Quantitative analysis of indirect decompression in extreme lateral interbody fusion and posterior spinal fusion with a percutaneous pedicle screw system for lumbar spinal stenosis
Introduction
Lumbar spinal stenosis (LSS) is a degenerative disease caused by spinal canal narrowing and compression of the dural sac (DS) and spinal nerve roots associated with aging. Surgical treatment is recommended for symptomatic LSS patients who do not respond to conservative therapy. Standard surgeries for LSS are decompression alone and decompression plus spinal fusion using a pedicle screw and rod system with bone graft materials in combination with lumbar interbody fusion (1). Furthermore, as an alternative minimally invasive spinal surgery, extreme lateral interbody fusion (XLIF), which is associated with indirect decompressive effects and the compressed lumbar spinal canal and neuroforamen, has been increasingly utilized to treat LSS (2,3).
Several reports have shown good improvement in the radiographic outcomes of indirect decompression by analyzing parameters such as disc height (DH) or central canal area and comparing conditions before and after XLIF (2-8). Notably, in 2017, Lang et al. performed a detailed review of indirect decompression of XLIF and focused on clinical and radiographic assessments using radiography, computed tomography (CT), and magnetic resonance imaging (MRI); they found a wide range of percentage increases in the central canal areas after surgery (9). They also discussed predictive factors for indirect decompression and proposed the XLIF cage width to have a potential influence on indirect decompression; however, contrast cage position, cage height, cage type, side of approach, presence of facet arthropathy, spinal level, number of operated spinal levels, and additional instrumentation were not considered influential factors. In addition, Wang et al. found bony lateral recess stenosis to be a significant preoperative predictor of failure of indirect decompression after XLIF (6). However, the distinct mechanism and predictive factors for indirect decompression are controversial.
The purpose of the current study is to clarify the distinct influential factors for indirect decompression using clinical and radiographical quantitative analyses of the lumbar spine before and after XLIF. We also examined factors that influence clinical improvements in XLIF.
Methods
Patients
Between March 2015 and June 2016, 37 patients (26 men, 11 women) with LSS underwent XLIF plus posterior spinal fixation using a percutaneous pedicle screw (PPS) system at our hospital. All patients whose individual follow-up period was more than 12 months were assessed during this study.
Surgical procedure
The XLIF procedure has been described previously (10). Each patient was implanted with a polyetheretherketone XLIF cage implant (CoRoent XL; NuVasive, Inc., San Diego, CA, USA) filled with the harvested autologous bone graft or spongy bone graft material (Refit; HOYA Technosurgical Corp., Tokyo, Japan) in the disc space. Then, bilateral PPS fixation was performed under fluoroscopic guidance (Precept; NuVasive Inc., San Diego, CA, USA).
Clinical assessment
Clinical improvements in neurological symptoms were evaluated using the Japanese Orthopaedic Association (JOA) score (range, −9 to 29) and JOA score improvement rate using the following formula: (post-JOA − pre-JOA)/(29 − pre-JOA) ×100.
Radiographic evaluation
All radiographic images obtained using plain radiography, MRI, and CT were digitally stored in a medical center picture archiving and collecting system (PACS), and all radiographic parameters were measured using the PACS measurement tools and a computer analysis system (HOPE DrABLE-EX OSS V2; Fujitsu Ltd, Tokyo, Japan).
The transverse area of the DS (DS area) and of the ligamentous flavum (LF area) before and after surgery at the middle of the operated level were evaluated using axial MRI for all patients. Then, the rate of change (RC) of the DS area (RC-DS) was calculated as follows: DS area after surgery/DS area before surgery ×100. The RC of the LF (RC-LF) was calculated as follows: LF area after surgery/LF area before surgery ×100. The length of the intervertebral disc bulge (DB length) from the middle point of the line connecting the upper and lower posterior ends of the vertebral bodies to the endpoint of the posteriorly protruded intervertebral disc before and after surgery at the operated level were measured using the middle section of the sagittal MRI findings for all patients. The RC of the DB length (RC-DB) was calculated as follows: DB length after surgery/DB length before surgery ×100. Furthermore, we divided all surgical sites into the small expansion group (SE group; RC-DS <150%) and large expansion group (LE group; RC-DS ≥200%) according to the degree of RC-DS.
Bony union of the grafted bone was distinctly evaluated using postoperative CT images for all patients to assess consecutive trabecular cancellous bony fusion between the grafted bone and the adjacent vertebral bodies. We divided all surgical sites into the slip-vertebra group and nonslip-vertebra group according to the existence of spondylolisthesis and then evaluated them using preoperative sagittal CT images. In the slip-vertebra group, the length of the slip distance (SD length) before and after surgery at the operated level was evaluated using preoperative and postoperative sagittal CT images. Then, the RC of the SD length (RC-SD) was calculated as follows: SD length after surgery/SD length before surgery ×100.
Statistical analysis
Differences between the two groups were analyzed using the Mann-Whitney U test. Statistical analyses were performed using Sigma Plot 10.0 and Sigma Stat 3.5 for Windows (Microsoft Corp., Seattle, WA, USA).
Results
For all patients, the mean age at the time of surgery was 69.5±10.3 years, the mean overall follow-up period was 16.9±5.4 months, one-level surgery was performed for 27 patients, and two-level surgery was performed for 10 patients. Plain radiography, MRI, and CT were performed for all patients preoperatively and postoperatively, and the latest postoperative CT and MRI results were obtained 13.6±5.8 and 11.9±5.7 months after surgery, respectively. The mean operative time for all patients was 239±51 min, and the mean operative time for single-level surgery was 171 min. Estimated blood loss was 34.9±30 mL. There were no major medical complications, and reoperation for recurrence of LSS or for postoperative spinal instability was not performed. Some patients had mild anterior thigh numbness and weakness on the left side after surgery; however, it spontaneously disappeared within 2 weeks after surgery. The bony union rate of the grafted bone was 85.1% for all patients after surgery. Cage subsidence with endplate collapse was seen in three cases (6.4%); however, bony union was gained at the individual levels, and there was no case of symptomatic subsidence. The overall results of the JOA score and JOA improvement score are shown in Table 1. JOA scores had significantly improved during the final follow-up compared with that before surgery for all patients, and the overall JOA improvement rate for all patients was 72.4%±24.8% (Table 1).
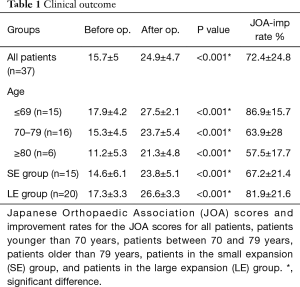
Full table
Additionally, there was a significant difference in the improvement rate between patients younger than 70 years and those older than 69 years (P=0.002). The overall results of DS area, LF area, and DB length before and after operation in all patients are shown in Table 2. There was a significant difference in the DS area, LF area, and DB length for all patients before (Figure 1A,B,C) and after (Figure 1D,E,F) surgery, and the RC-DS, RC-LF and RC-DB length were 202.5%±79.1%, 73.9%±13.1% and 36.5%±44.8%, respectively (Table 2). The 47 surgical sites of all patients were divided into the slip-vertebra group (n=24) and the nonslip-vertebra group (n=23). There was a significant difference in the SD length in the slip-vertebra group before (5.9±2 mm) and after (3.4±1.6 mm) surgery (P<0.001), and the RC-SD was 43.4%±22.3%. The overall results of the DS area, LF area, and DB length before and after operation in both the slip-vertebra group and nonslip-vertebra group are shown in Table 2. The RC-DS in the slip-vertebra group and nonslip-vertebra group were 204.9%±70.3% and 199.9%±88.9%, respectively (Table 2), and no significant difference was found between the two groups (P=0.578). In addition, the RC-DB in the slip-vertebra group and nonslip-vertebra group were 28.6%±35.1% and 44.9%±52.6%, respectively (Table 2), and no significant difference was noted between the two groups (P=0.458).
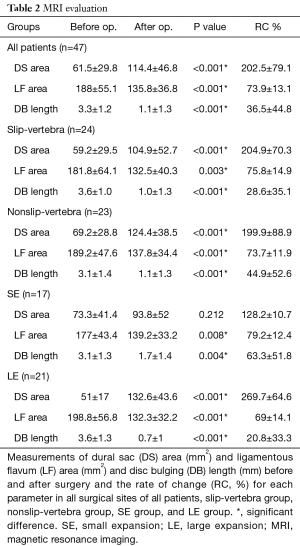
Full table
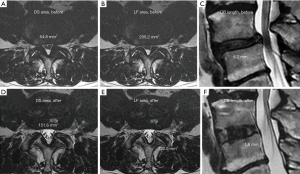
We selected the patients for the SE group (n=15; level n=17) and LE group (n=20; level n=21) according to the RC-DS. The overall results of DS area, LF area, and DB length before and after operation in patients in both SE and LE groups and the RC % are shown in Table 2. The JOA score for the SE group had significantly improved at the final follow-up compared with that before surgery, and the JOA improvement rate was 67.2%±21.4% (Table 1). There was no significant difference in the DS area of the SE group before and after surgery, and the RC-DS was 128.2%±10.7%. There was a significant difference in the LF area and DB length before and after surgery, and the RC-LF and RC-DB was 79.2%±12.4% and 63.3%±51.8%, respectively. The JOA score of the LE group had significantly improved at the final follow-up compared with that before surgery, and the JOA improvement rate was 81.9%±21.6% (Table 1). There was a significant difference in the DS area and LF area before and after surgery, respectively, and the RC-DS, RC-LF, and RC-DB was 269.7%±64.6%, 69%±14.1%, and 20.8%±33.3%, respectively (Table 2). A significant difference was found in the JOA improvement rate between patients in the SE group and the LE group (P=0.02). Moreover, no significant difference in the RC-LF was noted for patients in the SE group and the LE group (P=0.726).
A summary of distinct parameters for both SE and LE groups is shown in Table 3. No significant difference in the patient’s age during surgery was found between the SE group and the LE group (P=0.07). We checked whether the duration from the surgery to the postoperative MRI obtained for assessment influenced the degree of expansion of the DS area; the durations for the SE and LE groups were 10.8±6.9 and 12.9±5.4 months, respectively, which were not significantly different (P=0.303). Of the 17 surgical sites in the SE group, 6 belonged to the slip-vertebra group and 11 to the nonslip-vertebra group. Moreover, of the 21 surgical sites in the LE group, 12 were allocated to the slip-vertebra group and 9 to the nonslip-vertebra group. Thus, 64.7% (11/17) of the surgical sites in the SE group were related to non-slippage vertebra, whereas 42.9% (9/21) of the surgical sites in the LE group were related to non-slippage vertebra.
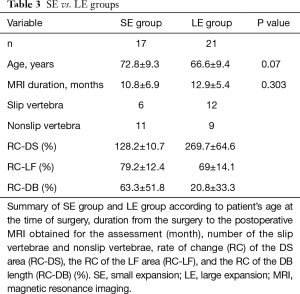
Full table
Discussion
Although the gold standard treatment for LSS is decompression, removing too much of the posterior elements and excessive facetectomy can cause postoperative spinal instability of the operative level (11). To resolve this complication, minimally decompressive surgery has been introduced (11-13). Another procedure for LSS is decompression plus spinal fusion with bone graft materials including posterior lumbar interbody fusion and transforaminal lumbar interbody fusion (TLIF). However, spinal fusion is often associated with more complications than decompression alone, such as deep infection, implant fracture, bleeding, or adjacent segment disease (14). Moreover, postoperative epidural hematoma and intraoperative cerebrospinal fluid leakage are common complications of spinal decompression with or without spinal instrumentation. For example, the incidence of a symptomatic hematoma requiring emergency evacuation was 0.5% during lumbar laminectomy and 0.67% during posterior lumbar interbody fusion (15). This is an advantage of XLIF that applies to spinal fusion without decompression. Because the XLIF interbody cage with a large footprint spans both lateral cortical rims while preserving the anterior longitudinal ligament, it restores DH and provides highly rigid segmental stability, particularly in combination with additional posterior PPS fixation, with minimal damage of the paravertebral muscles. As a result, it allows indirect decompression. Clinical improvements in leg pain and intermittent claudication for XLIF with indirect decompression and TLIF with direct decompression are comparable (16). Because of the sophisticated XLIF retractor system and directional electromyography stimulating device, a surgeon can implant the XLIF cage safely within 5 cm of the lateral skin incision. Besides, XLIF has a potential risk of complications, including thigh dysesthesia, psoas weakness, infection, rupture of the anterior longitudinal ligament, cage migration, subsidence of the intervertebral cage, myocardial infarction, and bowel perforation (17). We observed no major complications during this study. Only minor adverse events such as transient anterior thigh numbness and weakness were found, similar to that in a previous report (18). Our clinical outcome was a significant improvement in the JOA score, particularly for patients younger than 70 years, which is likely reasonable because of the aging effects associated with general physical activity (19).
With regard to indirect decompression after XLIF for all patients, we quantitatively examined the area of the DS and LF both before and after surgery and found approximately 200% expansion of the DS after surgery, which was associated with an approximately 25% reduction of the area of the LF. The review by Lang et al. indicated that the expansion rate for the central canal area after XLIF had a wide range (116.5%±45.2% to 190%±67.2%) (9). Furthermore, we selected the patients for SE and LE groups based on the expansion rate of the DS area and evaluated the change in DB length before and after surgery, and we found that the bulging was significantly shortened in the LE group than in the SE group, implicating that indirect decompression is influenced by how much the bulge is reduced after XLIF. In other words, we may expect indirect decompression with XLIF in cases of LSS accompanied by rather large bulging. Elowitz et al. previously reported that the DS area increased an average of 143% after XLIF with LSS, and they implied that indirect decompression may be related to stretching and unbuckling of the LF and decreased DB; however, the quantitative analysis was not able to show the mechanism of indirect decompression (20). In this study, we suggest that indirect decompression may be more related to a decrease in the bulging than in the stretching and unbuckling of the LF. In addition, we found a tendency toward less expansion of the DS at the surgical sites without spondylolisthesis following XLIF, suggesting that decompression alone is likely more suitable for typical LSS without spondylolisthesis or any spinal deformity as a first-choice operation, considering surgical invasion and medical costs. This is reasonable, as the improvement rate of the JOA score for the LE group after surgery was significantly higher than that for the SE group.
Conclusions
We found that XLIF plus PPS fixation led to significant clinical improvement in patients with LSS. Since indirect decompression after XLIF was particularly influenced by the degree of reduction in disc bulging, we may expect indirect decompression with XLIF in cases of LSS accompanied by rather large bulging.
Acknowledgments
None.
Footnote
Conflicts of Interest: The authors have no conflicts of interest to declare.
Ethical Statement: The authors are accountable for all aspects of the work in ensuring that questions related to the accuracy or integrity of any part of the work are appropriately investigated and resolved. This study was approved by the Ethics Committee of Shimonoseki City Hospital (2019SCHEC-011), and informed consent was obtained from the patients for publication of this study and any accompanying images.
References
- Postacchini F. Surgical management of lumbar spinal stenosis. Spine (Phila Pa 1976) 1999;24:1043-7. [Crossref] [PubMed]
- Youssef JA, McAfee PC, Patty CA, et al. Minimally invasive surgery: lateral approach interbody fusion: results and review. Spine (Phila Pa 1976) 2010;35:S302-11. [Crossref] [PubMed]
- Pereira EA, Farwana M, Lam KS. Extreme lateral interbody fusion relieves symptoms of spinal stenosis and low-grade spondylolisthesis by indirect decompression in complex patients. J Clin Neurosci 2017;35:56-61. [Crossref] [PubMed]
- Oliveira L, Marchi L, Coutinho E, et al. A radiographic assessment of the ability of the extreme lateral interbody fusion procedure to indirectly decompress the neural elements. Spine (Phila Pa 1976) 2010;35:S331-7. [Crossref] [PubMed]
- Tessitore E, Molliqaj G, Schaller K, et al. Extreme lateral interbody fusion (XLIF): A single-center clinical and radiological follow-up study of 20 patients. J Clin Neurosci 2017;36:76-9. [Crossref] [PubMed]
- Wang TY, Nayar G, Brown CR, et al. Bony lateral recess stenosis and other radiographic predictors of failed indirect decompression via extreme lateral interbody fusion: multi-institutional analysis of 101 consecutive spinal levels. World Neurosurg 2017;106:819-26. [Crossref] [PubMed]
- Alimi M, Lang G, Navarro-Ramirez R, et al. The impact of cage dimensions, positioning, and side of approach in extreme lateral interbody fusion. Clin Spine Surg 2018;31:E42-9. [Crossref] [PubMed]
- Navarro-Ramirez R, Berlin C, Lang G, et al. A new volumetric radiologic method to assess indirect decompression after extreme lateral interbody fusion using high-resolution intraoperative computed tomography. World Neurosurg 2018;109:59-67. [Crossref] [PubMed]
- Lang G, Perrech M, Navarro-Ramirez R, et al. Potential and limitations of neural decompression in extreme lateral interbody fusion - a systematic review. World Neurosurg 2017;101:99-113. [Crossref] [PubMed]
- Ozgur BM, Aryan HE, Pimenta L, et al. Extreme lateral interbody fusion (XLIF): a novel surgical technique for anterior lumbar interbody fusion. Spine J 2006;6:435-43. [Crossref] [PubMed]
- Ghogawala Z, Dziura J, Butler WE, et al. Laminectomy plus fusion versus laminectomy alone for lumbar spondylolisthesis. N Engl J Med 2016;374:1424-34. [Crossref] [PubMed]
- Hatta Y, Shiraishi T, Sakamoto A, et al. Muscle-preserving interlaminar decompression for the lumbar spine: a minimally invasive new procedure for lumbar spinal canal stenosis. Spine (Phila Pa 1976) 2009;34:E276-80. [Crossref] [PubMed]
- Watanabe K, Hosoya T, Shiraishi T, et al. Lumbar spinous process-splitting laminectomy for lumbar canal stenosis. Technical note. J Neurosurg Spine 2005;3:405-8. [Crossref] [PubMed]
- Deyo RA, Mirza SK, Martin BI, et al. Trends, major medical complications, and charges associated with surgery for lumbar spinal stenosis in older adults. JAMA 2010;303:1259-65. [Crossref] [PubMed]
- Aono H, Ohwada T, Hosono N, et al. Incidence of postoperative symptomatic epidural hematoma in spinal decompression surgery. J Neurosurg Spine 2011;15:202-5. [Crossref] [PubMed]
- Sembrano JN, Tohmeh A, Isaacs R. SOLAS degenerative study group: Two-year comparative outcomes of MIS lateral and MIS transforaminal interbody fusion in the treatment of degenerative spondylolisthesis: part I: clinical findings. Spine (Phila Pa 1976) 2016;41:S123-32. [PubMed]
- Malham GM, Parker RM, Blecher CM, et al. Assessment and classification of subsidence after lateral interbody fusion using serial computed tomography. J Neurosurg Spine 2015;23:589-97. [Crossref] [PubMed]
- Januszewski J, Beckman JM, Bach K, et al. Indirect decompression and reduction of lumbar spondylolisthesis does not result in higher rates of immediate and long term complications. J Clin Neurosci 2017;45:218-22. [Crossref] [PubMed]
- Nomura H, Yanagisawa Y, Arima J. Long-term outcome of patients undergoing microscopic lumbar spinous process-splitting laminectomy. J Spine 2016;5:1000343. [Crossref]
- Elowitz EH, Yanni DS, Chwajol M, et al. Evaluation of indirect decompression of the lumbar spinal canal following minimally invasive lateral transpsoas interbody fusion: radiographic and outcome analysis. Minim Invasive Neurosurg 2011;54:201-6. [Crossref] [PubMed]