Case series of posterior instrumentation for repair of burst lumbar vertebral body fractures with entrapped neural elements
Introduction
White and Panjabi (1) put forward a landmark definition of instability of thoracolumbar burst fractures, combining clinical and biomechanical perspectives, as inability to maintain structural integrity under physiological loads to prevent a progression of neurological deficit and pain. Thoracolumbar spine burst vertebral body fractures represent a spectrum of traumatic spinal injuries that usually occur because of axial loading and some flexion compression injuries of the spine. It results from the failure of at least the anterior and middle columns of a vertebral segment (2). These frequently involve the lower thoracic and lumbar vertebrae, likely due to the high compression forces generated by the weight of the relatively more rigid thoracic rib cage and pelvis on a region that acts as a fulcrum connecting an immobile to mobile portion of the spinal column. They are seen commonly in patients with falls from height and high speed traffic collisions in restrained passengers, or with sudden deceleration (3). Over the previous 2 years, our group has accumulated experience with a particular type of traumatic burst vertebral body fracture, namely fractures with associated complete or incomplete (greenstick) fractures of the posterior element of the same or an adjacent level. Due to the transmission of the axial load, as the vertebral body absorbs the compressive forces, it transmits the load perpendicular to the axis of compressive force. If this is of significant magnitude, it will result in failure of the anterior and middle columns of the “burst” component of the vertebral body. The transmission of the compressive force through the pedicles leads to splay of the entire posterior arch of the vertebra involved. This has been reported as an increase in the interpedicular distance and vertical laminar fractures (2,4). Due to the intimate relationship with the spinal canal, it is common to see retropulsion of fractured segments into the spinal canal causing varying degrees of neural compromise. At the time of impact which is likely a fraction of a second, there will be a substantial increase in the intradural pressure localised to that level and related to direct force of the retropulsed fractured posterior vertebral cortical bone with high possibility of a ruptured dural sac.
Forceful retropulsion of fractured segment, as well as the triangular shape of the spinal canal (with the apex at the spinous process), causes transmission of forces directly onto the posterior lamina. The retropulsed bone is in the horizontal axis to the triangular-shaped spinal canal, impacting on the inner lateral aspect of the lamina causing laterally directed load on the inner lamina, which in association with the splay of the posterior arch of the vertebra through the pedicles, leads to a vertical fracture of the lamina. The degree of the separation amongst fractured segments is probably far greater at the time of impact than we see later on the computed tomography (CT) or magnetic resonance imaging (MRI) images. This may either result in splitting along the spinous process, but as this is the strongest point in the vertebral arch, what is more likely is transmission of force along the length of the lamina and fracture at its weakest point, which may be the facet joint below to one side, or most commonly across either lamina resulting in the classic green stick or complete laminar fracture. Entrapments of neural elements within this posterior lamina fracture are well described; and many attempts have been made to try to predict this occurrence via radiological methods as it will affect operative management (4). The combination of lamina fracture and clinical neurological deficit is of significant predictive value of a dural rupture, CSF leaks, and entrapment of the neural tissue in the fracture line. However, intra-operative observation is the only confirmatory method (5,6).
The purpose of surgical repair of this type of injury includes spinal canal decompression, release of the entrapped neural elements and instrumentation of the vertebral column to stabilise the fracture. Our group has accumulated experience in managing patients with burst fracture associated with vertical laminar fracture and entrapped neural elements. A special surgical technique of an open-book laminoplasty has been described.
Significance of the work
At present, there is no consensus regarding the management of burst lumbar vertebral body fractures with entrapped neural elements as it is a relatively infrequent occurrence for this type of fracture. However, being a tertiary care referral centre for this type of injury receiving referrals from a population of approximately 3 million, our institution was able to accrue the largest case series and implement a novel technique for the management of this infrequent complication. To our knowledge, the release of entrapped neural elements in burst lumbar vertebral body fractures, and reduction of bone fragments from the spinal canal to accelerate remodelling has not been described. We have demonstrated a safe and effective technique for this purpose.
Methods
Study design
This study involves data collected within routine clinical practice at our trauma unit. The UK National Health Service National Research Ethics Service guidance on such research (National Health Service Health Research Authority, 2011) has determined that such case series do not require ethical appraisal or clearance, as this is an evaluation of routine practice/National Health Service audit. The imaging (intraoperative and CT imaging) used was consented by the patients involved in the study.
We identified 17 patients older than 15 years of age, who were admitted to our department with burst vertebral body fractures of the L1 to L5 vertebral bodies with retropulsion of fractured segments into the spinal canal, and associated lamina fractures identified on CT and MRI scans (Figures 1,2). Patients were included in the study if they have intra-operative confirmation of a ruptured dural sac along with neural tissue entrapment. All the patients were followed up in outpatient clinics. There was no further neurological deterioration in any of the patient during the period of follow up, 3–12 months. All the patients underwent posterior decompression and stabilisation of the spinal column using pedicular screws and rods construct.
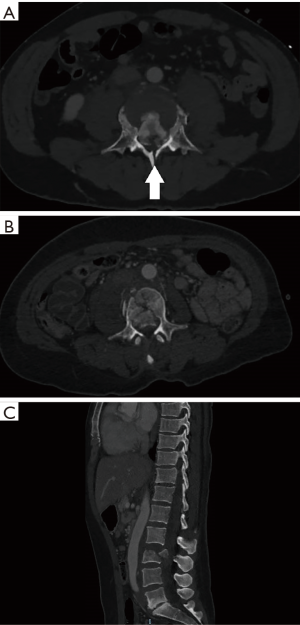
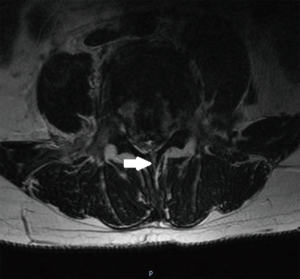
Patients who have unstable burst vertebral body fractures presenting to our department were evaluated and resuscitated according to our departmental trauma protocols. Once the appropriate candidate was deemed suitable for operative intervention, routine pre-operative checks were conducted in the standard fashion. The patient’s pre-operative neurology was rigorously charted using the Standard American Spinal Injury Association Impairment Scale (ASIA), and radiology reviewed by a multi-disciplinary team.
Operative technique
Intra-operatively, after identifying the fractured level using fluoroscopy, standard midline posterior approach was performed in all cases. Extra care was taken when stripping the muscles at the level of the fractured lamina. We avoided direct contact of monopolar diathermy close to the laminar fracture to avoid damage of the neural tissues. In 2 of the cases (n=2, 11.8%), nerve roots were identified within the paraspinal muscles, even before removal of any bone. We used fluoroscopy to confirm the entry points of the pedicle screws. The screws were inserted in the standard manner followed by rod fixation on one side (Figures 3,4). This is done to provide spinal stability. Once spinal stability was ensured, a laminectomy at the level of the retropulsed fracture segment was commenced, decompressing the spinal canal. Care should be taken that the laminectomy dimensions are wide enough, usually from the pedicle of the fractured vertebra to the pedicle of the vertebra below, laterally extending to the facet joint or beyond to provide enough space for the nerve roots to lag away from the retropulsed segment and to facilitate dural retraction and reduction of the retropulsed segment in a later stage of the surgery. The spinous process of the fractured vertebral body is left intact to give a pivot point during open book removal of the fractured lamina, especially when the fracture line is within the spinous process. The cranial and caudal supraspinous and inter-spinous ligamentous attachments were then removed. The laminectomy was done in a fashion to ensure that the level where the dorsal lamina is fractured was not immediately disturbed. Ligamentum flavum was preserved to aid in this function. At present, there is no good evidence for predictive tests for entrapped elements, and for safety, entrapment must be assumed until proven otherwise. The fractured lamina that may entrap neural elements was then carefully mobilised from surrounding bone away from the fractured side using a combination of appropriately sized Kerrison punches. The fractured lamina can also be disconnected on one side, lateral to fracture line, and an attempt to lift the lamina from fracture line as open book to avoid damage of the entrapped nerve roots. Extra care should be taken to free the nerve roots while doing this step. In our experience, keeping the spinous process intact for this purpose facilitates in stabilising the fractured lamina, and earlier removal of the spinous process may injure the entrapped neural elements. It may be necessary to disintegrate the lamina with the entrapped nerve roots to free them. The next step was to remove the bone of the lamina on the other side of the fracture away from the nerve roots until they became free. Care and precision should free the entrapped neural element and allow for removal of the remaining fractured lamina from the operative field. The resulting decompression should be adequate to allow visualisation of the torn dura and herniated nerve roots (Figures 3B,5). In one case, small bony fragments attached to neural tissue were difficult to remove and left behind within the dura. Dural repair was attempted if possible, although in most of our cases we left the dura open or we increased the dural opening intentionally to relieve the pressure from swollen nerve roots which were compressed, or strangulated from swelling. The next phase of the operation consisted of distraction of the unilateral posterior instrumentation, allowing some degree of reduction of the burst vertebral body fragments (Figures 3C,5). This cannot be done safely without the posterior decompression. Using distraction, height was restored under image intensifier guidance and comparing with the adjacent normal vertebral bodies. Once satisfactory anatomical positioning was achieved, the retropulsed segments of burst vertebral body were ready to be reduced.
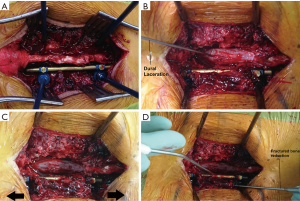
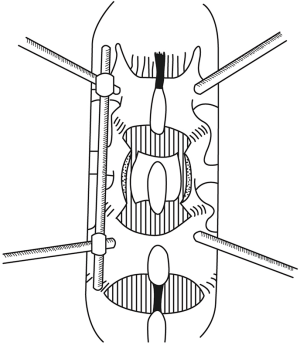
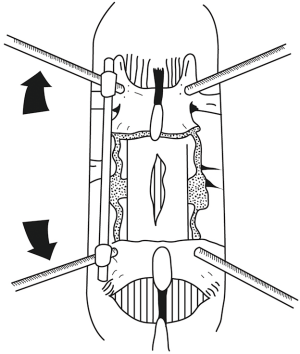
A significant amount of caution is required at this stage. A good understanding of the anatomical clearances lateral to the dura at each level, and an appreciation for the anatomical location of the posterior longitudinal ligament, and middle column is absolutely vital, as an error in judgment may result in significant morbidity.
A nerve root retractor instrument such as an O’Connell’s retractor, a McDonald’s retractor or an appropriately sized bone impactor was introduced lateral to the dura, ensuring the position of the exiting nerve roots at that level (Figures 3D,6,7). The retropulsed vertebral body fracture segment was identified, and the bone impactors positioned over it. The retropulsed segment was gently pushed back into the fractured vertebral body space. Use of a mallet is not encouraged due to the risk of slipping and injury to the cauda equina nerves. If there are multiple retropulsed segments, the reduction may have to be repeated from the other side. Smaller fragments may be removed altogether. Reduction may be confirmed by fluoroscopy.
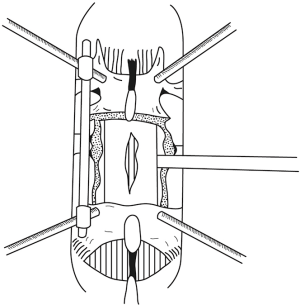
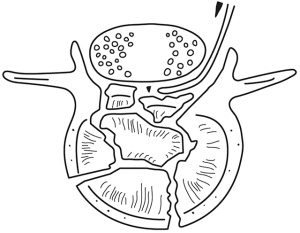
Once the vertebral body burst fracture segments were adequately reduced, rod fixation on the remaining side was achieved and bone graft placed after decorticating the facet joint and transverse process, lateral to facet joint and the fixation rods. The dural defect may then be repaired, and the wound closed. Post-operative CT scan were done in all patients to confirm good fracture reduction and implants position (Figure 8).
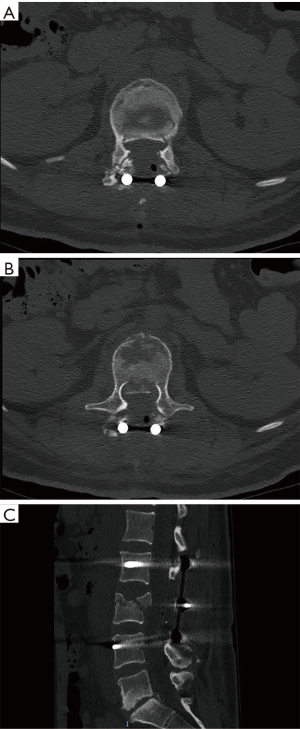
Results
From June 2015 to April 2017, we identified 17 patients who met the inclusion criteria. The age range was from 15 to 70 years with a median age of 43 years. On admission, seven patients had no neurological deficit, or minor deficits quantified as ASIA category E, two patients were ASIA A, two patients ASIA B, three patients ASIA C and three patients ASIA D. With the exception of 1 patient who fell down a flight of stairs, all the remaining 16 patients fell from a height of greater than 2 m. L1 to L5 lumbar vertebrae were fractured with the described pathology. The most common vertebra fractured was L4 in 7 patients, 5 of which were in combination with other vertebrae. The second most commonly fractured vertebrae was L2 in 6 patients. Five patients had two level fractures with three of them being L2/L4 fractures. The mean and median lengths of stay in hospital were 29.3 and 28 days respectively, with one patient ASIA B staying 79 days. This length of stay reflects the prolonged wait for a bed in the regional spinal rehabilitation centre rather than any surgical complications. Out of 17 patients, 13 had other organ injuries including head injury, long bone and sacral fractures, rib fractures, lung contusions, aortic dissection and sternal fractures. Eight patients had associated ankle fractures. Suicide attempts were identified as a cause of the falls in 10 of the patients.
Early post-operative neurological evaluation showed that 7 (41%) of the patients had at least one grade improvement on the ASIA scale. All the 7 (41%) patients with ASIA E didn’t develop any new neurological deterioration post-operatively. On the 3-month follow up assessment, 5 (30%) of the patients with neurological deficits pre-operatively improved to ASIA E. One patient remained ASIA A throughout the follow up which was related to his multiple thoracolumbar spinal fractures and conus medullaris injury confirmed with MRI scan (Table 1).
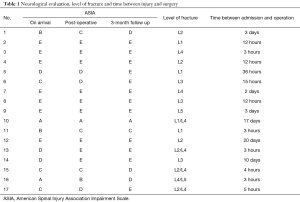
Full table
The time between admission and operation was within 24 hours for 10 (59%) patients, 72 hours for 4 (24%) patients and the remaining 3 (17%) patients were 10, 17 and 20 days respectively. This delay was related to patient’s other injuries and fitness for surgery.
Out of the 10 patients who underwent surgery within 24 hours, 6 had neurological deficits ranging from ASIA A to D. All of these 6 patients showed good neurological recovery especially with one patient improving from ASIA A to D and another patient from ASIA C to E.
Discussion
Posterior dural tears and herniation of the cauda equina nerves associated with thoracolumbar burst fractures were originally described by Miller et al. in 1980 (7). Denis and Burkus (8) reported that splaying of the posterior arch of the vertebra under axial load caused vertical lamina fractures, either complete fracture of the lamina or the splitting of the inner aspect of the lamina only. This was termed greenstick fractures of the anterior cortex of the lamina. At the time of impaction, the retropulsed pieces of the fractured vertebral body would hit the triangular shape, inner aspects of the posterior arch, through the dural sac, which forces the neural tissue to the fracture line during maximum separation (8-10).
There is a high rate of neurological injuries resulting from thoracolumbar burst fractures. Improved prediction rate of such injuries and appropriate medical and surgical treatment may reduce the neurological damage and improve the overall outcome. Significant neurological deficits associated with a radiological finding of burst fractures of vertebral body and green stick laminal fractures, should raise suspicion of burst dura and entrapment of neural tissue at the laminar fracture area (7,11-13). This necessitates an immediate initiation of a management plan including surgical decompression to avoid further permanent neural tissue damage. Surgical treatment of the lumbar spine burst fractures has been controversial and depending on the type of lamina fractures (whether of complete or incomplete greenstick type) as there is a high risk of dural tears and nerve root entrapment with complete lamina fractures (10,14).
In such patients, the goals of the operative treatment are the restoration of the anatomy of the spinal column, including the spinal canal, and to release entrapped neural structures. The decompression of compressing bone fragments over the spinal canal provides a better environment for restoration of neurologic function of the entrapped neural tissues.
Although there are suggestions that there is no difference between operative and non-operative treatment regarding neurological recovery and long term functional outcomes (15), studies in animal models demonstrate that neurological recovery is enhanced by early decompression (16). In a meta-analysis by La Rosa et al., the surgical outcome of early decompression (<24 h) was statistically better compared to both delayed decompression (>24 h) and conservative treatment especially in patients experiencing progressive neurological deterioration or incomplete paraplegia (16,17).
All cases with such injuries admitted to our major trauma centre have been treated operatively in the form of a dorsal midline approach, decompression of the cauda equina nerves, reduction of the fractured vertebrae and stabilization with transpedicular internal fixators. The time from injury to the operation was variable, depending on other injuries requiring urgent attention; however, we aim to do the surgery as soon as feasible. The mean and median times from admission to surgery were 3.6 days and 16.8 hours respectively with over half of patients having their surgery within 24 hours of the injury.
Entrapped neural elements can be successfully extracted from the lamina fracture by an open book laminectomy of the posterior neural arch. Patients with lumbar burst fractures and radiographic evidence of posterior arch fracture, are highly likely to have entrapment of the neural elements in the lamina fracture, and should undergo posterior exploration of the spinal canal, extraction of cauda equina neural elements, and repair of the dural laceration before any spinal reduction manoeuvre (8). Under axial loading (fall from a height) the pedicles and the posterior elements splay laterally and the bone is retropulsed from the vertebral body, which can cause the dura to protrude between the lamina fracture fragments. After dissipation of axial loading, the nerve roots and the dura are entrapped.
There is a correlation between the level of the spinal fracture and the probability of neurological deficit. The more cranial the fracture the higher the probability of neurological deficit related to the upper cauda equina nerves and the level of the conus medullaris (18).
Most of the cases reported in the literature were treated either conservatively allowing the bone to heal by remodelling, or surgically using a dorsal approach, by postural reduction, instrumental angular reduction and stabilization with a long segment transpedicular internal fixator (19,20). In our practice, we have used a novel surgical technique: short unilateral fixation, decompression, reduction and complete the fixation to reduce damage of the neural tissue and perform a vital role in restoration the vertebral column anatomy.
From our neurological status evaluation, there is significant recovery in patients presenting with neurological deficits, which indicates that a combined entrapment of the neural tissue and compression by displaced fractured vertebra are the main reasons behind this deficit. However, it is difficult to confirm which one is the main contributor, as both were corrected at the same time. As reported in the literature, it is also difficult to identify which category has dural rupture and neural tissue entrapment in the pre-operative imaging. However, these are highly suspicious in cases with neurological deficit and such entity of fracture.
Conclusions
Our institution has compiled a case series of a relatively infrequent type of traumatic burst lumbar vertebral body fracture; one with dural rupture and entrapped neural elements. Using our technique of a posterior instrumentation, spinal canal decompression, release of entrapped nerve roots and reduction of the retropulsed segment, we have shown that this is a safe and effective method of treating this type of fracture. The 17 patients of varying ages in our study demonstrated no deterioration in post-operative neurology, and our technique allows for earlier mobilisation than would be possible with conservative treatment. Caution needs to be exercised with generalising this type of treatment as there is no consensus on standard of treatment for this type of injury, and the procedure requires a degree of expertise with treating spine pathology. Further study is needed to compare other methods of treatment and long-term outcomes.
Acknowledgements
None.
Footnote
Conflicts of Interest: The authors have no conflicts of interest to declare.
Ethical Statement: The UK National Health Service National Research Ethics Service guidance on such research (National Health Service Health Research Authority, 2011) has determined that such case series do not require ethical appraisal or clearance, as this is an evaluation of routine practice/National Health Service audit. The imaging (intraoperative and CT imaging) used was consented by the patients involved in the study.
References
- White AA, Panjabi MM. Clinical Biomechanics of the Spine. 2nd Edition. Baltimore: Lippincott Williams & Wilkins, 1990.
- Denis F. The three column spine and its significance in the classification of acute thoracolumbar spinal injuries. Spine (Phila Pa 1976) 1983;8:817-31. [Crossref] [PubMed]
- Heary RF, Kumar S. Decision-making in burst fractures of the thoracolumbar and lumbar spine. Indian J Orthop 2007;41:268-76. [Crossref] [PubMed]
- Lee IS, Kim HJ, Lee JS, et al. Dural tears in spinal burst fractures: predictable MR imaging findings. AJNR Am J Neuroradiol 2009;30:142-6. [Crossref] [PubMed]
- Pau A, Silvestro C, Carta F. Can the lacerations of the thoracolumbar dura be predicted on the basis of radiological patterns of the spinal fractures? Acta Neurochir (Wien) 1994;129:186-7. [Crossref] [PubMed]
- Silvestro C, Francaviglia N, Bragazzi R, et al. On the predictive value of radiological signs for the presence of dural lacerations related to fractures of the lower thoracic or lumbar spine. J Spinal Disord 1991;4:49-53. [PubMed]
- Miller CA, Dewey RC, Hunt WE. Impaction fracture of the lumbar vertebrae with dural tear. J Neurosurg 1980;53:765-71. [Crossref] [PubMed]
- Denis F, Burkus JK. Diagnosis and treatment of cauda equina entrapment in the vertical lamina fracture of lumbar burst fractures. Spine (Phila Pa 1976) 1991;16:S433-9. [Crossref] [PubMed]
- Aydinli U, Karaeminogullari O, Tiskaya K, et al. Dural tears in lumbar burst fractures with greenstick lamina fractures. Spine (Phila Pa 1976) 2001;26:E410-5. [Crossref] [PubMed]
- Skiak E, Karakasli A, Harb A, et al. The effect of laminae lesion on thoraco-lumbar fracture reduction. Orthop Traumatol Surg Res 2015;101:489-94. [Crossref]
- Pickett J, Blumenkopf B. Dural lacerations and thoracolumbar fractures. J Spinal Disord 1989;2:99-103. [Crossref] [PubMed]
- Cammisa FP Jr, Eismont FJ, Green BA. Dural laceration occurring with burst fractures and associated laminar fractures. J Bone Joint Surg Am 1989;71:1044-52. [Crossref] [PubMed]
- Kahamba JF, Rath SA, Antoniadis G, et al. Laminar and arch fractures with dural tear and nerve root entrapment in patients operated upon for thoracic and lumbar spine injuries. Acta Neurochir (Wien) 1998;140:114-9. [Crossref] [PubMed]
- Limb D, Shaw DL, Dickson RA. Neurological injury in thoracolumbar burst fractures. J Bone Joint Surg Br 1995;77:774-7. [Crossref] [PubMed]
- Vaccaro AR, Kim DH, Brodke DS, et al. Diagnosis and management of thoracolumbar spine fractures. Instr Course Lect 2004;53:359-73. [PubMed]
- Fehlings MG, Perrin RG. The role and timing of early decompression for cervical spinal cord injury: update with a review of recent clinical evidence. Injury 2005;36 Suppl 2:B13-26. [Crossref] [PubMed]
- La Rosa G, Conti A, Cardali S, et al. Does early decompression improve neurological outcome of spinal cord injured patients? Appraisal of the literature using a meta-analytical approach. Spinal Cord 2004;42:503-12. [Crossref] [PubMed]
- Fontijne WP, de Klerk LW, Braakman R, et al. CT scan prediction of neurological deficit in thoracolumbar burst fractures. J Bone Joint Surg Br 1992;74:683-5. [Crossref] [PubMed]
- Tropiano P, Huang RC, Louis CA, et al. Functional and radiographic outcome of thoracolumbar and lumbar burst fractures managed by closed orthopaedic reduction and casting. Spine (Phila Pa 1976) 2003;28:2459-65. [Crossref] [PubMed]
- Weinstein JN, Collalto P, Lehmann TR. Thoracolumbar "burst" fractures treated conservatively: a long-term follow-up. Spine (Phila Pa 1976) 1988;13:33-8. [Crossref] [PubMed]