Systematic review of 3D printing in spinal surgery: the current state of play
Introduction
Three-dimensional printing (3DP) is a rapidly growing industry. A variety of techniques can be used to print physical models from three-dimensional renderings based on CAD software, and STL design files. Whilst the use of this technology in medicine is still in its infancy, 3DP offers the possibility of revolutionising healthcare with its ability to rapidly create customized shapes from a wide range of materials (1-6).
One sector of medicine where 3DP offers great potential benefits is in neurosurgery, and in particular spinal surgery. Due to the complex anatomy of the spine, as well as the delicate nature of the surrounding structures, any technique that may aid surgical planning and procedural accuracy offers the ability to improve patient outcomes (5). This systematic review will cover the current applications of 3DP in spinal surgery, including its role in surgical planning, surgical guides, customised implants and “Off-the-Shelf” implants. It will conclude with a brief overview of future directions of 3DP in this field.
Methods
Purpose
The objective of this review is to summarize the literature regarding the use of 3D printing technologies for the planning or production of patient-specific implants (PSIs) for spinal surgery. A comprehensive search of the Medline and EMBASE databases was conducted in line with the PRISMA guidelines (7-9) (Supplementary).
Search strategy
A comprehensive search of published reports was performed via six electronic databases; namely, Ovid Medline, PubMed, Cochrane Central Register of Controlled Trials, Cochrane Database of Systematic Reviews, American College of Physicians Journal Club and Database of Abstracts of Review of Effectiveness from their date of inception to March 2017. To maximise the sensitivity of the search strategy, the terms; “three-dimensional”, “3D”, “patient-specific”, “spinal surgery”, “fusion”, were combined as either key words or MeSH terms. The reference lists of all retrieved articles were reviewed to identify additional potentially relevant studies.
The population to be studied included any patient presenting for spinal surgery. Exclusion criteria included a non-surgical focus, non-spinal focus, animal studies and 3D imaging focus. No limits were set on time or level of evidence, as 3DP in spinal surgery is recent and evidence is mainly limited to low-power studies.
Results
These searches returned a combined 2,411 articles, of which 453 duplicates were removed, before the remaining 1,958 articles were screened by title and abstract for relevancy, leaving 75 articles for full text review. Of these, 54 were included in this review. A flow-chart of this process is shown in Figure 1 (9).
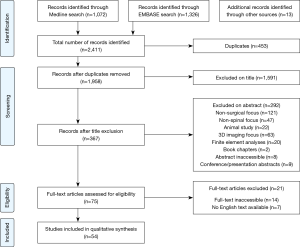
A summary of 3DP of pre-operative models is given in Table 1. A summary of 3DP surgical guides is given in Table 2. Table 3 summarizes the current literature on the use of customised 3DP implants for spine surgical procedures.
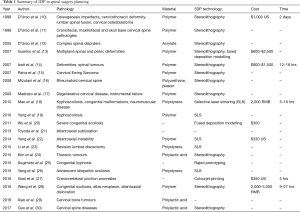
Full table
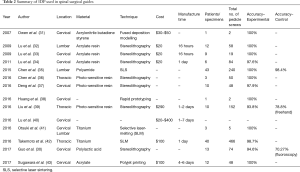
Full table
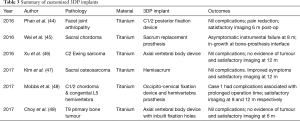
Full table
Discussion
Surgical planning
3DP is most frequently utilised in spinal surgery in the pre-operative planning stage. A full-scale, stereoscopic understanding of the pathology allows for more detailed planning and simulation of the procedure (10-18,20-30,50-52). Assessing complex pathologies on a model overcomes many of the issues associated with traditional 3D imaging, such as the lack of realistic anatomical representation and the associated complexity of computer-related skills and techniques (10,12). Sugimoto et al. (25) reported that the usefulness of 3DP increases with the complexity of the pathology, with the surgeon’s ability to manoeuvre a model being useful to appreciate patient’s anatomy without having to mentally reconstruct multiple 2D images (Table 1).
The improved visualization and preparation afforded by the use of individualized models has clinical benefits, with reduced operation time and perioperative blood loss being most commonly reported (11,13,14,21-23,26-28,30). Reduction in operation time of 15–20% has been reported in multiple studies (11,14,23) across various surgical procedures. The main reasons given for reduced operation time included a more evolved understanding of the pathology, such as location and surgical approach, and the facilitation of pre-operative instrumentation decisions (14,16,18-21,24,27,28,50). Other clinical benefits such as improved diagnosis, reduction in fluoroscopy time, better communication within the surgical team and lower rates of screw misplacement have been reported when compared with the use of conventional imaging in pre-operative planning (11,14,20,26,30). Izatt et al. (14) found that the use of a 3DP biomodel improved surgical outcomes in 78% of cases, though this is contradicted by Li et al. (23) who reported no change in complication rates or clinical outcomes. As many of the studies performed are small scale with no controls, it is likely there is not enough evidence to properly elicit benefits of 3DP models in surgical preparation on these parameters. Larger scale studies with the power to detect differences more accurately are required.
Some barriers exist for the translation of 3DP models for surgical planning in small scale studies to general clinical practice. In the studies reviewed, time taken to create the models ranged from 5 hours to 2 days, with extra costs anywhere between $300 and >$1,000 (10,11,13,18,20,22,26,27). Izatt et al. (14) stated that these additional costs were offset in 59% of cases by the more efficient operative technique and planning afforded by the models, as well as fewer intra-operative complications and greater accuracy associated with their use. This was supported by Wang et al. (28), who found that the decreased need for intra-operative navigation made the procedure more economical. However, time and cost are still likely to limit the use of this technology in the immediate future. Other limitations include a lack of surgically useful information, such as joint instability, and an absence of real-time information like those provided by imaging (18). Guarino et al. (13) also found that access issues were not only due to cost, but also a paucity of providers with the equipment and expertise to make the widespread use of this technology commercially viable. The learning curve for the required familiarity with the software has also been reported as a barrier (23,30). As time taken to create the models and the associated costs continue to decline, there should be an increase in the use of this technology in clinical practice.
Another well reported benefit of 3DP models is improved patient education (11,13,17,19,53). A physical model is much easier for a patient to understand than complex MRI and CT scans. D’Urso et al. (11) reported a statistically significant improvement in informed consent from the patient’s perspective, confirmed by 25% higher patient informed consent scores using a biomodel demonstration when compared to pre-operative image demonstration. In one study (17), anxiety-related pain was found only to be relieved after a patient understood her condition with the aid of a biomodel, suggesting using models in patient education may improve clinical outcomes.
Surgical guides
Spinal surgery is inherently dangerous due to the delicate nature of the surrounding anatomy. Intra-operative guides, created with patient-specific data, may have the ability to mitigate the risks associated with these procedures (4,54).
Pedicle screws are a fixation technique routinely used in spinal surgery, as they are the most effective way to stabilize vertebrae (4,37,43) and lower the risk of complications if inserted accurately (31). However, traditional techniques are associated with many problems and carry a high risk of breaching the pedicle, with the possibility of causing potentially fatal neurovascular injury (32). Thus, manual insertion based on surface anatomical landmarks, which is heavily dependent on the experience of the surgeon, is potentially undesirable. Computer assisted surgery can be used to monitor the placement of screws and increase the safety of these procedures. However this system is very expensive, complex to operate, cumbersome, has a long learning curve, often requires additional personnel and is associated with prolonged intra-operative time due to bone registration requirements (32,35,39). There are also issues with intra-operative changes in position, decreasing the accuracy of this technique (32). The use of intra-operative imaging increases harmful radiation exposure for both doctors and patients (30,34-36). 3DP guides may offer an alternative as a simple, convenient, low cost and complex-equipment free way to improve the accuracy of pedicle screw placement (30-37,39-43).
To create 3DP screw guides, a 3D CT scan must be taken of the target vertebrae. Next, a 3D model is generated by specialist software from which the optimal screw trajectory and size can be determined, often in consultation with the surgeon. Finally, a navigational template is designed and built with optimal screw trajectory, the surface of which is the inverse of the posterior vertebral surface to ensure a good fit and accuracy of screw insertion (30-43,55). Numerous studies demonstrated that the guides help to lower operation time, with Deng et al. (37) suggesting this may decrease complications related to operative time (e.g., infection) (30,35,37,43). Other benefits include decreased intra-operative radiation, simplicity of use, elimination of procedural subjectivity, enhanced pre-operative planning and moderate cost in comparison with other techniques (30,32-38,42,43).
It has been suggested that these guides may be particularly useful with more complex anatomy. In the cervical spine, asymmetry, vast inter-patient differences such as anomalous vertebral artery course, and the sensitive nature of surrounding tissues make procedural success more difficult to achieve, and hence able to benefit from individualised guides (30,31,33,34,37,38,40,43,56). Otsuki et al. (41) demonstrated that 3DP guides provide an extra benefit in revision surgery, where screw insertion is more difficult due to morphology changes caused by the first operation.
The most commonly reported issue with the use of these templates is the necessity for clean bone preparation. Soft tissue must be completely removed for the template to fix into the correct spot, which may increase operation time and intra-operative blood loss (34,37,40,43). Depending on the material of the template, debris may be produced intra-operatively. Takemoto et al. (42) utilised titanium templates with reduced contact area to minimise these problems, though the resulting templates were 5× the price of polymer based guides. Whilst cost is generally favourable in comparison to other techniques, the time taken to design and manufacture the guides was viewed as a negative. This limits the usefulness of this technology, for example it is not feasible in an emergency (30,37,40,42). Other concerns include deformation during the production, sterilisation or surgical procedure (37) as well as the long learning curve for software operation (30,40).
Whilst the use of 3DP surgical guides in spinal surgery is mainly limited to fixation screw placement, several other procedures have been found to benefit from the technology. Rong et al. (57) utilised 3DP to create guides for expansive open-door laminoplasty in cervical myelopathy. Trough preparation requires experience, and the authors reported positive results with high correlation of trough position to the ideal placement planned. Anchoring the drill template served to minimise any intra-operative movement, whilst depth control measures on the templates helped prevent iatrogenic injury to the spinal cord or nerve roots. Lin et al. (58) also prevented iatrogenic complications with a customized osteotomy tool guiding resection of a giant sacral schwannoma. As these tumours are clinically difficult to remove due to the extent of local invasion and the complexity of surrounding anatomy, complete removal without neurovascular complications or spinal instability is ideal, although difficult. In this case, more accurate intra-operative localization of the resection margin allowed total resection, with no complications at 2-year follow-up.
Customised implants
One of the most exciting applications of 3DP in spinal surgery is the ability to manufacture customised, patient-specific implants. Patients being subjected to complex surgery with difficult anatomy and deformity, have an increased risk of implant failure, especially if the “off-the-shelf” reconstructive option does not fit accurately into the reconstructive defect. Despite being a novel field of study, it is hoped that PSIs will prove to have better durability due to a more even load distribution and superior osseointegration. Currently, literature on PSIs in spinal surgery is limited to a select few case reports and case series, though this is predicted to expand rapidly over the next few years.
The cases performed thus far are limited to anatomically challenging, rare pathologies where an individualized solution to restore patient-specific anatomy is a key prognostic factor (44,46-48,59,60). These range from customised fixation in the upper cervical and lower lumbar spine (44,48,59) to insertion of a 3DP vertebra (46) and sacral reconstructions (45,47). A summary of PSIs used in spinal surgery in the literature is shown in Table 3. The lack of specialised implants for reconstruction after tumour resection is evidenced by the majority of cases being oncological in nature (45-48). The remaining cases utilised 3DP PSIs due to unique anatomy associated with degenerative change and a congenital anomaly (44,48). All customised prostheses were made from titanium alloy (TiV6Al4) due to its biocompatibility and ability to enhance bone healing by porosity optimisation to match trabecular bone structure.
All the implants were reported to fit well, indicating the accuracy of the 3DP process. This increases the stability of the implant whilst minimising complications such as stress shielding and subsidence (45-47). Xu et al. (46) created a prosthesis with zero profile anteriorly for this purpose, minimising the risk of dysphagia in their patient. This case also noted the minimisation of other morbidities, such as pain and limited function, associated with bone grafts. Another benefit of customised implants is a reduction in operation time (44,46-48), with Mobbs et al. (48) noting the ability to avoid bone harvesting with intra-operative fashioning to fit complex defects. Wei et al. (45) produced three different sized prostheses to fit the intra-operative bone defect. Kim et al. (47) described a process of multiple pre-operative modifications made to the implant to ensure anatomical accuracy. Both these measures shifted the time from intra-operative to the pre-operative planning phase, a positive for minimising complications such as infections. The sacrum produced by Wei et al. (45) had pre-designed holes and screw heads to simplify the reconstructive method. The PSI employed by Phan et al. (44) was found to reduce the risk of neurovascular complications due to screw holes with pre-calculated angulation and depth in-built.
Negatives are similar to those for other 3DP applications in spinal surgery, with extra time and cost required to design these highly specialised PSIs (44). The sophisticated software and machinery necessary for creation of the implants is also a barrier (48). Wei et al. (45) reported an instrumental failure, indicating more direct comparison with standard of care implants is required to demonstrate the efficacy of these prostheses. Comparative studies would also serve to remove the inherent subjectivity of case reports on a new technology, where results are often positively skewed (5). There is also a lack of long-term data on the performance of these prostheses (44). It should be noted that no regulatory framework exists for the use of PSIs in spinal surgery. A system for registration and approval for the production and implantation of these devices will be necessary in the future. Despite these factors, due to the positive patient outcomes and lack of serious complications shown thus far, it would appear that these techniques offer a viable future direction for spinal prostheses, particularly in complex cases.
3D printing of “Off-the Shelf” implants
Whilst customisation to patient-specific data is one of the biggest drawcards of 3DP, the technique itself offers advantages compared to traditional manufacturing processes. Spinal prosthetic manufacturers, such as Stryker and 4Web Medical, are beginning to utilise the technology to optimise the properties of devices to be implanted (61-64). 3DP allows the manufacturing of previously un-manufacturable geometries (61), including the ability to mimic the interconnected structure of cancellous bone. Through control of the porosity and surface roughness of implants, osseointegration can be optimized. When combined with an open architecture to allow maximum bone graft volume, implants can be created that make full use of the capabilities of 3DP, without all the planning associated with customisation. By offering a range of sizes of implants, including parameters such as widths, heights, lengths and angles, spinal instrumentation can be utilised in patients whilst minimising complications such as implant migration and stress-shielding (62-64). The use of 3DP in this setting is cost-effective and can produce the quantity of implants necessary to be competitive in the market place. In the future, it is predicted that more innovative features may be able to be incorporated, such as porous matrices where density, pore diameter and mechanical properties can differ in different regions of the implant (61).
Future directions
As 3DP technology continues to become cheaper, faster and more accurate, its use in the setting of spinal surgery is likely to become routine, and in a greater number of procedures (1-4,6,42,44,65). Lower cost desktop 3D printers for everyday use could soon be a reality, aiding real-time model and implant creation for more personalised surgical care (46,48). A greater range of materials is also expected to open new avenues, with improved biocompatibility, osseointegration, biodegradability and load-bearing properties just some of the expected benefits (3,4,48,65). However, the greatest step forward is anticipated to be bioprinting, where cells, growth factors and biomaterial are used to create living tissue. This could conceivably be used for direct tissue repair, and even the printing of complex organs in the foreseeable future (6).
Conclusions
3DP is rapidly becoming intimately integrated in both spinal surgical practice and the literature. It is currently used for surgical planning, intra-operative surgical guides, customised prostheses as well as “Off-the-Shelf” implants. The technology allows for enhanced implant properties, as well as decreased surgical time and improved patient outcomes. However, much of the data thus far is from low-quality studies with inherent biases linked with the excitement of a new field. As the body of literature continues to grow, larger scale studies and longer-term follow ups will enhance our knowledge of the effect 3DP has in spinal surgery.
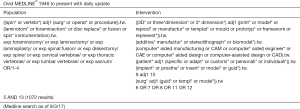
Full table
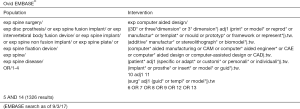
Full table
Acknowledgements
None.
Footnote
Conflicts of Interest: The authors have no conflicts of interest to declare.
References
- Eltorai AE, Nguyen E, Daniels AH. Three-Dimensional Printing in Orthopedic Surgery. Orthopedics 2015;38:684-7. [Crossref] [PubMed]
- Gibbs DM, Vaezi M, Yang S, et al. Hope versus hype: what can additive manufacturing realistically offer trauma and orthopedic surgery? Regen Med 2014;9:535-49. [Crossref] [PubMed]
- Martelli N, Serrano C, van den Brink H, et al. Advantages and disadvantages of 3-dimensional printing in surgery: A systematic review. Surgery 2016;159:1485-500. [Crossref] [PubMed]
- Provaggi E, Leong JJ, Kalaskar DM. Applications of 3D printing in the management of severe spinal conditions. Proc Inst Mech Eng H 2017;231:471-86. [Crossref] [PubMed]
- Tack P, Victor J, Gemmel P, et al. 3D-printing techniques in a medical setting: a systematic literature review. Biomed Eng Online 2016;15:115. [Crossref] [PubMed]
- Ventola CL. Medical Applications for 3D Printing: Current and Projected Uses. P T 2014;39:704-11. [PubMed]
- Phan K, Mobbs RJ. Systematic reviews and meta-analyses in spine surgery, neurosurgery and orthopedics: guidelines for the surgeon scientist. J Spine Surg 2015;1:19-27. [PubMed]
- Phan K, Tian DH, Cao C, et al. Systematic review and meta-analysis: techniques and a guide for the academic surgeon. Ann Cardiothorac Surg 2015;4:112-22. [PubMed]
- Moher D, Liberati A, Tetzlaff J, et al. Preferred reporting items for systematic reviews and meta-analyses: the PRISMA statement. PLoS Med 2009;6:e1000097. [Crossref] [PubMed]
- D'Urso PS, Askin G, Earwaker JS, et al. Spinal biomodeling. Spine (Phila Pa 1976) 1999;24:1247-51. [Crossref] [PubMed]
- D'Urso PS, Barker TM, Earwaker WJ, et al. Stereolithographic biomodelling in cranio-maxillofacial surgery: a prospective trial. J Craniomaxillofac Surg 1999;27:30-7. [Crossref] [PubMed]
- D'Urso PS, Williamson OD, Thompson RG. Biomodeling as an aid to spinal instrumentation. Spine (Phila Pa 1976) 2005;30:2841-5. [Crossref] [PubMed]
- Guarino J, Tennyson S, McCain G, et al. Rapid prototyping technology for surgeries of the pediatric spine and pelvis: benefits analysis. J Pediatr Orthop 2007;27:955-60. [Crossref] [PubMed]
- Izatt MT, Thorpe PL, Thompson RG, et al. The use of physical biomodelling in complex spinal surgery. Eur Spine J 2007;16:1507-18. [Crossref] [PubMed]
- Paiva WS, Amorim R, Bezerra DA, et al. Aplication of the stereolithography technique in complex spine surgery. Arq Neuropsiquiatr 2007;65:443-5. [Crossref] [PubMed]
- Mizutani J, Matsubara T, Fukuoka M, et al. Application of full-scale three-dimensional models in patients with rheumatoid cervical spine. Eur Spine J 2008;17:644-9. [Crossref] [PubMed]
- Madrazo I, Zamorano C, Magallón E, et al. Stereolithography in spine pathology: a 2-case report. Surg Neurol 2009;72:272-5; discussion 275. [Crossref] [PubMed]
- Mao K, Wang Y, Xiao S, et al. Clinical application of computer-designed polystyrene models in complex severe spinal deformities: a pilot study. Eur Spine J 2010;19:797-802. [Crossref] [PubMed]
- Yang JC, Ma XY, Lin J, et al. Personalised modified osteotomy using computer-aided design-rapid prototyping to correct thoracic deformities. Int Orthop 2011;35:1827-32. [Crossref] [PubMed]
- Wu ZX, Huang LY, Sang HX, et al. Accuracy and safety assessment of pedicle screw placement using the rapid prototyping technique in severe congenital scoliosis. J Spinal Disord Tech 2011;24:444-50. [Crossref] [PubMed]
- Toyoda K, Urasaki E, Yamakawa Y. Novel approach for the efficient use of a full-scale, 3-dimensional model for cervical posterior fixation: a technical case report. Spine (Phila Pa 1976) 2013;38:E1357-60. [Crossref] [PubMed]
- Yang JC, Ma XY, Xia H, et al. Clinical application of computer-aided design-rapid prototyping in C1-C2 operation techniques for complex atlantoaxial instability. J Spinal Disord Tech 2014;27:E143-50. [PubMed]
- Li C, Yang M, Xie Y, et al. Application of the polystyrene model made by 3-D printing rapid prototyping technology for operation planning in revision lumbar discectomy. J Orthop Sci 2015;20:475-80. [Crossref] [PubMed]
- Kim MP, Ta AH, Ellsworth WA 4th, et al. Three dimensional model for surgical planning in resection of thoracic tumors. Int J Surg Case Rep 2015;16:127-9. [Crossref] [PubMed]
- Sugimoto Y, Tanaka M, Nakahara R, et al. Surgical treatment for congenital kyphosis correction using both spinal navigation and a 3-dimensional model. Acta Med Okayama 2012;66:499-502. [PubMed]
- Yang M, Li C, Li Y, et al. Application of 3D rapid prototyping technology in posterior corrective surgery for Lenke 1 adolescent idiopathic scoliosis patients. Medicine (Baltimore) 2015;94:e582. [Crossref] [PubMed]
- Goel A, Jankharia B, Shah A, et al. Three-dimensional models: an emerging investigational revolution for craniovertebral junction surgery. J Neurosurg Spine 2016;25:740-4. [Crossref] [PubMed]
- Wang YT, Yang XJ, Yan B, et al. Clinical application of three-dimensional printing in the personalized treatment of complex spinal disorders. Chin J Traumatol 2016;19:31-4. [Crossref] [PubMed]
- Xiao JR, Huang WD, Yang XH, et al. En Bloc Resection of Primary Malignant Bone Tumor in the Cervical Spine Based on 3-Dimensional Printing Technology. Orthop Surg 2016;8:171-8. [Crossref] [PubMed]
- Guo F, Dai J, Zhang J, et al. Individualized 3D printing navigation template for pedicle screw fixation in upper cervical spine. PLoS One 2017;12:e0171509. [Crossref] [PubMed]
- Owen BD, Christensen GE, Reinhardt JM, et al. Rapid prototype patient-specific drill template for cervical pedicle screw placement. Comput Aided Surg 2007;12:303-8. [Crossref] [PubMed]
- Lu S, Xu YQ, Zhang YZ, et al. A novel computer-assisted drill guide template for lumbar pedicle screw placement: a cadaveric and clinical study. Int J Med Robot 2009;5:184-91. [Crossref] [PubMed]
- Lu S, Xu YQ, Zhang YZ, et al. A novel computer-assisted drill guide template for placement of C2 laminar screws. Eur Spine J 2009;18:1379-85. [Crossref] [PubMed]
- Lu S, Xu YQ, Chen GP, et al. Efficacy and accuracy of a novel rapid prototyping drill template for cervical pedicle screw placement. Comput Aided Surg 2011;16:240-8. [Crossref] [PubMed]
- Chen H, Wu D, Yang H, et al. Clinical Use of 3D Printing Guide Plate in Posterior Lumbar Pedicle Screw Fixation. Med Sci Monit 2015;21:3948-54. [Crossref] [PubMed]
- Chen H, Guo K, Yang H, et al. Thoracic Pedicle Screw Placement Guide Plate Produced by Three-Dimensional (3-D) Laser Printing. Med Sci Monit 2016;22:1682-6. [Crossref] [PubMed]
- Deng T, Jiang M, Lei Q, et al. The accuracy and the safety of individualized 3D printing screws insertion templates for cervical screw insertion. Comput Assist Surg (Abingdon) 2016;21:143-9. [Crossref] [PubMed]
- Huang KY, Lin RM, Fang JJ. A novel method of C1–C2 transarticular screw insertion for symptomatic atlantoaxial instability using a customized guiding block: A case report and a technical note. Medicine 2016;95:e5100. [Crossref] [PubMed]
- Liu K, Zhang Q, Li X, et al. Preliminary application of a multi-level 3D printing drill guide template for pedicle screw placement in severe and rigid scoliosis. Eur Spine J 2017;26:1684-9. [Crossref] [PubMed]
- Lu T, Liu C, Dong J, et al. Cervical screw placement using rapid prototyping drill templates for navigation: a literature review. Int J Comput Assist Radiol Surg 2016;11:2231-40. [Crossref] [PubMed]
- Otsuki B, Takemoto M, Fujibayashi S, et al. Utility of a custom screw insertion guide and a full-scale, color-coded 3D plaster model for guiding safe surgical exposure and screw insertion during spine revision surgery. J Neurosurg Spine 2016;25:94-102. [Crossref] [PubMed]
- Takemoto M, Fujibayashi S, Ota E, et al. Additive-manufactured patient-specific titanium templates for thoracic pedicle screw placement: novel design with reduced contact area. Eur Spine J 2016;25:1698-705. [Crossref] [PubMed]
- Sugawara T, Higashiyama N, Kaneyama S, et al. Accurate and Simple Screw Insertion Procedure With Patient-Specific Screw Guide Templates for Posterior C1-C2 Fixation. Spine (Phila Pa 1976) 2017;42:E340-E6. [Crossref] [PubMed]
- Phan K, Sgro A, Maharaj MM, et al. Application of a 3D custom printed patient specific spinal implant for C1/2 arthrodesis. J Spine Surg 2016;2:314-8. [Crossref] [PubMed]
- Wei R, Guo W, Ji T, et al. One-step reconstruction with a 3D-printed, custom-made prosthesis after total en bloc sacrectomy: a technical note. Eur Spine J 2017;26:1902-9. [Crossref] [PubMed]
- Xu N, Wei F, Liu X, et al. Reconstruction of the Upper Cervical Spine Using a Personalized 3D-Printed Vertebral Body in an Adolescent With Ewing Sarcoma. Spine (Phila Pa 1976) 2016;41:E50-4. [Crossref] [PubMed]
- Kim D, Lim JY, Shim KW, et al. Sacral Reconstruction with a 3D-Printed Implant after Hemisacrectomy in a Patient with Sacral Osteosarcoma: 1-Year Follow-Up Result. Yonsei Med J 2017;58:453-7. [Crossref] [PubMed]
- Mobbs RJ, Coughlan M, Thompson R, et al. The utility of 3D printing for surgical planning and patient-specific implant design for complex spinal pathologies: case report. J Neurosurg Spine 2017;26:513-8. [Crossref] [PubMed]
- Choy WJ, Mobbs RJ, Wilcox B, et al. Reconstruction of Thoracic Spine Using a Personalized 3D-Printed Vertebral Body in Adolescent with T9 Primary Bone Tumor. World Neurosurg 2017;105:1032.e13-e7. [Crossref] [PubMed]
- Baskaran V, Štrkalj G, Štrkalj M, et al. Current Applications and Future Perspectives of the Use of 3D Printing in Anatomical Training and Neurosurgery. Front Neuroanat 2016;10:69. [Crossref] [PubMed]
- Bova FJ, Rajon DA, Friedman WA, et al. Mixed-reality simulation for neurosurgical procedures. Neurosurgery 2013;73 Suppl 1:138-45. [Crossref] [PubMed]
- Wu AM, Shao ZX, Wang JS, et al. The accuracy of a method for printing three-dimensional spinal models. PLoS One 2015;10:e0124291. [Crossref] [PubMed]
- Liew Y, Beveridge E, Demetriades AK, et al. 3D printing of patient-specific anatomy: A tool to improve patient consent and enhance imaging interpretation by trainees. Br J Neurosurg 2015;29:712-4. [Crossref] [PubMed]
- Anderson PA. Clinical Applications of 3D Printing. Spine (Phila Pa 1976) 2017;42 Suppl 7:S30-S1. [Crossref] [PubMed]
- Shao ZX, Wang JS, Lin ZK, et al. Improving the trajectory of transpedicular transdiscal lumbar screw fixation with a computer-assisted 3D-printed custom drill guide. PeerJ 2017;5:e3564. [Crossref] [PubMed]
- Wu AM, Wang S, Weng WQ, et al. The radiological feature of anterior occiput-to-axis screw fixation as it guides the screw trajectory on 3D printed models: a feasibility study on 3D images and 3D printed models. Medicine (Baltimore) 2014;93:e242. [Crossref] [PubMed]
- Rong X, Wang BY, Deng YX, et al. Three-dimensional Printed Patient-specific Drilling Templates for Expansive Open-door Laminoplasty. Chin Med J (Engl) 2016;129:2644-6. [Crossref] [PubMed]
- Lin CL, Fang JJ, Lin RM. Resection of giant invasive sacral schwannoma using image-based customized osteotomy tools. Eur Spine J 2016;25:4103-7. [Crossref] [PubMed]
- Spetzger U, Frasca M, König SA. Surgical planning, manufacturing and implantation of an individualized cervical fusion titanium cage using patient-specific data. Eur Spine J 2016;25:2239-46. [Crossref] [PubMed]
- Haglin JM, Eltorai AE, Gil JA, et al. Patient-Specific Orthopaedic Implants. Orthop Surg 2016;8:417-24. [Crossref] [PubMed]
- Tritanium Manufacturing Overview. 2016. Available online: http://www.stryker.com/builttofuse/media/assets/TRITA-BR-2 Tritanium Manufacturing Overview FINAL.pdf
- Tritanium Posterior Lumbar Cage Technical Summary. 2016. Available online: http://www.stryker.com/builttofuse/media/assets/TRITA-BR-3 Tritanium Technical Summary FINAL.pdf.).
- Lamellar 3D Titanium Technology. 2015. Available online: http://www.k2m.com/platform-technologies/technology/lamellar-titanium-technology/
- 4WEB Medical Products. 2017. Available online: http://4webmedical.com/products/posterior-spine-truss-system/
- Figueroa-Cavazos JO, Flores-Villalba E, Diaz-Elizondo JA, et al. Design Concepts of Polycarbonate-Based Intervertebral Lumbar Cages: Finite Element Analysis and Compression Testing. Appl Bionics Biomech 2016;2016:7149182. [Crossref] [PubMed]