with S2AI fixation (1.0±10.6°), TLIF (0.7±0.6°), AxiaLIF (0.7±1.0°) and AxaLIF + S2AI (0.4±0.5°, Figure 2). If no interbody device or spacer was used, then S2AI fixation had reduced ROM compared to pedicle. There appeared to be no differences between whether an AxiaLIF or TLIF was used as the interbody device. The use of cross-connectors also did not change the ROM.
In lateral bending, ROM values were the same for pedicle screws, TLIF and AxiaLIF at 0.5°. ROM in lateral bending was reduced with S2AI fixation and AxiaLIF + S2AI to 0.3° for both (Figure 3). As such, the short lever arm constructs (pedicle screws, AxiaLIF and TLIF) had higher overall ROM for lateral bending compared to long lever arm constructs (S2AI or S2AI+AxiaLIF).
In axial torsion, ROM was greatest with pedicle screws at 1.3±0.7° and lowest with S2AI fixation at 0.9±0.2° (Figure 4). Axial torsion ROM was similar for TLIF, AxiaLIF and AxiaLIF + S2AI at approximately 1.1°. Differences in ROM were only significant between pedicle screws alone and S2AI + AxiaLIF in flexion-extension, both with and without cross connectors. Thus, the trends showed that interbody devices (regardless of AxiaLIF or TLIF) were better than pedicle screws alone; S2AI constructs (S2AI + AxiALIF) were better compared to S1 (i.e., stopping at S1) constructs; and that cross-connectors appeared to have a beneficial effect in all constructs.
Flexion and extension strain
In flexion-extension the greatest screw strain occurred in the pedicle screws alone with values of 1.0±0.7 Nm in flexion and 0.6±0.5 Nm in extension (Figure 5). In flexion, strain was reduced to 0.5±0.3 Nm with TLIF; values were similar between AxiaLIF and S2AI at 0.3 Nm and were lowest with AxiaLIF + S2AI at 0.1±0.04 Nm. Similar trends were observed in extension. This suggests that all constructs performed better than pedicle screws alone in terms of screw bending moment; AxiaLIF (fixation interbody device) performs better than TLIF (non-fixed interbody device), AxiaLIF results appeared to be equivalent to S2AI, but S2AI + AxiaLIF produce the best result. The addition of cross-connectors was beneficial for all cases except S2AI + AxiaLIF.

The overall rod bending strains were greatest in flexion-extension for each instrumentation state (Figure 6). In flexion, rod bending strains were highest with S2AI fixation (1.2±0.7 Nm), reduced with pedicle screws alone (0.9±0.6 Nm) and were similar between TLIF, Axialif and AxiaLIF + S2AI (~0.55 Nm). In extension, pedicle screws and S2AI fixation had similar rod strain values of ~1.1 Nm, a reduction was observed with TLIF (0.8±0.6 Nm), AxiaLIF + S2AI (0.5±0.2 Nm) and values were lowest with AxiaLIF (0.4±0.1 Nm). Cross-connectors did not have an effect on flexion or extension rod strain.
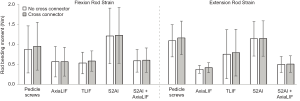
Torsion strain
Overall screw bending strains were greatest in axial torsion for each instrumentation state (Figure 7). Pedicle screws, TLIF and AxiaLIF had similar screw bending strains in torsion with values of 1.0 Nm. S2AI fixation and AxiaLIF + S2AI had the lowest screw strains in right torsion with values of 0.7 and 0.6 Nm, respectively.
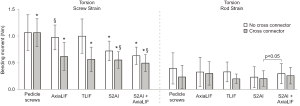
In axial torsion, rod strains were similar between pedicle screws (0.40 Nm), TLIF (0.33 Nm), AxiaLIF (0.34 Nm) and AxiaLIF + S2AI (0.32 Nm) with the smallest strains in the S2AI group (0.23 Nm).
Thus for torsion screw strain, S2AI constructs performed better compared to S1 constructs. The type of interbody device did not have an effect. The additional of cross-connectors improved the bending moment in all causes except for pedicle screws alone. In terms of torsion rod strain, all constructs were approximately equal, with cross-connectors having a modest effect.
Lateral bending strain
Lateral bending exhibited the lowest screw strains of the three test directions with values under 0.25 Nm for all instrumentation states (Figure 8). Pedicle screws, TLIF and AxiaLIF had similar screw strain values of ~0.20 Nm. The lowest screw strain values in lateral bending occurred with S2AI fixation and AxiaLIF + S2AI fixation with values of ~0.10 Nm. Thus, S2AI constructs produced lesser bending moments compared to S1 constructs, and the type of interbody device did not appear to have an effect. The additions of cross-connectors also did not affect lateral bending moment screw strain.
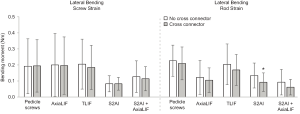
Lateral bending for rod strain exhibited the lowest rod strains of the three test directions with values under 0.26 Nm for all instrumentation states. There were not large variations in lateral bending rod strain with the largest values occurring with pedicle screws (0.2 Nm) and TLIF (0.15 Nm), and the smallest values with S2AI (0.11 Nm) and AxiaLIF + S2AI (0.08 Nm). Thus overall, S2AI constructs were better than S1 constructs in terms of lateral bending rod strain. AxiaLIF fixation was better than non-fixed TLIF interbody device and equivalent to S2AI constructs. Cross-connectors had a beneficial effect on lateral bending rod strain.
A summary of the statistical tests performed between the different subgroups is summarized in Tables S1-S6.
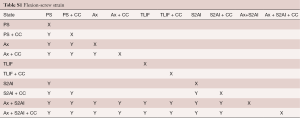
Full table
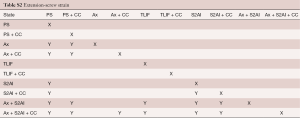
Full table
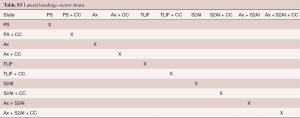
Full table
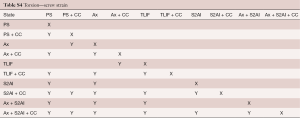
Full table
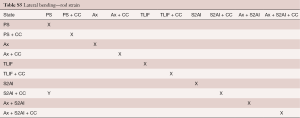
Full table
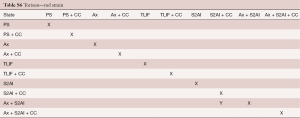
Full table
Discussion
Pelvic fixation remains a challenging concept in spinal deformity surgery, with ongoing developments to improve distal fixation and maintain stability of constructs in thoracolumbar surgery (13-15). Various techniques have been introduced and tested over time with the aim of maximizing fusion rates, minimizing construct failure, pseudarthrosis and loss of sagittal balance (15). In the context of long lumbosacral constructs, support instrumentation have included Galveston techniques, S1 alar screws, interbody fusion and iliac fixation (8,16-20). However, these solutions have their limitations. Whilst iliac fixation has been associated with reasonable fusion rates (1,7), there have been concerns over instrumentation prominence and postoperative pain reported with traditional iliac fixation (21). Further, iliac fixation also requires exposure of the posterior superior iliac spine (PSIS) via dissection which could further exacerbate wound healing and infections (7).
The S2AI technique was introduced as an alternative to traditional iliac fixation, with purported advantages of reduced soft tissue dissection, lower profile instrumentation, and the avoidance of lateral connectors (9,10,22). S2AI fixation offers the advantages of being able to use the same posterior approach as the main procedure and without requiring the exposure and retraction of neural elements, which makes this technique theoretically more attractive compared to ALIF, AxiaLIF and TLIF/PLIF. Early biomechanical studies have compared S2AI and standard iliac screws and have demonstrated similar reductions in ROM when compared with pedicle screws alone (11). However, the question of their relative effects on sacral screw strain and rod strain in terms of flexion, extension, lateral bending, and axial torsion have not been reported in the literature to the best of our knowledge. Furthermore, the biomechanics of S2AI fixation compared with interbody support has not been well elucidated, providing further impetus for the present report.
In the current study, any type of supplemental fixation (pedicle screws, S2AI, TLIF, AxiaLIF) significantly reduced ROM in all directions. S2AI reduced flexion-extension ROM to a greater degree compared to pedicle screws alone, but with similar ROM compared to supplemental TLIF and AxiaLIF. Lateral bending ROM for S2AI was reduced compared to pedicle screws, TLIF and AxiaLIF. The greatest reduction in ROM in all directions was found using a combination of S2AI and AxiaLIF fixation. Axial torsion ROM was lowest with S2AI, but similar between TLIF, AxiaLIF and AxiaLIF+S2AI. ROM values and trends were found to be comparable to a previous biomechanical study, which also demonstrated similar reductions in flexion and extension for iliac screws and AxiaLITR, compared to PS alone. Whilst our study noted also similar reductions in flexion and extension ROM for TLIF anterior column loading, this was found to have a greater effect compared to ALIF used by the Fleischer et al. study (5).
In terms of S1 screw flexion and extension strain, this was similar between AxiaLIF and S2AI, both which had lower strain compared to TLIF and PS alone. The AxiaLIF and S2AI combination appeared to have an additive effect, with the lowest S2 flexion and extension screw strain. For lateral bending and axial torsion, the S1 screw strain was lowest for S2AI and AxiaLIF + S2AI fixation. We also found that axial torsion strains were lowest using the S2AI or S2AI + AxiaLIF strategies, whilst AxiaLIF or TLIF produced similar screw bending moment when compared to PS alone. These trends were similar to that reported in Fleischer et al., who demonstrated the greatest reductions in screw bending moments with either AxiaLIF or “Galveston-type” iliac fixation strategy, compared to ALIF or PS alone for flexion and extension. Whilst Fleischer et al. noted similar right and left torsion between iliac screw and AxiaLIF strategies, we had found that AxiaLIF produced similar screw torsion strain compared to PS alone.
In terms of rod strain, flexion and extension strain was significantly higher for S2AI compared to AxiaLIF, TLIF or S2AI + AxiaLIF supplementation. This was unexpected, one explanation may be that the rod strain above the S1 screws is greater in S2AI fixation compared to pedicle screw constructs ending at S1 because there is likely more movement at the S1 bone and screw interface in the pedicle screw construct compared to S2AI fixation. Another more likely explanation may be that the S2AI construct exposes the rods to a longer lever arm than the S1 pedicle screw alone constructs, resulting in higher moments and strains within the rods. In contrast, lateral bending and axial torsion rod strain was similar amongst S2AI with other fixation approaches. It appears that at the expense of high rod strain in flexion/extension, S2AI produces reduced S1 screw strain and rod strain in lateral/axial directions in comparison to traditional iliac fixation and interbody instrumentation. Furthermore, the use of cross-connectors in each instrumentation state produced additional small reductions in screw strain in flexion-extension, but had a limited effect in lateral bending.
There was a significant strain reduction noted in axial torsion. In comparison to Fleischer et al.’s study which used screws with a traditional “tulip head and set screw” mechanism, the present study used a K2M Mesa “taper lock” screw mechanism. No significant differences were found in ROM and S1 screw strain for PS only subgroup outcomes between the present paper and previous investigation. As such, there is no definitive evidence for differences in biomechanical stability for the “taper lock” mechanism versus “tulip head and set screw” mechanism, but this hypothesis needs further in-depth investigation.
Overall, these results demonstrate: (I) Similar flexion, extension, and lateral ROM and S1 screw strain for PS, PS + AxiaLIF and PS + interbody compared to a prior biomechanical study on long construct pelvic fixation strategies; (II) the use of cross-links produce additional small benefits in terms of screw strain reduction in flexion-extension and axial torsion; (III) similar screw strain results achieved with taper-lock versus set-screw mechanisms; (IV) similar ROM and bending moments achieved with either ALIF versus TLIF for anterior column support using spacers; and (V) S2AI fixation strategy appears to be biomechanically equivalent to the traditional “Galveston-type” iliac fixation in terms of ROM, screw strain and rod strain.
Our results confirm prior reports of similar torsional stiffness in extension, flexion, left bending or right bending between S2AI and iliac screw constructions. Burns et al. (23) also reported similar failure torque between S2AI versus iliac screws as well as yield torque, and the authors concluded that the S2AI approach may be an alternative associated with less morbidity but similar stiffness and load-to-failure biomechanics. O’Brien et al. (11) compared the biomechanical strength of the S2AI screw compared with iliac fixation as well as the effect of length and trajectory. The authors reported similar biomechanical stability between the two approaches in all loading modes, and similar biomechanical characteristics between 90 mm iliac screws with 80 mm S2AI screws. There was statistically no significant difference between the S2AI screws of all lengths and the iliac screw constructs with offset connectors.
Our study is the first to compare the biomechanical properties of the S2AI compared with interbody instrumentation using a similar fixation construct. We demonstrated that the addition of S2AI screws and cross-connections to the long construct improved stiffness and reduced S1 screw strain, with a greater effect compared to TLIF supplementation. Furthermore, we also demonstrated that the concurrent S2AI and AxiaLIF supplementation produced the greatest reductions in S1 screw strain and rod strain across all loads applied.
There are several limitations in this study. Firstly, a small sample size of cadaveric specimens were used, with two specimens excluded due to being osteopenic or osteoporotic. There was significant variation in the T-score of each specimen, partly attributed to the limited number of cadaveric specimens included which caused diversity in bone density distribution. Secondly, other parameters such as fatigue, pull-out profile, and clinical outcomes were not measured in the present study, which may be important when deciding the method of distal fixation in long lumbosacral constructs. Future clinical studies with larger sample sizes will be required to confirm the presented biomechanical concepts.
Conclusions
In summary, S2A1 produces reduced S1 screw strain for flexion-extension, lateral bending and axial torsion, as well as reduced rod strain in lateral bending and axial torsion in comparison to AxiaLIF and interbody instrumentation, at the expense of increased rod flexion-extension strain. Cross-connectors may have a role in further reduction of S1 screw and rod strain. From a biomechanical standpoint, the use of the S2AI technique is at least equivalent to traditional iliac screws, but offers lower prominence and ease of assembly compared to conventional sacroiliac stabilization.
Acknowledgements
The authors wish to thank Jane Luscombe RN of ProCRO Pty Ltd, Sydney, NSW Australia and Spinal Health International, Longboat Key, Florida USA for her review of this manuscript.
Funding: The authors received financial support in the form of an unrestricted research grant from LifeHealthcare Pty Ltd North Ryde NSW Australia and K2M Inc., Leesburg, Virginia USA.
Footnote
Conflicts of Interest: Chester E. Sutterlin III is a consultant for LifeHealthcare and receives royalties from K2M (for an implant unrelated to this research). The other authors have no conflicts of interest to declare.
Ethical Statement: No formal ethics approval was required as the present study is a biomechanical study with no patients involved.
References
- Kuklo TR, Bridwell KH, Lewis SJ, et al. Minimum 2-year analysis of sacropelvic fixation and L5-S1 fusion using S1 and iliac screws. Spine (Phila Pa 1976) 2001;26:1976-83. [Crossref] [PubMed]
- Weistroffer JK, Perra JH, Lonstein JE, et al. Complications in long fusions to the sacrum for adult scoliosis: minimum five-year analysis of fifty patients. Spine (Phila Pa 1976) 2008;33:1478-83. [Crossref] [PubMed]
- Lee CS, Chung SS, Choi SW, et al. Critical length of fusion requiring additional fixation to prevent nonunion of the lumbosacral junction. Spine (Phila Pa 1976) 2010;35:E206-11. [Crossref] [PubMed]
- Camp JF, Caudle R, Ashmun RD, et al. Immediate complications of Cotrel-Dubousset instrumentation to the sacro-pelvis. A clinical and biomechanical study. Spine (Phila Pa 1976) 1990;15:932-41. [Crossref] [PubMed]
- Fleischer GD, Kim YJ, Ferrara LA, et al. Biomechanical analysis of sacral screw strain and range of motion in long posterior spinal fixation constructs: effects of lumbosacral fixation strategies in reducing sacral screw strains. Spine (Phila Pa 1976) 2012;37:E163-9. [Crossref] [PubMed]
- Bridwell KH. Utilization of iliac screws and structural interbody grafting for revision spondylolisthesis surgery. Spine (Phila Pa 1976) 2005;30:S88-96. [Crossref] [PubMed]
- Tsuchiya K, Bridwell KH, Kuklo TR, et al. Minimum 5-year analysis of L5-S1 fusion using sacropelvic fixation (bilateral S1 and iliac screws) for spinal deformity. Spine (Phila Pa 1976) 2006;31:303-8. [Crossref] [PubMed]
- Tumialán LM, Mummaneni PV. Long-segment spinal fixation using pelvic screws. Neurosurgery 2008;63:183-90. [Crossref] [PubMed]
- Chang TL, Sponseller PD, Kebaish KM, et al. Low profile pelvic fixation: anatomic parameters for sacral alar-iliac fixation versus traditional iliac fixation. Spine (Phila Pa 1976) 2009;34:436-40. [Crossref] [PubMed]
- O’Brien JR, Yu WD, Bhatnagar R, et al. An anatomic study of the S2 iliac technique for lumbopelvic screw placement. Spine (Phila Pa 1976) 2009;34:E439-42. [Crossref] [PubMed]
- O’Brien JR, Yu W, Kaufman BE, et al. Biomechanical evaluation of S2 alar-iliac screws: effect of length and quad-cortical purchase as compared with iliac fixation. Spine (Phila Pa 1976) 2013;38:E1250-5. [Crossref] [PubMed]
- Mattei TA, Fassett DR. Low-profile pelvic fixation with sacral alar-iliac screws. Acta Neurochir (Wien) 2013;155:293-7. [Crossref] [PubMed]
- Crawford CH 3rd, Carreon LY, Bridwell KH, et al. Long fusions to the sacrum in elderly patients with spinal deformity. Eur Spine J 2012;21:2165-9. [Crossref] [PubMed]
- Kostuik JP. Spinopelvic fixation. Neurol India 2005;53:483-8. [Crossref] [PubMed]
- Moshirfar A, Rand FF, Sponseller PD, et al. Pelvic fixation in spine surgery. Historical overview, indications, biomechanical relevance, and current techniques. J Bone Joint Surg Am 2005;87 Suppl 2:89-106. [PubMed]
- Allen BL Jr, Ferguson RL. The Galveston technique of pelvic fixation with L-rod instrumentation of the spine. Spine (Phila Pa 1976) 1984;9:388-94. [Crossref] [PubMed]
- Kasten MD, Rao LA, Priest B. Long-term results of iliac wing fixation below extensive fusions in ambulatory adult patients with spinal disorders. J Spinal Disord Tech 2010;23:e37-42. [Crossref] [PubMed]
- Neustadt JB, Shufflebarger HL, Cammisa FP. Spinal fusions to the pelvis augmented by Cotrel-Dubousset instrumentation for neuromuscular scoliosis. J Pediatr Orthop 1992;12:465-9. [Crossref] [PubMed]
- O’Brien MF, Kuklo TR, Lenke LG. Sacropelvic instrumentation: Anatomic and biomechanical zones of fixation. Seminars in Spine Surgery 2004;16:76-90. [Crossref]
- Taddonio RF. Segmental spinal instrumentation in the management of neuromuscular spinal deformity. Spine (Phila Pa 1976) 1982;7:305-11. [Crossref] [PubMed]
- Peelle MW, Lenke LG, Bridwell KH, et al. Comparison of pelvic fixation techniques in neuromuscular spinal deformity correction: Galveston rod versus iliac and lumbosacral screws. Spine (Phila Pa 1976) 2006;31:2392-8; discussion 2399. [Crossref] [PubMed]
- Sponseller PD, Zimmerman RM, Ko PS, et al. Low profile pelvic fixation with the sacral alar iliac technique in the pediatric population improves results at two-year minimum follow-up. Spine (Phila Pa 1976) 2010;35:1887-92. [Crossref] [PubMed]
- Burns CB, Dua K, Trasolini NA, et al. Biomechanical Comparison of Spinopelvic Fixation Constructs: Iliac Screw Versus S2-Alar-Iliac Screw. Spine Deformity 2016;4:10-5. [Crossref] [PubMed]
Contributions: (I) Conception and design: All authors; (II) Administrative support: CE Sutterlin, A Field, LA Ferrara, AL Freeman; (III) Provision of study materials or patients: CE Sutterlin, A Field, LA Ferrara, AL Freeman; (IV) Collection and assembly of data: CE Sutterlin, A Field, LA Ferrara, AL Freeman; (V) Data analysis and interpretation: All authors; (VI) Manuscript writing: All authors; (VII) Final approval of manuscript: All authors.