What should my hospital buy next?—Guidelines for the acquisition and application of imaging, navigation, and robotics for spine surgery
Introduction
The range of imaging, navigation, and robotics technologies available for spinal fusion surgery has significantly increased. Contemporary assistive technology options demonstrate a high rate of accuracy for pedicle screw placement. Surgeons and hospital administrators do not have sufficient information to compare imaging, navigation, and robotics technologies when making purchasing decisions. Traditional open spine surgery using anatomical landmarks results in rates of free-hand pedicle screw misplacement as high as 40% according to post-operative CT imaging (1). Accuracy of screw placement improves to 86.6–94.9% with 2D fluoroscopy (2,3).
The evolution of minimally invasive spine (MIS) approaches introduced 2D fluoroscopy using K wires and EMG neuromonitoring to insert percutaneous pedicle screws and have higher accuracy rates of 90.2–97.5% (2,4). However, these approaches require surgical teams to wear lead protection. Three-dimensional spinal navigation with intraoperative 3D fluoroscopy and bone-anchored (spinous process or iliac crest) tracking provides comparable accuracy without requiring lead protection (5). This approach also minimises radiation exposure by the surgical team and patient (6,7).
Renaissance (Mazor Robotics, Caesarea, Israel) was the first widely used spinal robotic platform. Combined with bone anchoring, Renaissance provides pedicle cannulation for K wires and yields high accuracy for screw placement with reduced exposure to radiation (8,9). Use of a non-invasive, rectangular skin-adhesive stereotactic tracker (SpineMask, Stryker Navigation, Kalamazoo, MI, USA) maintains high accuracy for percutaneous screw placement without requiring bone-anchored tracking or the morbidity of K wires (10,11). Contemporary technologies, including intraoperative CT and new 3D fluoroscopy, offer improved image quality but significantly increase radiation exposure (12,13). New robotic platforms offer more versatility with integrated navigation and can be used without K wires. Clinical evaluation to justify the increased radiation of 3D fluoroscopy is ongoing. Similarly, new robotic technologies for spine surgery require evidence to justify higher costs.
We aim to: (I) present a synopsis of imaging, navigation, and robotics technologies currently available for spine surgery in Australia and New Zealand; and (II) make recommendations for applications of these technologies based on the authors’ experiences, publicly available information, and peer-reviewed data. Distinguishing features and utility of each technology are discussed and include information, such as price and compatibility, which are rarely included in peer-reviewed literature.
Imaging
Use of intraoperative CT has increased in Australia because of improved image quality and a larger field of view (FOV) compared with those obtained with first-generation 3D fluoroscopy scanners. Increasing compatibility with navigation and robotics platforms expands their utility outside of diagnostic imaging. Compared with 3D fluoroscopy, intraoperative CT scanners are larger. Although they are technically mobile, specialist or integrated tables are required, restricting their use due to logistic and scheduling challenges. By contrast, 3D-fluoroscopy units are readily mobile between theatres.
Intraoperative CT demonstrates the greatest benefit in the following contexts: soft-tissue imaging for tumour resection; a wide FOV for pelvic imaging; a long scan length for deformity correction with multilevel construct; image quality in tissue-dense areas, especially the cervicothoracic junction; and in large patients, who require higher doses of radiation to penetrate tissue (13).
Second-generation 3D fluoroscopy scanners, Cios Spine (Siemens AG, Erlangen, Germany), RFD 3D (Ziehm, Nuremberg, Germany), and the O-arm II (Medtronic Navigation, Louisville, CO), produce significantly improved image quality and wider FOVs compared with first-generation 3D scanners Orbic (Siemens), Vario 3D (Ziehm), and O-arm (Medtronic). Newer 3D scanners deliver radiation doses equivalent to or greater than CT and are typically cheaper, lighter, smaller, and more manoeuvrable. Three-dimensional scanners are advantageous if speed is required over image quality and add to theatre workflow efficiency due to their utility as 2D-fluoroscopy units.
Siemens, Ziehm, and Samsung Neurologica do not produce navigation or robotics platforms for spine surgery, but their scanners are often compatible with imaging and navigation systems. By contrast, the Airo and O-arms are optimised for integration with BrainLab and Stealth navigation and robotics platforms, respectively.
Siemens Orbic 3D, which was launched in 2007, is easily mobile with a 12-cm FOV and costs A$300,000–$380,000. An updated version of the Orbic, called Siemens Cios Spin, has a larger bore. The wider bore can help with clearance around larger patients but shrinks the FOV to 16 cm for 3D scans, even though the scanner has 30-cm detectors. Because it is relatively new, Siemens Cios currently integrates with BrainLab navigation; other options are likely to become available in the future. It costs A$400,000–$500,000.
The Ziehm RFD 3D CMOS is also highly mobile, resembling standard 2D-fluoroscopy units in size, and has a larger 20-cm FOV on a 3D scan. The Ziehm RFD generates the same power as an O-arm II with a smaller pixel size, giving the two scanners similar image quality. Among the new 3D-fluoroscopy units, the Ziehm offers the highest compatibility and integrates with most navigation and robotics platforms. The Ziehm RFD has good image quality, a large FOV, 2D functionality, wide-ranging compatibility, and is a cost-effective option at A$430,000–$450,000.
The Medtronic O-arm II introduced image stacking of two scans completed side by side, allowing for longer segments of the spine to be imaged efficiently. Thus, the O-arm II has the longest scan length of the 3D-fluoroscopy units but cannot be used easily for 2D fluoroscopy. The O-arm II is the largest and heaviest of the 3D-fluoroscopy units with CT-like radiation doses (2–2.5 mSv) (14). The O-arm II requires an assisted-drive mechanism similar to mobile CT scanners with optional manual motion. The O-arm integrates well with Stealth Navigation and costs A$850,000. Figures of the different 3D-fluoroscopy imaging systems are provided in Figure 1.
Image quality and radiation doses of 3D-fluoroscopy units are similar to CT scanners due to increased power and FOV. CT scanners remain the only option for diagnostic soft-tissue imaging. Two intraoperative CT scanners are available.
Mobius Airo (Mobius Imaging, Shirley, MA, USA) is an open-platform imaging CT with a 100-cm scan length and a 56-cm FOV. The wide, 107-cm bore accommodates large patients. Airo has an assisted drive for movement and an integrated table, which reduces setup time but keeps the scanner close to the operative area. Until recently, BrainLab distributed Airo exclusively. Airo integrates well with BrainLab navigation and has basic CT diagnostic and viewing capabilities. Airo costs A$600,000–$1,200,000.
BodyTom (Neurologica, Danvers, MA, USA) is another open-platform imaging CT with assisted drive. In contrast to Airo, BodyTom requires a cantilevered carbon fibre table, which is detached from the scanner. Thus, the scanner can be transported from the operative area when not in use. BodyTom is a popular diagnostic CT scanner, because it has smaller detectors, a more powerful generator, less noise, and software to reduce metal artefacts. However, these advantages appear to have little impact on intraoperative outcomes. BodyTom has the largest scan length (200 cm) and a comparable FOV (60 cm), but its 80-cm bore is narrower. BodyTom is also the largest and heaviest imaging unit with a live video monitor to assist movement. It costs A$700,000-$1,100,000. Figures of the different intraoperative CT imaging systems are provided in Figure 2.
Radiation
Radiation safety, including dose and exposure of staff and patients in the operating theatre, is receiving increased international attention (15,16). Although radiation emissions data are available for different imaging units, these data are rarely collected or presented in a standardised and easily understood fashion for spine surgeons.
Methods for assessing radiation exposure are challenging. The most common measurement of radiation output is provided in Gray (Gy) for the absorbed dose. Dose area product (DAP) is defined as the absorbed dose multiplied by the area irradiated expressed in mGy.cm2. DAP is easily measured and is a metric of radiation risk. Effective dose is the preferred measurement but is more difficult to measure. Effective dose is based on absorption by a specific tissue or organ in a patient and is measured in Sievert (Sv). One imaging study may compare radiation time per screw, but another may compare total radiation dose generated at the source for an entire operation using a different scale or radiation measurement. Data interpretation and comparison of clinical applications of imaging units are challenging for non-specialist radiology staff due to the lack of non-standardised reporting.
Use of different techniques significantly alters radiation exposure during surgery. For example, multiple spins with a 3D-fluoroscopy unit may increase overall radiation doses to staff and patient compared with a single intraoperative spin and co-registration with a pre-operative CT. Further, the radiation of a particular system is difficult to define due to large variations in applications of intraoperative imaging. Consider a robotics case, in which a surgeon uses pre-operative CT for planning and scans the patient with a 3D-fluoroscopy unit to confirm intraoperative screw placement. Pre-operative CT dose varies according to the preference of the radiologist, which a patient selects based on proximity and convenience. Exposure settings of the intraoperative fluoroscopy unit depend on multiple factors, including the experience and level of the radiographer, patient body habitus, and requirements set by the surgeon, who may prefer the highest image quality for a difficult operation.
Spine surgeons should understand that new 3D-fluoroscopy and intraoperative CT scanners deliver similar levels of radiation to patients and staff. The greatest determinant of overall radiation dose delivered to a patient is the number of scans. Therefore, surgeons should combine imaging, navigation, and robotics platforms to reduce the number of pre and post-operative scans required for a spine fusion. Operators use high power settings on 3D-fluoroscopy and intraoperative CT scanners to obtain clinically usable intraoperative images that clearly delineate cortical bone around pedicle walls. Although marketing information includes low-dose options for scanners, these settings are rarely utilised and should not be used to compare systems.
Our institution consistently uses higher dose settings. Therefore, scan length is likely to be the main determinant of radiation dose to a patient. Compared with a 10-cm intraoperative CT scan length, a 20-cm scan doubles the radiation dose delivered to a patient. Similarly, a doubling effect occurs for an O-arm II if the scan length is increased from 20 to 40 cm using image stacking. Only intraoperative CT scanners have a shorter minimum scan length ≤4 cm. Intraoperative CT with a short segment fixation that does not require a 16 to 20 cm FOV reduces radiation by imaging only target areas. Spine surgeons should consider balancing setup time with image quality rather than variations in radiation doses when comparing 3D-fluoroscopy versus intraoperative CT units.
Proximity to the radiation source (1/distance2) and use of protective lead gowns have the biggest impact on radiation exposure of staff. We advocate for an ergonomic and comfortable work environment, in which surgical teams leave the theatre during scanning and are adequately protected from radiation without requiring lead glasses and gowns. Other authors report similar systems for reducing radiation exposure of staff (13). Systems that rely on pre-operative CT scanning reduce intraoperative radiation exposure of staff.
Navigation
Major navigation suppliers offer a similar range of navigated instrumentation for cervical and thoraco-lumbar spinal fixation (open and MIS). Software features, such as screw planning and co-registration of pre-operative CT with intraoperative 3D imaging, are now standard. In addition to instruments from navigation companies, navigation-compatible instrumentation are produced by spinal implant manufacturers to increase options for spinal fusion equipment that easily integrate with a navigation platform.
Medtronic’s O-arm II was recently updated with the ability to stack two scans to create a 40-cm scan length to help with longer spinal fusions. Launch of Airo intraoperative CT by BrainLab provides surgeons with a well-integrated alternative to the O-arm. The new navigation technology, 7D Surgical, uses machine vision to co-register exposed bone with existing imaging. This novel registration method saves time by eliminating the need for intraoperative imaging and permitting rapid re-registration if a navigation tracker is moved. Early reports indicate that this technology reduces time but preserves high levels of accuracy compared with existing navigation technology.
The universal registration mechanisms Stryker SpineMask and BrainLab Universal Air permit on-table registration and are compatible with contemporary 3D and CT scanners. These mechanisms eliminate the need to calibrate between navigation and imaging systems. Calibration of navigation and robotics platforms with imaging systems is a slow process that limits integration between platforms and often requires collaboration between commercial competitors. Updating technologies to be compatible with rapidly evolving imaging approaches provides a cost benefit. In addition, risk of inaccuracy and requirements for technical expertise may be reduced by eliminating the need for calibration between systems. Stealth, BrainLab, Stryker, and 7D Surgical are the currently available navigation systems.
Medtronic Stealth
The Stealth system from Medtronic (Minneapolis, MN, USA) is the most common navigation platform used for the spine. Stealth is compatible with 3D fluoroscopy and typically used with O-arm intraoperative imaging. This open-navigation platform was the first to offer integrated screw placement instruments. O-arm was recently updated [2017] to permit the O-arm II to stack two scans to create a 40-cm scan length. In 2017, Medtronic and the FDA issued a warning about using non-Medtronic instrumentation with Stealth and suggested using only Medtronic instrumentation with Stealth.
BrainLab
BrainLab (Munich, Germany) is a navigation platform that is not linked with an implant company. BrainLab offers integration with the widest range of imaging platforms and is compatible with 2D and 3D fluoroscopy. Surface-matching registration permits the use of pre-operative CT scans. BrainLab has automatic image registration with Airo intraoperative CT imaging. Region matching is similar to traditional point-based fiducial type registration but allows surgeons to identify larger regions rather than specific points. These larger regions are then refined using surface matching.
Stryker
In contrast to all other navigation and robotics platforms that use reflective spheres, Stryker Navigation (Kalamazoo, MI, USA) uses battery-powered instruments to generate infrared light for instrument tracking. This highly accurate tracking mechanism produces instruments with a different design and feel than other navigation systems. Stryker was the first to release a universal registration mechanism, which works with most imaging systems. Stryker includes a patient tracker called SpineMask, which consists of an adhesive cutaneous frame that does not require bone anchoring. We reported on the first use of a navigated K-wireless screw insertion technique using this system (10).
7D Surgical
7D Surgical (Toronto, Canada) uses machine vision, which is similar to technology found in self-driving cars. Machine vision is used to co-register bony anatomy with pre-existing imaging without the use of radiation. Registration requires direct visualisation of bone and captures up to >250,000 data points over a 40 cm × 30 cm area to a resolution of 4–6 points/mm2. The registration process is rapid and can be easily repeated if the patient tracker is displaced. Rapid registration allows the patient tracker to be moved during each registration, maintaining proximity to the operative area using a standard, non-radiolucent operating table. Early reports demonstrate significant improvements in workflow and registration time compared with those of contemporary navigation systems (41 vs. 258–794 seconds) without compromising accuracy (17).
Figures of the different spinal navigation systems are provided in Figure 3.
Robotics
The major difference between navigation and robotic platforms is that robotics requires pre-planning for screw trajectories and pre-operative CT. Proponents of pre-operative screw planning believe that robotics can predict anatomical challenges and save operative time. However, others challenge the need for this additional step.
Updated registration processes for robotics permit registration with intraoperative 3D and CT imaging using a workflow that mirrors traditional navigation systems that are often more familiar. Pre-operative CT planning prevents the removal of large amounts of bone prior to registration and image correlation. Thus, interbody and decompression work cannot be completed before registration and application of a robotic platform. On-table registration provides more flexibility with the operative workflow, allowing surgeons to complete interbody cage insertion without concerns about registration inaccuracy.
The Mazor Renaissance is the only widely used robotic spine platform in Australia. The Renaissance is a mechanical guidance unit fastened to the patient with bone-anchored attachments, which can be used to cannulate pedicles and place K wires.
Two new robotic arm platforms have launched in Australia and New Zealand: the MazorX (Medtronic) and Excelsius (Globus Medical, Audubon, PA, USA). These platforms have mechanical arms with a floor or table mount. Both offer optical instrument tracking navigation units, assist with K-wire placement, and provide guidance for instrumentation. The mechanical arms require surgeons to introduce tools through ports and provide haptic feedback if instruments deviate from the pre-planned trajectory. Compared to the Mazor Renaissance, the active robotic arms take longer to setup. However, they can be moved to pre-planned screw trajectories more rapidly during instrumentation.
Two robotic systems are in development. The ROSA robot from Zimmer Biomet (Warsaw, IN, USA) was originally developed for cranial applications. Similar to the Stryker MAKO robot, ROSA is currently being adapted to increase its utility in spinal surgery. These next-generation robots are expected not only to drill but also to remove bone. This increased function will expand their applications in spinal procedures and differentiate them from contemporary navigation systems.
Pre-planning features of robotic software platforms facilitates rod placement through optimised screw head alignment. Future software versions are likely to have increased functions for planning spinal correction and may facilitate custom spinal prostheses, such as patient-specific contoured rods. The robotic systems currently available are Mazor Renaissance, MazorX, ExcelsiusGPS, and Cirq.
Mazor Renaissance
The Mazor Renaissance launched in 2011 and is the most established robotic guidance unit for the spine. More than 30,000 cases have been completed worldwide. The Mazor can be mounted to bony anatomy and/or the operating table. This open-platform unit places K wires only. The majority of cases utilise pre-operative planning. The Mazor unit costs A$600,000–$900,000 with disposable costs of A$1,000 per case.
MazorX
Although the MazorX launched in the US in 2016 as a stand-alone robotic platform, it will launch in Australia integrated with Stealth and optical instrument tracking. The MazorX is table-mounted and can be used as an open platform for K-wire placement. This system has navigation compatibility with Medtronic prostheses and costs A$1,000,000–$1,500,000.
Excelsius GPS
The Excelsius GPS from Globus Medical launched in 2018 and uses robotic arm technology with fully integrated navigation. Excelsius GPS provides K-wireless, real-time screw placement in all spinal segments with a free-hand image guidance option. It is compatible with most imaging systems and allows for pre-operative CT, intraoperative CT, and 2D-fluoroscopic workflows. Excelsius GPS is open platform but optimised for Globus prostheses and costs A$1,500,000.
BrainLab Cirq
Cirq is a recently released passive robotic arm. Cirq has a port that can be locked into place and used as a placeholder to rapidly identify previously established trajectories. Future iterations are likely to permit moving to predefined trajectories. Cirq is not a stand-alone device and utilises existing BrainLab navigation. Cirq costs A$100,000–$250,000.
Figures of the different robotic platforms are provided in Figure 4.
Guidelines
Guidelines for the purchase of new assistive technologies for spinal fusion are provided in Table 1.
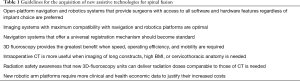
Full table
Application of technologies
Growth of imaging, navigation, and robotics technologies provide surgeons with an increasing number of registration options. Registration is the process by which a navigation or robotics platform determines the location of a patient in space and may include co-registration with pre-operative or intraoperative imaging. The effectiveness of a registration method depends on many factors, such as speed, accuracy, reproducibility, convenience, approach, operative location, patient body habitus, anatomy, and radiation exposure. Surgical teams prioritise workflow efficiency over image quality. Recommendations are provided for registration using navigation (Table 2) and robotics (Table 3) based on the above factors.
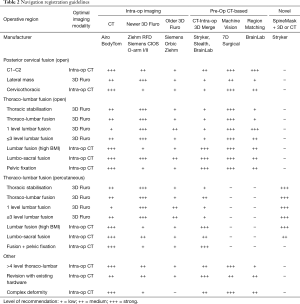
Full table
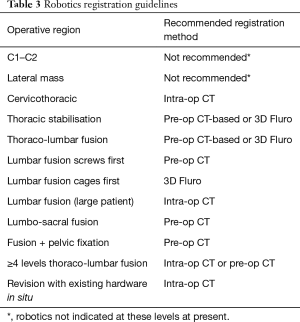
Full table
Conclusions
Assistive technologies and prostheses for spinal fusion are evolving rapidly. Open-platform navigation and robotics systems that provide surgeons access to all features of the assistive technology regardless of the spinal prosthesis being used are preferred. Navigation and robotics platforms that offer universal registration should be favoured for ongoing utility as hospitals acquire new imaging technology. Imaging systems that offer a wide range of integration with navigation and robotics platforms should be favoured until all systems offer universal registration mechanisms. Intraoperative 3D fluoroscopy is recommended for standard thoracic and lumbar fusions, as it provides good value for money, high mobility, and efficient registration. However, 3D fluoroscopy delivers radiation comparable to that of intraoperative CT, which should be balanced against favourable intraoperative efficiency. Intraoperative CT is better if high accuracy is of paramount importance, or if high tissue density reduces image quality. It is recommended for posterior cervical, thoracic, and long-segment fusions and for patients with high BMI. Intraoperative CT scanners are harder to use across multiple operating rooms. New robotic arm technologies with integrated navigation are costly and require additional data to justify the expense.
Acknowledgements
The authors wish to thank the imaging, navigation, and robotics suppliers, all of whom provided information and permission to use the images in this article.
Footnote
Conflicts of Interest: GM Malham—Globus (consultancy), NuVasive (consultancy), Stryker (consultancy), Lifehealthcare (travel). T Wells-Quinn—LifeHealthcare Employee, Distributor in ANZ of: Mazor Renaissance, BodyTom, 7D Surgical.
References
- Castro WH, Halm H, Jerosch J, et al. Accuracy of pedicle screw placement in lumbar vertebrae. Spine (Phila Pa 1976) 1996;21:1320-4. [Crossref] [PubMed]
- Malham GM, Goss B, Blecher C. Percutaneous Pedicle Screw Accuracy with Dynamic Electromyography: The Early Experience of a Traditionally Open Spine Surgeon. J Neurol Surg A Cent Eur Neurosurg 2015;76:303-8. [Crossref] [PubMed]
- Oh HS, Kim JS, Lee SH, et al. Comparison between the accuracy of percutaneous and open pedicle screw fixations in lumbosacral fusion. Spine J 2013;13:1751-7. [Crossref] [PubMed]
- Soriano-Sanchez JA, Ortega-Porcayo LA, Gutierrez-Partida CF, et al. Fluoroscopy-guided pedicle screw accuracy with a mini-open approach: a tomographic evaluation of 470 screws in 125 patients. Int J Spine Surg 2015;9:54. [Crossref] [PubMed]
- Wood MJ, McMillen J. The surgical learning curve and accuracy of minimally invasive lumbar pedicle screw placement using CT based computer-assisted navigation plus continuous electromyography monitoring - a retrospective review of 627 screws in 150 patients. Int J Spine Surg 2014.8. [PubMed]
- Du JP, Fan Y, Wu QN, et al. Accuracy of Pedicle Screw Insertion Among 3 Image-Guided Navigation Systems: Systematic Review and Meta-Analysis. World Neurosurg 2018;109:24-30. [Crossref] [PubMed]
- Mason A, Paulsen R, Babuska JM, et al. The accuracy of pedicle screw placement using intraoperative image guidance systems. J Neurosurg Spine 2014;20:196-203. [Crossref] [PubMed]
- Barzilay Y, Liebergall M, Fridlander A, et al. Miniature robotic guidance for spine surgery--introduction of a novel system and analysis of challenges encountered during the clinical development phase at two spine centres. Int J Med Robot 2006;2:146-53. [Crossref] [PubMed]
- Fan Y, Du JP, Liu JJ, et al. Accuracy of pedicle screw placement comparing robot-assisted technology and the free-hand with fluoroscopy-guided method in spine surgery: An updated meta-analysis. Medicine (Baltimore) 2018;97:e10970. [Crossref] [PubMed]
- Malham GM, Parker RM. Early experience of placing image-guided minimally invasive pedicle screws without K-wires or bone-anchored trackers. J Neurosurg Spine 2018;28:357-63. [Crossref] [PubMed]
- Mobbs RJ, Raley DA. Complications with K-wire insertion for percutaneous pedicle screws. J Spinal Disord Tech 2014;27:390-4. [Crossref] [PubMed]
- Hecht N, Yassin H, Czabanka M, et al. Intraoperative Computed Tomography Versus 3D C-Arm Imaging for Navigated Spinal Instrumentation. Spine (Phila Pa 1976) 2018;43:370-7. [Crossref] [PubMed]
- Navarro-Ramirez R, Lang G, Lian X, et al. Total Navigation in Spine Surgery; A Concise Guide to Eliminate Fluoroscopy Using a Portable Intraoperative Computed Tomography 3-Dimensional Navigation System. World Neurosurg 2017;100:325-35. [Crossref] [PubMed]
- Medtronic. Dose considerations O-Arm surgical imaging system. 2018. Available online: https://www.medtronic.com/us-en/healthcare-professionals/products/neurological/surgical-imaging-systems/o-arm/dose-considerations.html. 2019.
- Kim CW, Lee YP, Taylor W, et al. Use of navigation-assisted fluoroscopy to decrease radiation exposure during minimally invasive spine surgery. Spine J 2008;8:584-90. [Crossref] [PubMed]
- Villard J, Ryang YM, Demetriades AK, et al. Radiation exposure to the surgeon and the patient during posterior lumbar spinal instrumentation: a prospective randomized comparison of navigated versus non-navigated freehand techniques. Spine (Phila Pa 1976) 2014;39:1004-9. [Crossref] [PubMed]
- Jakubovic R, Guha D, Gupta S, et al. High Speed, High Density Intraoperative 3D Optical Topographical Imaging with Efficient Registration to MRI and CT for Craniospinal Surgical Navigation. Sci Rep 2018;8:14894. [Crossref] [PubMed]