The future of free vascularized fibular grafts in oncologic spinal and pelvic reconstruction
Introduction
The free vascularized fibular graft (FVFG) was first described in 1975 as a solution for large bone defects in orthopedic trauma (1). Since its appearance, the FVFG emerged as the first-line option for a number of reconstructions, including intercalary defects of the upper and lower extremities, craniofacial defects, as well as pelvis and spine applications. This popularity can be attributed to the fibula’s favorable anatomy, which allows it to be tailored to suit a variety of defects: it is long and straight, providing up to 26 cm of bone with a dependable dual endosteal and periosteal blood supply (2). This robust vascularity supports multiple osteotomies, enabling the bone to be used as a straight or shaped construct. The FVFG has more recently been used in the reconstruction of oncologic spine and pelvic defects. Given the relatively early experience using FVFG for the reconstruction of spinopelvic defects, the utility of the FVFG for this indication has not been clearly defined. Houdek et al. chronicle 15 years of experience with FVFGs for spine and pelvic reconstruction at the Mayo Clinic (3). In doing so, the authors nicely highlight the rarity and complexity of these cases; herein, we examine the role of the FVFG in the future of spinal and pelvic oncologic reconstruction.
Background
The goals of oncologic spine and pelvic surgery are to treat the patient’s cancer appropriately and maximize their chances to achieve the highest possible level of postoperative function. Houdek and colleagues appropriately asked specific questions regarding the survival and outcomes in patients with spine and pelvic tumors, specifically looking at bony union, complications, rates of revision, and functional outcomes. Isolated pelvic tumors have been studied by others, and it is now well established that the internal hemipelvectomy leads to higher functional outcome scores and lower complications rates when compared to the hindquarter amputation without compromising survival (4-6). Functional outcomes after total sacrectomy and other spinopelvic dissociating resections are not well defined in the literature.
Originally, the internal hemipelvectomy was without reconstruction with the allowance for the hip to develop a pseudarthrosis “flail hip” (4). A myriad of methods were described to reconstitute the pelvic ring in the 1980s and 90s, including cement constructs, megaprostheses, saddle prostheses, allografts and autoclaved and re-implanted autograft techniques. Allograft became the favored approach to reconstruction considering that muscle reattachment and soft tissue coverage were more straightforward compared to metal prosthetic reconstruction (7). The early results of allograft reconstruction were encouraging, but highly variable and dependent on graft fusion, which barely approached 50% (8).
The FVFG possesses several unique advantages over nonvascularized bone allo or autograft due to from the vascularized graft’s biologic superiority and subsequent ability to adapt to the hostile environment in which it is typically transplanted. The reported fracture rate of nonvascularized tibial allograft in spinopelvic fixation is reported at 50% at 6 months (9). However, in vascularized bone, the risk of fracture is minimized because the osteocytes remain viable, thus minimizing creeping substitution and maximizing primary bone healing. Graft consolidation is accelerated resulting in increased union rapidity, thereby decreasing morbidity. FVFGs have fared well in terms of union in our experience. The time to union averaged 8 months (range, 3–10 months) with only one noted FVFG fracture in twenty-four patients (10-12). Although, FVFGs in patients receiving adjuvant chemotherapy exhibited increased time to union, all FVFG achieved union (10). Other options for vascularized osseous reconstruction of the spine include pedicled rib flaps in the thoracic spine and free iliac crest vascularized bone grafts in the mobile spine, sacrum, and ilium (9). These sources of vascularized bone have several shortcomings compared to the fibula. The ribs are curved and less stable, resulting in a less anatomic construct. As well, they are generally limited to thoracic reconstruction. The vascularized iliac crest graft yields a comparatively small length of bone, which is intolerant of osteotomies, and creates a highly morbid donor site. In contrast, the fibula can yield up to 26 cm of bone, and can be osteotomized multiple times without disrupting the perfusion to the bone. This allows for double-strut designs for pelvectomy defects (Figure 1), inverted “V” configurations for total sacrectomy reconstructions (Figure 2) and single or multi barrel segments for vertebrectomy defects (2). The donor site is well-tolerated and associated with minimal morbidity.
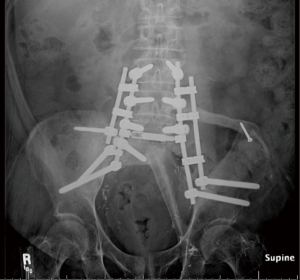
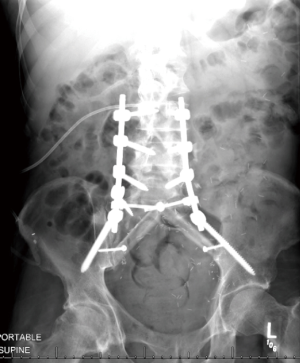
Relationship to survival
While the use of FVFG is not expected to impact disease-free survival, one of the criticisms many have with the free fibula flap reconstruction is the potential morbidity inflicted upon patients with a limited life expectancy. On the contrary, Houdek and colleagues identified substantial survival in their patient cohort, reporting 3-, 5-, and 10-year disease-free survival rates of 81%, 72%, and 48%, respectively (3). Considering this patient population’s improved life expectancies, the relevance of the superior biomechanical and biologic properties of FVFG supplemented instrumentation are amplified.
Biomechanical benefits and complications
In vitro, a cadaveric study demonstrated spinopelvic fixation supplemented with fibular strut allograft doubled the load to failure from 560 to 1,125 N (13). Although this in vitro study has not been replicated with vascularized strut grafts, it is likely that the biologically-active, vascularized fibula strengthens such a construct even further. In a canine model, the transplanted vascularized fibula is able to hypertrophy to the stress and strain of its new environment in accordance with Wolf’s law demonstrating increased stiffness and stability compared to non-vascularized grafts at 3 months with continued mechanical improvement at 6 months (14). Regarding spinal and spinopelvic reconstructions, Houdek and colleagues report a 15% FVFG fracture rate (2 of 13) and an 8% nonunion rate (1 of 13) (3). The decrease in structural failure rate is significant compared to tibial allograft which fails 50% of the time structurally in spinal and spinopelvic reconstructions (9). The two FVFG fractures and one nonunion were revised from a two-bar to a four-bar construct. Houdek’s proposed remedy for FVFG failure in spinal and spinopelvic reconstruction is the use of a four-bar over a two-bar posterior instrumentation construct (3). We use the four-bar construct on a case by case basis at our institution; however, given the morbidity associated with construct failure, movement toward a primary four-bar construct is something to consider. Plastic surgeons can mobilize the posterior trunk musculature in order to reliably cover this bulkier instrumentation.
Overall complications
Houdek et al. report an overall complication rate of 83%, the majority of which were related to the magnitude of the resection and not necessarily related to the FVFG. This patient population is prone to surgical site complications for a variety of reasons, including their numerous comorbidities, frequent use of neoadjuvant chemoradiation, prior surgeries, and the need for lengthy instrumentation constructs and significant dead space creation. There were six deep wound infections and five wound dehiscences. Twelve patients (50%) required a reoperation for treatment of a wound complication. Seven underwent irrigation and debridement and one patient required CT guided abscess drainage (3).
Based on our anecdotal institutional experience, we believe these wound-related complications can be minimized through a number of practices including preoperative nutritional optimization, postoperative prophylactic intravenous antibiotics during the hospital stay, intraoperative antibiotic irrigation, the liberal, long-term (weeks to months) use of closed-suction drains, dead space obliteration with well-vascularized soft tissue flaps, and strict postoperative activity restrictions designed to offload pressure on the surgical sites.
We also believe staging these resections to be a risk-reducing maneuver; spreading out the physiologic demand of such an extensive resection over the course of 2 days is safer for both the patient and the surgical team (15). The fibula can be safely and reliably dissected in the supine, lateral, or prone positions. This allows for a flexible surgical plan. At our institution, we prefer to dissect the fibula when the patient is in either the supine or lateral position. In general, the first stage of a total sacrectomy is done with the patient in the supine position. We use this opportunity to dissect the entire fibula flap with the exception of ligating the peroneal vessels. The fibula is then secured and “banked” in situ, and the skin incision provisionally closed. During the second stage, the fibula is rendered ischemic and revascularized at the recipient site. Conversely, for mobile spine defects, the first stage is typically performed in the prone position and the second stage with the patient in lateral decubitus; in this clinical scenario, we harvest the fibula during the second stage, avoiding the “banking” step. We believe that a second team should dissect fibula while the extirpative team performs the resection, in order to minimize operative time.
Pedicle thrombosis remains a risk of any free flap procedure and the choice of a FVFG over allograft must be balanced with the need for structural support. Most spine and plastic surgeons do not monitor the microvascular anastomosis of the free fibula flap when used in spinal or sacral applications. The bone is typically buried deep within the body and therefore the incorporation of a monitoring skin paddle is not possible. Such a skin paddle is otherwise useful for estimating fibula perfusion, by examining its color, temperature, turgor, capillary refill, and arterial and venous Doppler signals. Implantable Doppler probes also exist, which can be placed around the fibula’s pedicle and detect flow in the absence of a skin paddle. Although placing such probes is technically feasible in spinal and sacral applications, if a disruption in flow were to be detected, it would warrant a return to the operating room for exploration. Unfortunately, the morbidity of an additional lengthy operation for flap salvage after two lengthy surgical stages in the preceding days, is likely too great to justify. Therefore, most plastic and spine surgeons with experience doing these procedures leave the flap unmonitored, with the understanding that if the pedicle were to thrombose, the bone would still have utility as a standard nonvascularized autograft.
Functional outcomes
The translation of the clear biomechanical advantages of FVFG into quantifiable functional improvement remains complex. The functional outcome scores in the Houdek paper (MSTS 53%) are similar to those reported for a series of fifty-one type 1 internal hemipelvectomy patients reconstructed with a FVFG at our institution (MSTS 62%) (3,11). However, Houdek’s patient population included internal hemipelvectomies, sacrectomy, and vertebrectomies, which are very dissimilar regarding the anatomy resected and the functional disability incurred. As such, although Houdek and colleagues reconstructed each of these defects with a FVFG, the variation in resection challenges any meaningful assessment of functional outcomes. While the mean MSTS score in the paper was 53%, there was a wide range (13% to 87%) highlighting the case heterogeneity. Again, it is difficult to know what factors may be attributed to the differences in functional outcome among the patients in their cohort and whether the use of FVFG played a role. In spinal and spinopelvic resections, an important factor affecting functional outcome is the level and number of nerve roots disrupted. Information regarding the nerve root sacrifice was not included in the paper. Of note, the MSTS score did improve over time from a mean of 44% at 6 months to 51% at 1 year. It is unknown, however, whether the changes in the MSTS score observed in the Houdek et al. paper were clinically significant.
Our criticisms of the paper are few. Among them, MSTS functional outcome methodology should have been reported in greater detail. As well, the majority of the cases in this paper were sacral and spine. The four iliac cases should either have been separated out or images shown. A type 1 internal hemipelvectomy has a much different biomechanical profile than a sacrectomy with or without internal hemipelvectomy.
Conclusions
The FVFG represents a more reliable form of biologic reconstruction for spinal, and spinopelvic defects with biomechanical superiority, higher rates of osseous union, and resistance to adjuvant oncologic treatments. The functional and survival benefits of this technique remain obscured by the rarity of cases, breadth of pathology, and the variation in anatomical reconstructions which the FVFG is utilized to treat. It is unlikely the limit of the FVFG has been reached in regards to its capabilities for oncologic reconstruction. Critical analysis of outcomes remains a challenge in fields like orthopedic and spine oncology due to a paucity of cases. Continued expansion of this technique will depend on the imagination and cooperation of orthopaedic oncologists, spine surgeons, and plastic surgeons across the major centers endeavoring to improve outcomes and push the boundaries of these complex reconstructions.
Acknowledgments
None.
Footnote
Conflicts of Interest: The authors have no conflicts of interest to declare.
References
- Taylor GI, Miller GD, Ham FJ. The free vascularized bone graft. A clinical extension of microvascular techniques. Plast Reconstr Surg 1975;55:533-44. [Crossref] [PubMed]
- Jones NF, Swartz WM, Mears DC, et al. The "double barrel" free vascularized fibular bone graft. Plast Reconstr Surg 1988;81:378-85. [Crossref] [PubMed]
- Houdek MT, Rose PS, Bakri K, et al. Outcomes and Complications of Reconstruction with Use of Free Vascularized Fibular Graft for Spinal and Pelvic Defects Following Resection of a Malignant Tumor. J Bone Joint Surg Am 2017;99:e69. [Crossref] [PubMed]
- Enneking WF, Dunham WK. Resection and reconstruction for primary neoplasms involving the innominate bone. J Bone Joint Surg Am 1978;60:731-46. [Crossref] [PubMed]
- Huth JF, Eckardt JJ, Pignatti G, et al. Resection of malignant bone tumors of the pelvic girdle without extremity amputation. Arch Surg 1988;123:1121-4. [Crossref] [PubMed]
- Yuen A, Ek ET, Choong PF. Research: Is resection of tumours involving the pelvic ring justified?: A review of 49 consecutive cases. Int Semin Surg Oncol 2005;2:9. [Crossref] [PubMed]
- Wedemeyer C, Kauther MD. Hemipelvectomy- only a salvage therapy? Orthop Rev (Pavia) 2011;3:e4. [Crossref] [PubMed]
- O'Connor MI, Sim FH. Salvage of the limb in the treatment of malignant pelvic tumors. J Bone Joint Surg Am 1989;71:481-94. [Crossref] [PubMed]
- Moran SL, Bakri K, Mardini S, Shin AY, Bishop AT. The use of vascularized fibular grafts for the reconstruction of spinal and sacral defects. Microsurgery 2009;29:393-400. [Crossref] [PubMed]
- Chang DW, Fortin AJ, Oates SD, et al. Reconstruction of the pelvic ring with vascularized double-strut fibular flap following internal hemipelvectomy. Plast Reconstr Surg 2008;121:1993-2000. [Crossref] [PubMed]
- Chao AH, Neimanis SA, Chang DW, et al. Reconstruction after internal hemipelvectomy: outcomes and reconstructive algorithm. Ann Plast Surg 2015;74:342-9. [Crossref] [PubMed]
- Garvey PB, Clemens MW, Rhines LD, et al. Vertical rectus abdominis musculocutaneous flow-through flap to a free fibula flap for total sacrectomy reconstruction. Microsurgery 2013;33:32-8. [Crossref] [PubMed]
- Eastlack RK, Yaszemski MJ, Huddleston PM. The Biomechanical Strength of the Sacral Reconstruction After Total Sacrectomy Utilizing Bilateral Fibular A-Frame or Truss Technique. Spine J 2012;12:161. [Crossref]
- Shaffer JW, Davy DT, Field GA, et al. The superiority of vascularized compared to nonvascularized rib grafts in spine surgery shown by biological and physical methods. Spine (Phila Pa 1976) 1988;13:1150-4. [Crossref] [PubMed]
- Ramamurthy R, Bose JC, Muthusamy V, et al. Staged sacrectomy--an adaptive approach. J Neurosurg Spine 2009;11:285-94. [Crossref] [PubMed]