Effects of spaceflight on cartilage: implications on spinal physiology
Introduction
Since human space exploration began with Vostok 1 in 1961, astronauts, cosmonauts, and taikonauts have been subjected microgravity. Microgravity alters the normal physiology of cells and tissues observed on Earth (1-3). Study of these effects reveal several adverse effects in space voyagers, including cardiovascular dysfunction, impaired immune response, vision impairment, and genitourinary complications (4-8). Spaceflight also impacts the musculoskeletal system by reducing bone mineral density and causing atrophy of skeletal muscle (9-12). The effects of spaceflight on cartilage, however, are not well characterized. Astronauts who experience negative impacts to cartilage will be at risk for injury during landing either on earth or in a planetary surface. This cohort of astronauts may experience long term health effects and be at risk for chronic issues or injury long after spaceflight (13,14).
Cartilage is a durable, smooth connective tissue comprised of dense matrix of collagen and elastic fibers within a ground substance (15). As with nearly all musculoskeletal connective tissues, cartilage is derived from the mesoderm (16). At the cellular level, it is derived from progenitor chondroblasts. When chondroblasts become embedded in matrix, they are named chondrocytes (17). Uniquely, cartilage is avascular and contains no nerves, except in the perichondrium (18). There are three types of cartilage which differ in relative amounts of collagen and proteoglycan: elastic (outer ear, Eustachian tube, epiglottis), hyaline (joint surfaces), and fibrocartilage (intervertebral discs, pubic symphysis, menisci) (19-21). Most spaceflight studies of cartilage have focused on hyaline and fibrocartilage.
Basic science research probes at the cellular and molecular level through animal experiments, cell studies, biochemical, genetic, and physiological studies (22). On the other hand, clinical studies include experimental or observational studies which usually explore mechanisms at the organ system level involving human subjects (22). Furthermore, these studies can be subdivided into those occurring in spaceflight or those taking place in simulated microgravity on earth (23-25). While simulated microgravity provides a practical and accessible platform to study cartilage, it cannot account for external influences present during spaceflight, such as the effects of radiation and physiologic stress; simulated models also introduce factors including vessel vibration and differing gravity vectors not present in spaceflight (26-28).
The effects of spaceflight on various tissues is becoming more relevant as plans for long duration missions such as Mars become feasible. The effects on intervertebral discs and articular cartilage are of particular interest as astronauts prepare for longer spaceflight exposure (29-31). We review the current body of knowledge regarding effects of spaceflight on cartilage with a focus on effects of spaceflight on intervertebral disc changes or spinal physiology changes. The findings are subdivided into cartilage engineering, basic science experiments, and clinical studies, to provide a comprehensive systematic review and analysis.
Methods
Search strategy
A systematic review of the literature was conducted using PubMed, MEDLINE, and Google Scholar for studies identifying the effects of spaceflight on intervertebral disc changes or spinal physiology changes. However, because there was a paucity of studies regarding this specific focus, the literature searches and review was expanded to include effects of spaceflight on all types of cartilage. As such, all studies on cartilage in spaceflight between the years of 1990–2016 were included. The references of these studies and reviews were cross-references. Search terms utilized were: “cartilage”/“chondrocyte”/“chondroblast”/“spine”/“spinal”/“disc”/“intravertebral”/“nucleus pulposus” AND “microgravity”/“weightlessness”/“spaceflight”.
Selection criteria and data extraction
A literature review was conducted using the search terms. The inclusion criteria were broad to include all possible pertinent studies involving cartilage and spaceflight. Articles out of scope, simulated microgravity papers, case reports, reviews, articles not in English, and inaccessible full-text manuscripts were excluded.
Quality assessment and exclusion
Three investigators (V Ramachandran, R Wang, SS Ramachandran) independently reviewed the titles and abstracts of all studies pulled from the search terms for inclusion. After this initial review, full manuscripts of the remaining included studies were obtained and re-assessed for inclusion. The information extracted for each paper included the author, publication year, study design, study population characteristics, and results.
Articles were placed into three categories by best fit reflecting: (I) cartilage engineering; (II) basic science studies; and (III) clinical studies.
Results
Literature review
The PRISMA methodology was utilized (Figure 1) (32).
Study characteristics
A total of 15 articles were included. These papers were divided into three categories: tissue engineering (13%), clinical studies (13%), and basic science (74%) (Table 1). Summaries of the studies included in this investigation are found in Table 2.
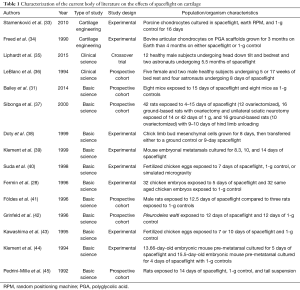
Full table
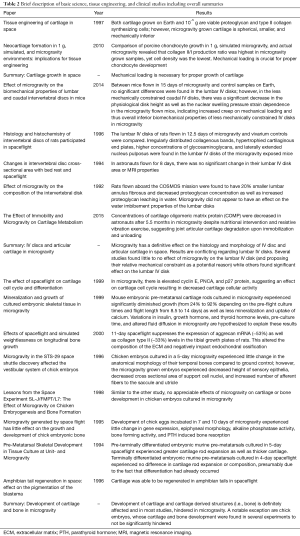
Full table
Tissue engineering studies
Researchers have attempted tissue engineering in space to elucidate the viability of differentiating cells and to assess the effects of spaceflight on tissues at the cellular level. Two such experiments have been performed, comparing samples in spaceflight, simulated microgravity, and normal control cartilage (33,34).
In a landmark study, Freed et al. grew bovine articular chondrocytes on polyglycolic acid scaffolds in rotating bioreactors for 3 months on Earth (34). This was followed by 4 months of growth aboard the Mir Space Station (10−4 to 10−6g) or on Earth (1 g control). Compared to natural cartilage, both the spaceflight and Earth-grown samples lagged approximately 4-fold in terms of chondrocyte function as measured by sulfate and proline incorporation. The spaceflight cartilage maintained its chondrogenic phenotype as measured by the amount of collagen type II as a fraction of the total wet weight. Aggregate modulus is a measure of the stiffness of a tissue when all fluid flow ceases. The higher the aggregate modulus, the less the tissue deforms under a given load. The Earth-grown cartilage was indistinguishable from natural cartilage in terms of aggregate modulus whereas the spaceflight cartilage aggregate modulus lagged 3-fold. The spaceflight constructs also had significantly lower glycosaminoglycan (GAG) content than the Earth-grown constructs and natural cartilage. The authors suggest that the aggregate modulus is determined mainly by GAG content. In addition, physical forces inducing mechanotransduction at the cellular level were present in the Earth-grown constructs, likely explaining the lower wet weights, GAG fractions, aggregate modulus, and more spherical shape of the spaceflight constructs (34).
Stamenković et al. studied the effects of spaceflight and simulated microgravity on scaffold-free cartilage development (33). Porcine chondrocytes (100×106 cells/mL) were inoculated into cylindrical chambers and exposed to one of three conditions: (I) microgravity of the Flight 7S (Cervantes mission) of the International Space Station (ISS); (II) simulated microgravity of a random positioning machine (RPM); or (III) normal gravity control (1 g) for 16 days. There were several findings. Immunohistochemistry analyses revealed weaker staining of the extracellular matrix in the ISS cartilage tissue compared to both RPM and 1-g samples. Cell density was reduced in spaceflight samples. Aggrecan is a cartilage-specific proteoglycan core protein while versican is a generalized proteoglycan found throughout the bodies of vertebrates (46,47). The 1-g controls had a higher aggrecan:versican gene expression ratio compared to both ISS and RPM samples. The ISS sample had the lowest aggrecan:versican gene expression ratio. Quantitative polymerase chain reaction (qPCR) revealed that collagen II:I gene expression ratio was highest in ISS samples despite immunostaining revealing that collagen II protein content was actually lowest in the ISS samples. The authors attributed this paradoxical finding to the negative effect of microgravity on post-translational modifications of collagen and a potential re-adaptation to gravity upon return of the ISS sample (33).
Clinical studies
Clinical studies conducted during spaceflight allow examination of human cartilage response to the space environment, possibly leading to the development of countermeasures or crossover application on Earth.
LeBlanc et al. investigated the effects of immobilization and spaceflight on fibrocartilage of the Intervertebral discs with subjects in three separate environments: one night of diurnal bedrest simulating regular sleep patterns (control), extended bedrest (5 weeks or 17 weeks with 6–7 weeks of an ambulatory recovery period), and 8 days aboard shuttle flight SL-J (36). Magnetic resonance imaging (MRI) was used to measure cross-sectional changes in area of the L2–L3 and L3–L4 discs and the transverse relaxation time (T2) of the intervertebral discs. The subjects who underwent 5- and 17-week bedrest protocols saw statistically significant increases in the entire disc area. Additionally, disc changes reached a steady state (+22% in area, range of 10–40%) by approximately 4 days of bedrest. Post-bedrest values were compared to pre-bedrest values. Only the 17-week cohort maintained significantly increased disc areas 6–7 weeks after conclusion of the study. Conversely, the change in disc areas of the SL-J astronauts (the only subjects exposed to actual spaceflight) did not change. There were no measureable changes in T2 or lumbar spine heights seen in any of the four astronauts 24 hours after they returned from 8 days of spaceflight (36). These findings suggest characterization of a known limitation in bedrest studies is possible. This is useful for determining when such studies are appropriate.
Liphardt et al. investigated the effects of spaceflight on articular cartilage (35). Cartilage oligomeric matrix protein (COMP), an established biomarker for monitoring cartilage metabolism in relation to joint degradation and disease, is sensitive to mechanical loading (48,49). This marker was used to evaluate the effects of: (I) head down tilt and bedrest (HDT-BR) for 21 days in twelve healthy male subjects and (II) 5.5 months of spaceflight in two astronauts. In the HDT-BR subjects, COMP concentrations decreased within the first day of study, but increased to baseline levels after the individuals became ambulatory at the conclusion of the study. Nutrition and resistive vibration exercises did not affect these changes. Preliminary investigation of the COMP concentrations in the astronauts revealed a substantial increase (+39.9% and +13.3%) from baseline 7 days after return to Earth. The levels remained elevated until 30 days after landing (35). This suggests that astronauts may be at greater risk for cartilage degeneration, including degenerative disc disease, and arthritis with long-duration spaceflight.
Basic science studies
Basic science research has been the predominant medium in which the effects of spaceflight on cartilage have been investigated.
Bailey et al. used C57BL/C mice to examine the effects of spaceflight on the biomechanical properties of lumbar and caudal discs of mice after 15 days aboard the STS-131 shuttle mission (31). Variables measured included the physiological disc height (PDH), endplate permeability (K), nuclear swelling pressure dependence (D) and annular viscoelasticity (G). The mice were sacrificed upon landing and measurements were compared to controls on Earth. No significant differences were observed in the lumbar intervertebral discs of the mice aboard the STS-131 and the control mice. However, the caudal intervertebral discs of the STS-131 mice had a decrease in PDH (−32%) and a decrease in D (−70%). The other caudal segment properties (K and G) did not appear to be influenced by spaceflight (31). The authors postulate that weightlessness coupled with different motions along the spine may help explain the relatively high rate of cervical disc herniation amongst astronauts (31).
Földes et al. exposed Wistar rats to 12.5 days of spaceflight aboard the COSMOS 1887 biosatellite (41). Histologic and histochemical analyses of the lumbar intervertebral discs were performed. The spaceflight rats demonstrated irregularly distributed bands of collagen in the external zone of annulus fibrosus and cartilage end plate. Cartilaginous end plates had hypertrophied with mild mineralization. There was also lateral extension of notochordal cells in the nucleus pulposus compared to controls, in which chondroid cells dominated. Mucopolysaccharides and glycoproteins also showed changes in the spaceflight samples. There was a significant increase in the orientation of GAGs in the external and internal zones of the annulus fibrosus and nucleus pulposus. The authors state that the observed alterations in macromolecular components of intervertebral discs, cartilage ends plates, and osteoporotic changes may lead to the spinal pathologies faced by astronauts (41).
Pedrini-Mille et al. involving the intervertebral discs of rats aboard the COSMOS 2044 mission (45). This study revealed that the annulus fibrosus of rats aboard the spaceflight mission weighed significantly less (−20%) than those of Earth-based (1 g) controls. Further, the collagen:proteoglycan ratio was significantly greater in the spaceflight cohort. There were no observed changes in the relative proportions of type I and II collagen or in the number of cross-links. In this study, the annuli were also submerged underwater for 2 hours to observe for leaching of proteoglycans into the fluid. Significantly more proteoglycans leached from the annuli of the spaceflight rats than controls, which the authors propose was due to abnormal or smaller proteoglycans. The authors posit that these changes may affect the biomechanical functions of the annulus (45).
Doty et al. addressed whether decreases in cellular activity in space are due to effects of spaceflight on the differentiated cell, on the cell cycle and proliferation process, or on apoptosis (38). Chick limb bud mesenchymal cells were grown for 8 days and then divided into either 1-g control or spaceflight groups aboard the STS-95 for nine additional days. Post-flight samples were analyzed using flow cytometry, immunocytochemistry, and measurement of glucose and lactate in the growth media. Flow cytometry was performed for proliferating cell nuclear antigen (PCNA), cyclin E, p27, relative cell size, DNA content, and percentage of cells undergoing apoptosis. In the cell cycle, cyclin E complexes with cyclin dependent kinase 2 (CDK2) to allow for transition from G1 to S phase of the cell cycle (50). Cyclin D also promotes the G1/S transition (51). PCNA is a DNA polymerase delta co-factor and increases processivity of leading strand DNA synthesis. PCNA is also involved in the RAD6-dependent DNA repair pathway (52). p27, also known as cyclin dependent kinase inhibitor 1B, is a cell cycle inhibitor protein which inhibits progression at G1 by binding to complexes such as cyclin E-CDK2 (53). Flow cytometry revealed that the flight group cells contained significantly elevated cyclin E (P=0.03), PCNA (P=0.08), and paradoxically p27 (P=0.005), as well as a lower proportion of cells in G1 compared to the ground control cells. Immunohistology did not reveal significant results. Media analysis indicated that the cartilage cells continuously metabolized glucose to lactate throughout the 9-day flight. Based on this data, the authors suggested that apoptosis did not play a role in reduced cellularity. The authors further suggested that spaceflight affects the cell cycle rather than cell death since cell cycle proteins appeared to be elevated and a difference in number of cells in G1 was found between flight and control cells (38).
Klement and colleagues studied mouse embryonic metatarsal cartilage rods cultured in spaceflight, collected from the STS-62, 63, and 67 space shuttle missions (39). Rod growth after the 14-day STS-62 mission without standard lab pre-culture showed a 24% decrease in growth relative to ground controls. The STS-63 mission lasted 8.3 days and included three experiments: a 5-day standard lab pre-cultured sample, a non-precultured sample, and an 11-day pre-cultured sample with radiolabeled calcium added at day 7. The non-precultured rods showed a 92% decrease in growth relative to the ground controls while the pre-cultured rods showed a 46% decrease in growth relative to the ground controls. Furthermore, the STS-63 results demonstrated less mineralization and uptake of radioactively-labeled calcium in the spaceflight group compared to ground controls, creating differences between the two groups in mineralized matrix composition. The STS-77 mission lasted for 10 days and used 6-day pre-cultured samples. The rods grown on the STS-77 mission were similar in length to the ground control rods. The authors postulated several theories to explain their results. The hindered actions of hormones including insulin-like growth factor-1, growth hormone, and thyroid hormone were hypothesized to restrict cartilage growth. Pre-culture time clearly played a significant role in the results. Additionally, microgravity affects fluid diffusion and convection, which may have affected radioactive calcium uptake and release, possibly due to differences in proteoglycan content or a suppression of the outward growth of the stable crystal nucleus (39).
Sibonga et al. performed a series of spaceflight experiments to investigate the effect on longitudinal bone growth (37). Eleven days of spaceflight suppressed the expression of aggrecan mRNA by 53% (P<0.05) in the tibial growth plates of 12 male rats (7–8 weeks in age) as determined by northern hybridization. The authors hypothesize that this altered the composition of the extracellular matrix. They also hypothesized that similar changes may have contributed to the observed 34% reduction (P<0.05) in longitudinal growth rate of the tibia in a cohort of nine 13-week-old rats with ovaries removed were subjected to hind-limb unloading for 8 days (i.e., simulated microgravity) compared to weight bearing control rats (37).
Fermin et al. investigated the effects of a 5-day spaceflight aboard the STS-29 on the vestibular system of chick embryos (28). Sixteen 2-day (E2) and 16 9-day (E9) embryos within eggs were selected for the flight along with identical aged Earth-based 1-g controls. Of the 32 embryos flown in space (16 E2 and 16 E9), only 16 survived (all 16 E2 were dead at landing). All 16 E9 survived and were without morphologic abnormalities. The ground controls were exposed to vibrations similar to those experienced aboard the shuttle. The study revealed several findings. Staining of keratan sulfate, chondroitin sulfate, and fibronectin (all of which are extracellular components of cartilage) did not show a difference between flight and ground samples. The gross morphology of the temporal bone, within which resides the vestibule, did not differ between flight and ground controls. There was no difference in the cross-sectional areas or circularity of cartilage cells of the temporal bones (28).
Kawashima et al. utilized fertilized chick eggs in their study (43). The eggs were incubated during spaceflight for seven and 10 days while controls were maintained on Earth in both an incubator akin to that used in space and in a standard commercially available incubator. Light microscope examination with Alcian blue staining of the chick embryo humeri and tibias showed the quantity and distribution of osteoid tissues and morphologic structure of the epiphysis were not different between the groups. Furthermore, the bone-resorbing and bone-forming activities and calcium and phosphorus composition of the femur were unchanged. Study of parathyroid hormone induced bone resorption at the femoral diaphysis was unchanged between the groups. Spaceflight also did not alter the length or wet weight of the bones at any stage of embryo. Kawashima and colleagues also demonstrated that the alkaline phosphatase activity of the tibia (divided into three sections: resting cartilage, growth plate and cortical bone) did not differ. At the molecular level, gene expressions in digits and tarsometatarsals of the hind limbs were studied using in situ hybridization. This investigation showed no difference in the expression of hepatocyte growth factor and receptors of fibroblast growth factor in perichondrium, trabecula, and skeletal muscles and tendons of hind limbs (43).
Suda et al. assessed effects of pre-launch incubation on fertilized chicken eggs (40). Thirty fertilized chicken eggs were incubated for 0 (n=10), 7 (n=10) and 10 days (n=10) prior to launch aboard the space shuttle Endeavor. They were incubated for an additional 7 days in spaceflight. Control groups were placed at the 1 g of Earth and in simulated microgravity. Twenty out of 30 flight eggs were recovered after landing (nine 10-day-old eggs, ten 7-day-old eggs, one 0-day-old egg). Investigation of the 0-day-old embryos revealed that these eggs developed a low amount of blood vessels in the chorioallantoic membrane in comparison to the control group. While the yolk (specific gravity, 1.029) would not have separated from the albumen (1.040) during space flight, the authors state that the subtle difference in specific gravity appeared to play a critical role in early chick embryogenesis of the 0-day-old embryos. However, all the tissues, including cartilage and bone, formed normally in the 7- and 10-day-old chicken embryos during spaceflight. There was only a slight decrease in bone density and changes in cartilage organization for the 7-day incubated embryos that were in the spaceflight environment. There were minor changes in in the deposition of type XI collagen and the distribution of type II collagen. Furthermore, when the 7- and 10-day-old spaceflight embryos were recovered at the Kennedy Space Center and incubated on Earth, they hatched normally. The authors did not suggest a reason for these unexpected results (40).
Klement and Spooner used embryonic mouse pre-metatarsals that had already initiated chondrogenesis and morphogenetic patterning in their study (44). First, the authors analyzed the growth and morphogenesis of pre-metatarsals after a 5-day spaceflight aboard the STS-54 mission via the orbiter Endeavor. In comparison to controls, spaceflight histologic sections of embryonic pre-metatarsals (13.66 days old) showed greater cartilage rod expansion in length and width. Serial section analysis of the rods demonstrated thicker cartilage than zero-time controls. Morphogenetic order was maintained and normal cartilage phenotype was continually expressed in the spaceflight samples. However, the authors deemed this initial spaceflight study inadequate to assess for terminal chondrocyte differentiation or mineralization. A subsequent experiment investigated the effects of a 4-day spaceflight (STS-56 via the space shuttle Discovery) on older embryonic pre-metatarsals (15.5 days old), which had already terminally differentiated into hypertrophied cartilage. The pre-metatarsal tissues maintained their structure and normal staining of cartilage extracellular matrix. Paralleling controls, the spaceflight cultures showed no further growth of the cartilage rods and no calcification (44).
Grinfeld et al. conducted an experiment using Urodele amphibians, organisms in which tails regenerate after transection (42). In order to regenerate different tissues are propagated, including bone from the vertebrae, muscles, epidermis, and nervous system components. This process of regeneration was studied during spaceflight aboard the Bion-10 satellite. The results were compared to synchronous controls on Earth. The investigators found that cartilage, regeneration, just like spinal cord and muscle tissues, occurred in spaceflight subjects just as in the controls (42).
Discussion
In the course of the literature review, it became evident that there is a shortage of literature regarding the effects of spaceflight on spinal physiology. Only four were identified addressing this focus. As such, the review was expanded to include all cartilage studies in spaceflight. However, these findings still have implications on our understanding of the effects on the spine and intervertebral discs, which is important given plans for long-duration spaceflight to Mars.
Evaluating the broad effects of spaceflight on cartilage brings several findings to light. First, the majority of studies were basic science in nature (11 out of 15). For instance, the work on cartilage development and metabolism was concentrated in animal studies with just one human study. The findings in basic science papers were at times inconsistent with one another and occasionally conflicted with results elucidated from clinical studies. For example, astronauts showed an increase in COMP after 5.5 months (i.e., relatively long term) in spaceflight, suggesting that astronauts may be at greater risk for cartilage degeneration, including degenerative disc disease, and arthritis with long-duration spaceflight (35). However, several basic science studies using animal models revealed some variation in results. For instance, the development of cartilage and cartilage derived structures (i.e., bone) was shown in most studies to be hindered in spaceflight (37,39). However, a notable exception was chick embryos, whose cartilage and bone development were not significantly hindered in several experiments (40).
Similarly, the present work on intervertebral discs has been concentrated in mice and rat studies with only one human study. The human SL-J spaceflight study appeared to be show no change in the lumbar IV disk parameters; however, it was only for a period of 8 days (36). Alternatively, rat and mice studies indicate either a decrease or malformation in the size of the lumbar IV disk with accompanying changes in proteoglycan content. Overall, it is difficult to conclusively determine the effect of spaceflight on human intervertebral disk fibrocartilage based on the current literature. Additional human research, ideally prospective spaceflight studies with ground-based controls, are needed to adequately elucidate the impact of spaceflight on this tissue.
The sometimes dissimilar findings of basic science studies in spaceflight may be attributed to variations in study design, including differences in model organisms used, duration of missions, and differences in ages of embryos or organisms used. For example, longer pre-culture times of chick embryos resulted in improved growth in spaceflight (39,44). Given the current dearth of human spaceflight cartilage studies, this variation is especially important to consider if attempting to extrapolate data for human application. At the present moment, such extrapolation to human physiology is not advisable based on the lack of consistent results.
In order to bridge the divide between basic science research and clinical studies conducted in space, further efforts and funding should be directed towards translational studies. The goal of translational research is to apply findings from spaceflight basic science studies to the design of robust human studies, and eventually countermeasure development, if warranted (54). To this extent, lifetime surveillance of astronaut health data on joint or back injuries in spaceflight are critical to obtain in order to elucidate if differences exist when compared to ground-based cohorts. Some experiments, such as tissue engineering studies, begin to do this. The two cartilage engineering papers surveyed illustrated that although metabolically viable cartilage cells could be grown in spaceflight, mechanical loading under normal gravity is critical for the development of mechanically sound cartilage (33,34). However, there is room to build upon these studies. For instance, both groups of researchers remarked that using a 1-g centrifuge in spaceflight as a control and freezing spaceflight samples before landing (to cancel out any effect of the tissue as a result of re-adaptation to gravity) would have provided more experimental control, but was not feasible given weight and size constraints. Furthermore, the small sample sizes limited definitive conclusions.
Due to an aging population and increased incidence of sports-related injuries, musculoskeletal disorders such as degenerative disc disease and cartilage injuries cause significant health burdens in the United States (55). Traditional therapies such as intervertebral disc fusion and arthroplasty, allograft, and autograft have shortcomings due to cost, supply, infection, surgical morbidity, and risk of rejection (56,57). This has led to increasing interest into tissue engineering in orthopaedic surgery (58). Translational space research has contributed to advancements in terrestrial research, such as tissue engineering using simulated microgravity on Earth (34,59,60). Future translational research into countermeasure development against the effects of spaceflight on cartilage, such as articular degeneration observed in astronauts during long-term spaceflight (35), may yield beneficial cross-over knowledge in understanding and treating terrestrial diseases with a high global burden like vertebral disc degeneration and osteoarthritis (61,62).
The CARTILAGE study by the National Aeronautics and Space Administration (NASA) is an ongoing experiment exploring the effect of microgravity on the articular cartilage of the knees and begins to address the limited human cartilage studies in space (63). Data collection consists of knee MRIs pre- and post-flight as well as urine samples post-flight to assess for the presence of cartilage biodegradation markers. The CARTILAGE study is an effort to address several issues brought up in this paper. However, the effect of long-term spaceflight on fibrocartilage of human intervertebral discs as well as on human development of cartilage are yet to be well studied. This is particularly important when considering possible deleterious effects during extended space voyages to Mars and beyond.
There are several limitations of the present study. In conducting this review there were five articles not published in English that could not be incorporated along with inaccessible full-text manuscripts through academic directories or library databases. We recommend that the aerospace and biomedical communities consider the benefits of open access material to facilitate distribution of findings, enhanced collaboration, and identifying gaps in the literature for future studies. Furthermore, the significant variation in methodology, experimental organism, length of study, techniques used, and the results themselves limits our ability to articulate definite and consistent findings from our analysis. Only three studies on cartilage in spaceflight have been published this decade, signifying the dearth of literature in this important domain. Additionally, while the effects of spaceflight on intervertebral discs may be extrapolated from other investigations of cartilage in space, these hypotheses have clear limitations.
Conclusions
Since forays into spaceflight began in the 1960s, simultaneously interesting and deleterious effects of the space environment on human physiology have been uncovered. Much research has focused on phenomena such as visual impairment, immune dysfunction, and skeletal changes (5,6,9). In comparison, cartilage research has received relatively modest attention, leading to the current shortage of robust information regarding its behavior in the environment of space. Our study demonstrates several themes present at the gross tissue level, individual cell level, and molecular level. Aggregate cartilage tissues such as intervertebral discs show irregular distribution of cartilage in endplates, decreased physiologic disc height, and decreased annulus fibroses weight in rat lumbar discs. Mouse metatarsal rod cartilage demonstrated decreased growth and decreased calcium uptake while rat tibial growth plate cartilage exhibited decreased aggrecan mRNA expression. At the cartilage cell level, decreases were found in cell density in porcine models, stiffness in bovine models, and mineralization in rat models. No changes were found in fertilized chick embryos with respect to epiphysis morphology, osteoid tissue quantity or distribution, alkaline phosphatase levels, bone length, or calcium and phosphate levels. At the molecular level, studies showed disparate results with decreased GAG content in bovine chondrocytes, no differences in chick embryo keratan sulfate, chondroitin sulfate, or fibronectin, changes in rat glycoproteins and mucopolysaccharides, and increased markers of cartilage metabolism in human research. The broad take-away is that cartilaginous tissue generally undergoes changes upon exposure to spaceflight with respect to physical properties, organization, cell cycle pathways, and associated macromolecules. However, the variability in duration of spaceflight exposure, model organisms, and parameters analyzed obfuscates concrete knowledge about the exact behavior of this tissue. Although short duration flyers have not necessarily experienced clinical consequences of cartilage effects in spaceflight, this will become more interesting for longer duration spaceflight, interplanetary flight, and commercial customers who may not be in peak health in the future. Future research, particularly larger human studies with ground-based controls, is needed to adequately shed light on the changes in cartilage structure and function in outer space. A specific focus of these future studies should include the effects of spaceflight on spine physiology and intervertebral discs.
Acknowledgements
None.
Footnote
Conflicts of Interest: The authors have no conflicts of interest to declare.
References
- Hughes-Fulford M. Altered cell function in microgravity. Exp Gerontol 1991;26:247-56. [Crossref] [PubMed]
- Matía I, González-Camacho F, Herranz R, et al. Plant cell proliferation and growth are altered by microgravity conditions in spaceflight. J Plant Physiol 2010;167:184-93. [Crossref] [PubMed]
- Crawford-Young SJ. Effects of microgravity on cell cytoskeleton and embryogenesis. Int J Dev Biol 2006;50:183-91. [Crossref] [PubMed]
- Charles JB, Lathers CM. Cardiovascular Adaptation to Spaceflight. J Clin Pharmacol 1991;31:1010-23. [Crossref] [PubMed]
- Cogoli A. Space flight and the immune system. Vaccine 1993;11:496-503. [Crossref] [PubMed]
- Zhang LF, Hargens AR. Intraocular/intracranial pressure mismatch hypothesis for visual impairment syndrome in space. Aviat Space Environ Med 2014;85:78-80. [Crossref] [PubMed]
- Pietrzyk RA, Jones JA, Sams CF, et al. Renal stone formation among astronauts. Aviat Space Environ Med 2007;78:A9-13. [PubMed]
- Jones JA, Jennings RT, Pietryzk R, et al. Genitourinary issues during spaceflight: a review. Int J Impot Res 2005;17 Suppl 1:S64-7. [Crossref] [PubMed]
- Sibonga JD. Spaceflight-induced bone loss: Is there an Osteoporosis Risk? Curr Osteoporos Rep 2013;11:92-8. [Crossref] [PubMed]
- Sibonga JD, Evans HJ, Sung HG, et al. Recovery of spaceflight-induced bone loss: Bone mineral density after long-duration missions as fitted with an exponential function. Bone 2007;41:973-8. [Crossref] [PubMed]
- Vandenburgh H, Chromiak J, Shansky J, et al. Space travel directly induces skeletal muscle atrophy. FASEB J 1999;13:1031-8. [Crossref] [PubMed]
- Keller TS, Strauss AM, Szpalski M. Prevention of bone loss and muscle atrophy during manned space flight. Microgravity Q 1992;2:89-102. [PubMed]
- Willey JS, Kwok AT, Moore JE, et al. Spaceflight-Relevant Challenges of Radiation and/or Reduced Weight Bearing Cause Arthritic Responses in Knee Articular Cartilage. Radiat Res 2016;186:333-44. [Crossref] [PubMed]
- McCabe NP, Androjna C, Hill E, et al. Simulated microgravity alters the expression of key genes involved in fracture healing. Acta Astronaut 2013;92:65-72. [Crossref]
- Sophia Fox AJ, Bedi A, Rodeo SA. The basic science of articular cartilage: structure, composition, and function. Sports Health 2009;1:461-8. [Crossref] [PubMed]
- Glenister TW. An embryological view of cartilage. J Anat 1976;122:323-30. [PubMed]
- Hall BK. Cartilage V1: Structure, Function, and Biochemistry. Academic Press, 2012:58-78.
- Hall BK. Cartilage V1: Structure, Function, and Biochemistry. Academic Press, 2012:281-307.
- Cox RW, Peacock MA. The growth of elastic cartilage. J Anat 1979;128:207-13. [PubMed]
- Bhosale AM, Richardson JB. Articular cartilage: structure, injuries and review of management. Br Med Bull 2008;87:77-95. [Crossref] [PubMed]
- Benjamin M, Ralphs JR. Biology of Fibrocartilage Cells. Int Rev Cytol 2004;233:1-45. [Crossref] [PubMed]
- Röhrig B, du Prel JB, Wachtlin D, et al. Types of study in medical research: part 3 of a series on evaluation of scientific publications. Dtsch Arztebl Int 2009;106:262-8. [PubMed]
- Grimm D, Grosse J, Wehland M, et al. The impact of microgravity on bone in humans. Bone 2016;87:44-56. [Crossref] [PubMed]
- Watenpaugh DE. Analogs of microgravity: head-down tilt and water immersion. J Appl Physiol (1985) 2016;120:904-14. [Crossref] [PubMed]
- Wuest SL, Richard S, Kopp S, et al. Simulated microgravity: critical review on the use of random positioning machines for mammalian cell culture. Biomed Res Int 2015;2015. [Crossref] [PubMed]
- Hellweg CE, Baumstark-Khan C. Getting ready for the manned mission to Mars: The astronauts’ risk from space radiation. Naturwissenschaften 2007;94:517-26. [Crossref] [PubMed]
- Brenner DJ, Elliston CD. The potential impact of bystander effects on radiation risks in a Mars mission. Radiat Res 2001;156:612-7. [Crossref] [PubMed]
- Fermin CD, Martin D, Jones T, et al. Microgravity in the STS-29 Space Shuttle Discovery affected the vestibular system of chick embryos. Histol Histopathol 1996;11:407-26. [PubMed]
- Belavy DL, Adams M, Brisby H, et al. Disc herniations in astronauts: What causes them, and what does it tell us about herniation on earth? Eur Spine J 2016;25:144-54. [Crossref] [PubMed]
- Johnston SL, Campbell MR, Scheuring R, et al. Risk of herniated nucleus pulposus among U.S. astronauts. Aviat Space Environ Med 2010;81:566-74. [Crossref] [PubMed]
- Bailey JF, Hargens AR, Cheng KK, et al. Effect of microgravity on the biomechanical properties of lumbar and caudal intervertebral discs in mice. J Biomech 2014;47:2983-8. [Crossref] [PubMed]
- Moher D, Shamseer L, Clarke M, et al. Preferred reporting items for systematic review and meta-analysis protocols (PRISMA-P) 2015 statement. Syst Rev 2015;4:1. [Crossref] [PubMed]
- Stamenković V, Keller G, Nesic D, et al. Neocartilage formation in 1 g, simulated, and microgravity environments: implications for tissue engineering. Tissue Eng Part A 2010;16:1729-36. [Crossref] [PubMed]
- Freed LE, Langer R, Martin I, et al. Tissue engineering of cartilage in space. Proc Natl Acad Sci U S A 1997;94:13885-90. [Crossref] [PubMed]
- Liphardt AM, Bruggemann GP, Hamann N, et al. The effect of immobility and microgravity on cartilage metabolism. Ann Rheum Dis 2015;74:919. [Crossref]
- LeBlanc AD, Evans HJ, Schneider VS, et al. Changes in intervertebral disc cross-sectional area with bed rest and space flight. Spine (Phila Pa 1976) 1994;19:812-7. [Crossref] [PubMed]
- Sibonga JD, Zhang M, Evans GL, et al. Effects of spaceflight and simulated weightlessness on longitudinal bone growth. Bone 2000;27:535-40. [Crossref] [PubMed]
- Doty SB, Stiner D, Telford WG. The effect of spaceflight on cartilage cell cycle and differentiation. J Gravit Physiol 1999;6:89-90. [PubMed]
- Klement BJ, Spooner BS. Mineralization and growth of cultured embryonic skeletal tissue in microgravity. Bone 1999;24:349-59. [Crossref] [PubMed]
- Suda T. Lessons from the space experiment SL-J/FMPT/L7: the effect of microgravity on chicken embryogenesis and bone formation. Bone 1998;22:73S-78S. [Crossref] [PubMed]
- Földes I, Kern M, Szilágyi T, et al. Histology and histochemistry of intervertebral discs of rats participated in spaceflight. Acta Biol Hung 1996;47:145-56. [PubMed]
- Grinfeld S, Foulquier F, Mitashov V, et al. Amphibian tail regeneration in space: effect on the pigmentation of the blastema. Adv Space Res 1996;17:237-40. [Crossref] [PubMed]
- Kawashima K, Yamaguchi A, Shinki T, et al. Microgravity generated by space flight has little effect on the growth and development of chick embryonic bone. Biol Sci Space 1995;9:82-94. [Crossref] [PubMed]
- Klement BJ, Spooner BS. Pre-metatarsal skeletal development in tissue culture at unit- and microgravity. J Exp Zool 1994;269:230-41. [Crossref] [PubMed]
- Pedrini-Mille A, Maynard JA, Durnova GN, et al. Effects of microgravity on the composition of the intervertebral disk. J Appl Physiol 1992;73:26S-32S. [Crossref] [PubMed]
- Kiani C, Chen L, Wu YJ, et al. Structure and function of aggrecan. Cell Res 2002;12:19-32. [Crossref] [PubMed]
- Wight TN. Versican: a versatile extracellular matrix proteoglycan in cell biology. Curr Opin Cell Biol 2002;14:617-23. [Crossref] [PubMed]
- Saxne T, Heinegård D. Cartilage oligomeric matrix protein: a novel marker of cartilage turnover detectable in synovial fluid and blood. Br J Rheumatol 1992;31:583-91. [Crossref] [PubMed]
- Mündermann A, Dyrby CO, Andriacchi TP, et al. Serum concentration of cartilage oligomeric matrix protein (COMP) is sensitive to physiological cyclic loading in healthy adults. Osteoarthr Cartil 2005;13:34-8. [Crossref] [PubMed]
- Chartier NT, Oddou CI, Lainé MG, et al. Cyclin-dependent kinase 2/cyclin E complex is involved in p120 catenin (p120ctn)-dependent cell growth control: A new role for p120ctn in cancer. Cancer Res 2007;67:9781-90. [Crossref] [PubMed]
- CCND1 cyclin D1 [Homo sapiens (human)]. NCBI, 2017. Available online: https://www.ncbi.nlm.nih.gov/gene/595
- PCNA proliferating cell nuclear antigen [Homo sapiens (human)]. NCBI, 2017. Available online: https://www.ncbi.nlm.nih.gov/gene/5111
- Chu IM, Hengst L, Slingerland JM. The Cdk inhibitor p27 in human cancer: prognostic potential and relevance to anticancer therapy. Nat Rev Cancer 2008;8:253-67. [Crossref] [PubMed]
- Rubio DM, Schoenbaum EE, Lee LS, et al. Defining translational research: Implications for training. Acad Med 2010;85:470-5. [Crossref] [PubMed]
- Kahlenberg CA, Nair R, Monroe E, et al. Incidence of injury based on sports participation in high school athletes. Phys Sportsmed 2016;44:269-73. [Crossref] [PubMed]
- Ng VY. Risk of disease transmission with bone allograft. Orthopedics 2012;35:679-81. [Crossref] [PubMed]
- Cerulli G, Placella G, Sebastiani E. ACL Reconstruction: Choosing the Graft. Joints 2013;1:18-24. [PubMed]
- Laurencin CT, Ambrosio AM, Borden MD, et al. Tissue engineering: orthopedic applications. Annu Rev Biomed Eng 1999;1:19-46. [Crossref] [PubMed]
- Barzegari A, Saei AA. An update to space biomedical research: Tissue engineering in microgravity bioreactors. BioImpacts 2012;2:23-32. [PubMed]
- Grimm D, Wehland M, Pietsch J, et al. Growing tissues in real and simulated microgravity: new methods for tissue engineering. Tissue Eng Part B Rev 2014;20:555-66. [Crossref] [PubMed]
- Deane JA, McGregor AH. Current and future perspectives on lumbar degenerative disc disease: a UK survey exploring specialist multidisciplinary clinical opinion. BMJ Open 2016;6. [Crossref] [PubMed]
- Cross M, Smith E, Hoy D, et al. The global burden of hip and knee osteoarthritis: estimates from the Global Burden of Disease 2010 study. Ann Rheum Dis 2014;73:1323-30. [Crossref] [PubMed]
- CARTILAGE (CARTILAGE) - 11.22.16. NASA. 2016. Available online: https://www.nasa.gov/mission_pages/station/research/experiments/872.html