Standalone lordotic endoscopic wedge lumbar interbody fusion (LEW-LIF™) with a threaded cylindrical peek cage: report of two cases
Introduction
Modern lumbar Interbody fusion procedures have become mainstream in reconstructive spinal surgeries for spondylolisthesis and decompression-induced instability for stenosis-related neurogenic claudication symptoms and sciatica-type low back and leg pain (1). While the addition of interbody fusion cages to a traditional open posterior lumbar decompression and instrumented posterolateral fusion was associated with increased operative time, blood loss, and higher complication rates (2), modern interbody fusion technologies have substantially decreased these drawbacks and have capitalized on their advantages (3). Namely, restoration of neuroforaminal height, lumbar lordosis, and improved fusion rates are the core principles that have driven technology advances and contributed to improved clinical outcomes (4).
Most contemporary lumbar interbody fusion systems require the use of supplemental posterior pedicle-screw based instrumentation for added stability, and their regulatory approval process mandates it in the United States and many European countries (5). There has been significant progress in minimizing the surgical access trauma with modern minimally invasive spinal surgery techniques (MISST). Reduced tissue trauma, blood loss, and improved return to function data have been obtained supporting the use of MISST in the deployment of interbody fusion cages (6). Many MISST interbody fusion cages have become commercially available some of which can be placed into the intervertebral disc space via the transforaminal approach (7). The associated MISSTs can be grossly divided into non-visualized and directly visualized procedures. In the non-visualized procedures, a cannulated interbody fusion cage is typically placed over a guide wire into the intervertebral disc space after its preparation with discectomy and endplate decortication using cannulated instruments, such as shavers and rongeurs. In directly visualized procedures, video-endoscopic equipment is employed throughout these steps of the interbody fusion procedure with the intent of minimizing the use of ionizing radiation and aiding in the direct decompression while visualizing the neural elements unswervingly (8).
Recent technological advances have shown the feasibility of performing standalone endoscopic transforaminal interbody fusion procedures (8-10). The impetus for this development was to further simplify the MISST lumbar decompression and reconstructive procedure for outpatient surgery in an ambulatory surgery center (ASC) setting by avoiding the need for posterior supplemental fixation. The FDA approved such an interbody fusion system in 2016 for standalone use in one- and two-level lumbar surgeries (8). The application of standalone transforaminal lordotic endoscopic wedge lumbar interbody fusion (LEW-LIF™) techniques has been recently reported while achieving favorable clinical outcomes in the majority of patients (8). The clinical and surgical LEW-LIF™ protocols developed by the senior author of this case report are based on his clinical experience with a standalone expandable interbody fusion system. Subsidence-related less favorable clinical outcomes induced by the implant’s high-stress concentration prompted the development of a customized endoscopic lumbar decompression and interbody fusion procedure which assesses patient-specific stress distribution patterns across the interbody fusion level intraoperatively in real time to achieve a stress-neutral and biomechanically stable fusion (8).
The focus of this report of two cases is to show the feasibility of a standalone stress-neutral lumbar endoscopic decompression interbody fusion procedure done for sciatica-type low back and leg pain due to spinal stenosis and instability limited to grade I spondylolisthesis. The authors employed a non-expandable threaded interbody fusion implant made from polyether ether ketone (PEEK) that could be deployed via a guidewire during a directly visualized endoscopic transforaminal surgery. This case report describes how to assess patient-specific stress distribution patterns across the interbody fusion space intraoperatively and how to use them to achieve biomechanically stable fusion without creating areas of stress concentration using the LEW-LIF™ protocols.
Materials & methods
The first author’s surgical techniques are a modification of the transforaminal approach originally described by Hoogland et al. (11) and further popularized by Ruetten et al. who incorporated it into his full-endoscopic methodology (12). This platform was used to place a threaded interbody fusion device into the intervertebral space to treat instability-induced symptoms of degenerated disc disease that have proven refractory to non-operative treatment. The patients subject to this report of two cases were selected using the following inclusion criteria:
- Radiculopathy, dysesthesias, and decreased motor function;
- Foraminal or lateral recess stenosis confirmed on magnetic resonance images (MRI) and computed tomography (CT) scans;
- Spondylolisthesis limited to grade I;
- Minimum of 12 weeks of failed conservative care with nonsteroidal anti-inflammatories, physical therapy, and epidural steroid injections.
The following exclusion criteria resulted in a patient not being considered for the Lew-LIF™:
- Spondylolisthesis grade II or greater;
- Severe central stenosis (less than 100 mm2) (13);
- Massive facet hypertrophy;
- Infection, and;
- Metastatic disease.
All patients in this consecutive case series provided informed consent and IRB approval was obtained (CEIFUS 106-19). Written informed consent was obtained from the patient for publication of this case report and any accompanying images. A copy of the written consent is available for review by the Editor-in-Chief of this journal.
LEW-LIF™ surgical technique & implant
The “outside-in” (11,12) transforaminal surgical technique is the backbone of the LEW-LIF™ procedure (8). The working sheath is placed into the safe zone between the pedicle, traversing, and exiting nerve root (14). For discectomy and endplate preparation, the working sheath may be advanced into the interspace using procedural steps of the “inside-out” (15) techniques. The interbody fusion procedure has been described in great detail (9). Both patients were operated in the prone position under sedation and monitored anesthesia care. Endoscopic instruments required to complete the decompression and interspace preparation include motorized drills, Kerrison rongeurs, trephines most of which were deployed through the central 4.1 mm inner working channel of the spinal endoscope. The main goal of the “outside-in” foraminoplasty is the near total removal of the superior articular process (SAP) via osteotomy from the distal pedicle to prepare the introitus for the cage insertion. Attention should be paid to align the instruments and the cage parallel to the endplates. In patients with collapsed motion lumbar segments, additional resection of the inferior articular process (IAP) or the superomedial pedicle wall may be required. The exiting nerve root should be carefully retracted to minimize irritation of its dorsal root ganglion (DRG) during cage insertion. Special cannulated instruments for these steps include paddle shavers and tapes which are also used to size the implant. During the decortication of the endplates, damage to the subchondral bone should be avoided to minimize implant subsidence. The first author and his team performed the surgeries. The senior author assisted in the surgeries and the writing of this manuscript.
The interbody fusion was done using the Ortomac™ cylindrical threaded interbody fusion cage made from PEEK (Figure 1). This cage is cannulated along its center line and has two perpendicular bone graft chambers connected to the central channel, which is also used to attach the inserter. The round threaded non-expandable implant is available in 28 and 32 mm in length and in sizes from 9 to16 mm in outer diameter. The implant’s outer diameter is 6 mm smaller at the tip than at the end of the implant’s body. This design has been approved in Colombia for clinical use without the need for posterior supplemental fixation and, hence, is genuinely a standalone design. Resistance to expulsion or dislocation is achieved by the maximum distraction of the intervertebral disc space, and the snug interference fit between the threads and the surrounding bone producing a high pull-out strength of 665 N (66.5 kg; Figure 2). Biomechanical testing revealed a compression failure load at 9,150 N (915 kg), and failure of the surrounding vertebral bone measured in human cadaver specimens at 2,040 N (204 kg; Figure 3).
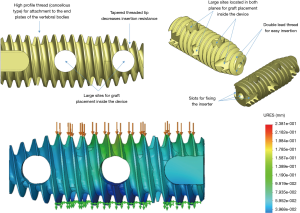
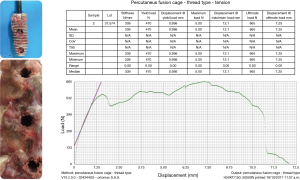
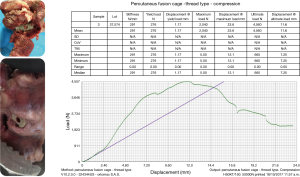
The Ortomac™ interbody fusion system consists of one cage positioned obliquely across the disc space parallel to the endplates using the LEW-LIF™ techniques. The trajectory for cage insertion is initially between 40 to 60 degrees in the axial plane and is primarily dictated by the patient’s vertical alignment of the intervertebral discs to the iliac crest. Before entering the disc space, however, the cage insertion trajectory is adjusted to match the interspace alignment prepared with the LEW-LIF™ techniques to maximize contact area with the ring apophysis, and parallel alignment to the endplates while avoiding stress concentration areas—recognized as a significant contributor to cage subsidence postoperatively. The LEW-LIF™ techniques were successfully applied in a prospective consecutive case series and mainly revolve around direct endoscopic visualization of the intervertebral disc space and the area where the interbody cage will be placed followed by real-time intraoperative measurement of the compression failure loads at the bone-implant interface using proprietary endoscopic instruments. These patient-specific intraoperative measurements are then entered into a proprietary algorithm to arrive at the most appropriate implant size maximized for best biomechanical stability and least propensity for the implant’s subsidence or loosening both of which can be caused by an over- or undersized implant or creation of areas of high-stress concentration. Before placement of the appropriately chosen implant over a nitinol guide-wire into the intervertebral disc space, local bone- or cancellous demineralized allograft should be placed into the cage’s graft chambers.
Clinical follow-up
Patients were typically evaluated at 2, 6, and 12 weeks postoperatively. At the time of this case report, the last available follow-up was 24 weeks. Clinical improvements were evaluated by calculating reductions in the visual-analog scales (VAS) (16) for leg pain ranging from no pain [0] to worst pain [10] and the Oswestry disability index (ODI) (17). The VAS and ODI scores were correlated any patient-related factors that could contribute to less favorable outcomes.
Case presentation
Case 1
A 62-year-old female presented with a 6-year history of progressively worsening right-sided sciatica-type leg and back pain with several acute on chronic episodes. The patient underwent an open discectomy at L5-S1 level several years prior. Before considering surgical treatment, she had a failed medical management with NSAIDs, physical therapy and lumbosacral support orthosis. Her preoperative diagnostic workup in the setting of multilevel lumbar degenerative disc disease and spinal stenosis also included transforaminal injections at the L3/L4 and the L5/S1 level as well as L3/4, and L5/S1 facet joints. Her preoperative plain film studies showed minimal coronal imbalance and grade I spondylolisthesis. Advanced imaging with MRI showed foraminal and lateral recess stenosis at the L3/L4 and L4/L5 level due to intraforaminal contained disc herniation. Also, there was loss of intervertebral height, a facet arthropathy producing significantly reduced neuroforaminal width of less than 1 mm in the foraminal entry- and midzone (Figure 4). The patient was considered to have symptomatic end-stage degenerative disc disease with the majority of symptoms originating from the L4/5 level. Ultimately, the decision was made to treat this patient surgically with an L3/4 transforaminal endoscopic discectomy and an L4/5 LEW-LIF™ procedure (Figure 5). The facet arthropathy pain was treated with L3/4, and L4/5 radiofrequency rhizotomy. The patient had an uneventful postoperative course and was asymptomatic at final available follow-up of 24 weeks postoperatively. At final follow-up, she reported a VAS reduction of 6 for both leg and back pain and an overall improvement of function equivalent to a 60-point reduction on the ODI rating.
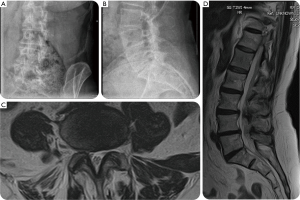
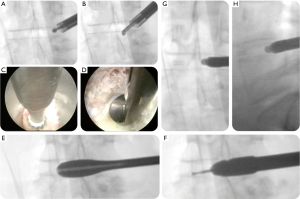
Case 2
A 79-year-old female presented with a 7-year history of progressively worsening left-sided sciatica-type leg and back pain. She presented to us with a history of 5 years of left buttock and leg pain. Extensive non-operative medical and supportive care management with NSAIDs, physical therapy and lumbosacral support was unsuccessful in spite of selective L5 nerve root blocks with transforaminal epidural steroid injection (TESI) at the L4/5 level. The patient’s plain film studies showed a L4/L5 grade I anterolisthesis grade I, and an MRI confirmed left foraminal contained disc herniation causing her foraminal and lateral recess stenosis (Figure 6). Ultimately, the patient underwent a full-endoscopic discectomy and a standalone LEW-LIF™ fusion with a lordotic endoscopic threaded standalone interbody fusion at L4/L5 which was accompanied by a radiofrequency rhizotomy for tentative treatment of axial back pain presumably caused by the patient’s concomitant facet arthropathy (Figure 6). The patient was sent home after her outpatient surgery, and her postoperative course was initially uneventful. Nine days postoperatively, she developed dysethetic leg pain on the surgical side typical of postoperative irritation of the exiting nerve root. Treatment consisted of L4/5 TESI on the approach side, gabapentin by mouth, and activity modification with frequent changes in position and a reduced walking schedule. Routine CT, MRI, and plain film studies (Figure 7) taking during her postoperative surveillance showed unchanged position of the endoscopic interbody fusion cage without any evidence of migration, or subsidence. The patient continued to improve, and at final follow-up of 24 weeks was asymptomatic. At final follow-up, this patient also reported a VAS reduction of 6 for both leg a back pain and an overall improvement of function equivalent to a 60-point reduction on the ODI rating.
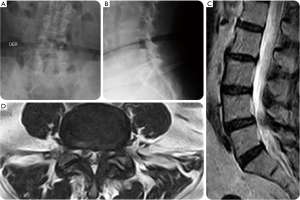
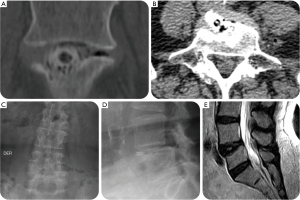
Discussion
This report of two cases illustrated the feasibility of a standalone interbody fusion using the LEW-LIF™ technique (8). The clinical relevance of a standalone interbody fusion that can be performed endoscopically through a small paraspinal skin incision using the transforaminal approach is high concerning an outpatient spinal surgery program that can be carried out in an ASC. The trend of moving spinal decompression surgeries into a more cost-effective outpatient setting has long had traction in the United States; particularly after the Centers for Medicare & Medicaid Services (CMS) substantially increased the number of approved outpatient procedural current procedural terminology (CPT) codes in 2017. Improved patient satisfaction scores with outpatient surgery, as well as lower infection and complication rates are additional incentives to apply technology advancements even towards more complicated problems such as spinal fusion (18). Hence, a simplified standalone lumbar interbody fusion not requiring the concomitant use of supplemental posterior pedicle screw fixation would lend itself beautifully to any outpatient spine surgery program because of its substantially reduced complexity. A standalone endoscopic lumbar interbody fusion would not only substantially reduce the clinical burden on the patient, but also reduce the complexity of the operational cost and problems needing to be managed by the ASC including instrument preparation and sterilization, more lengthy surgery and anesthesia times, and increased cost related to implants and payroll.
In comparison to lumbar pedicle screws, there have been numerous new product launches based on innovative interbody fusion cage technology with vertically and horizontally expandable designs to address problems related to small footprint and loss of neuroforaminal height. Many of these newer expandable cage designs are intended for either open or minimally invasive translaminar implantation. Others are suitable for transforaminal implantation as well, but very few are threaded. Most of contemporary lumbar PEEK, titanium, composite, or 3D-printed interbody fusion cages are intended to be impacted into the lumbar interspace after its adequate preparation. Impaction may violate subchondral bone the carefully decorticated of the endplates and propagate subsidence, loosening which may be followed by the expulsion of the interbody fusion cage (8). The latter has been observed in oblique lumbar interbody fusion (OLIF) surgeries with cannulated transforaminal PEEK cages (19). Also, the expansion of a lumbar interbody fusion cage itself may lead to substantial stress concentration in small contact areas between the implant and the endplates and, thus, in itself be a strong impetus to cage subsidence and new-onset postoperative pain due to loss of neuroforaminal height and fractures of the endplates (20).
The cage design of a cylindrical, cannulated, non-expandable, PEEK cage with three bone graft chambers with deep cutting threads typical of a cancellous bone screw, and a double-threaded lead-in tip seems outdated on the surface. This team of authors, however, has chosen this design intentionally for several reasons. A cylindrical design was more conducive to screwing the cage into the intervertebral disc space without impaction, thus, reducing the risk of injury to the endplates. The cancellous thread design with a lead-in tip would facilitate auto-distraction of the vertebral bodies and generate high-interference fit by maximizing cage size. The lack of an expansion mechanism was considered one of the main prerequisites to avoiding stress concentration between the implant and endplates at any portion of the joint contact area. The addition of the LEW-LIF™ techniques during surgery aided further in optimizing implant size to achieve stress-neutral implantation during surgery by determining patient-specific compression peak force directly at the bone-implant interface. The purpose of the proprietary LEW-LIF™ algorithm is to minimize common subsidence problems observed with expandable standalone cages (8).
The favorable clinical course of the two patients who underwent standalone lumbar interbody fusion endstage degenerative disc disease-related symptoms with and without spondylolisthesis showed the feasibility of the use of a non-expandable cylindrical PEEK cage for these clinical indications. There was no evidence of cage subsidence or migration in either one of our two patients on postoperative surveillance studies corroborating prove of concept of our approach with a PEEK implant whose modulus of elasticity is similar to cancellous bone and whose biocompatibility is well accepted. At final follow-up of 24 weeks postoperatively, both patients maintained their postoperative improvements and resolution of sciatica-type low back and leg pain with a 60% reduction of the respective VAS and ODI scores.
Conclusions
Standalone outpatient lumbar transforaminal endoscopic interbody fusion with a non-expandable threaded cylindrical cage is feasible. Utilization of the LEW-LIF™ endoscopic interbody fusion techniques and algorithm analyzed patient specific compression peak force directly at the bone-implant interface and aids in the most appropriate choice of implant size to achieve a stress neutral interbody fusion. Favorable clinical outcomes provide proof of concept to study long-term clinical outcomes in larger groups of patients.
Acknowledgments
None.
Footnote
Conflicts of Interest: The first author and his team of co-authors designed and marketed the standalone clyndrical interbody fusion cage and receive royalties and distributions from the sale of their product by Ortomac. Additional indirect conflicts of interest may exist due to honoraria, consultancies to other vendors and companies including Elliquence, LLC. The senior author has no direct or indirect conflicts and his involvement in this manuscript is not meant for or intended to endorse any products or push any other agenda other than the associated clinical outcomes with endoscopic standalone lumbar fusion. The senior author’s motive for assisting in the surgeries of the two patients involved in this case report and in the compilation of this clinically relevant information is by no means created and/or correlated to directly enrich anyone due to its publication. This publication was intended to substantiate contemporary endoscopic spinal surgery concepts to facilitate technology advancements.
Ethical Statement: All patients in this consecutive case series provided informed consent and IRB approval was obtained (CEIFUS 106-19). Written informed consent was obtained from the patients for publication of this case report and any accompanying images. A copy of the written consent is available for review by the Editor-in-Chief of this journal. The authors are accountable for all aspects of the work in ensuring that questions related to the accuracy or integrity of any part of the work are appropriately investigated and resolved.
References
- Seaman S, Kerezoudis P, Bydon M, et al. Titanium vs. polyetheretherketone (PEEK) interbody fusion: Meta-analysis and review of the literature. J Clin Neurosci 2017;44:23-9. [Crossref] [PubMed]
- Patel AA, Zfass-Mendez M, Lebwohl NH, et al. Minimally Invasive Versus Open Lumbar Fusion: A Comparison of Blood Loss, Surgical Complications, and Hospital Course. Iowa Orthop J 2015;35:130-4. [PubMed]
- Lin GX, Park CK, Hur JW, et al. Time Course Observation of Outcomes between Minimally Invasive Transforaminal Lumbar Interbody Fusion and Posterior Lumbar Interbody Fusion. Neurol Med Chir (Tokyo) 2019;59:222-30. [Crossref] [PubMed]
- Alvi MA, Kurian SJ, Wahood W, et al. Assessing the Difference in Clinical and Radiologic Outcomes Between Expandable Cage and Nonexpandable Cage Among Patients Undergoing Minimally Invasive Transforaminal Interbody Fusion: A Systematic Review and Meta-Analysis. World Neurosurg 2019. [Epub ahead of print]. [Crossref] [PubMed]
- Schmoelz W, Sandriesser S, Loebl O, et al. Effect of cage design, supplemental posterior instrumentation and approach on primary stability of a lumbar interbody fusion - A biomechanical in vitro study. Clin Biomech (Bristol, Avon) 2017;48:30-4. [Crossref] [PubMed]
- Regev GJ, Lador R, Salame K, et al. Minimally invasive spinal decompression surgery in diabetic patients: perioperative risks, complications and clinical outcomes compared with non-diabetic patients' cohort. Eur Spine J 2019;28:55-60. [Crossref] [PubMed]
- Zairi F, Sunna TP, Westwick HJ, et al. Mini-open oblique lumbar interbody fusion (OLIF) approach for multi-level discectomy and fusion involving L5-S1: Preliminary experience. Orthop Traumatol Surg Res 2017;103:295-9. [Crossref] [PubMed]
- Lewandrowski KU, Ransom NA, Ramírez León JF, et al. The Concept for A Standalone Lordotic Endoscopic Wedge Lumbar Interbody Fusion: The LEW-LIF. Neurospine 2019;16:82-95. [Crossref] [PubMed]
- Ahn Y, Youn MS, Heo DH. Endoscopic transforaminal lumbar interbody fusion: a comprehensive review. Expert Rev Med Devices 2019;16:373-80. [Crossref] [PubMed]
- Yang JC, Hai Y, Ding Y, et al. Percutaneous endoscopic transforaminal lumbar interbody fusion for lumbar spinal stenosis. Zhonghua Yi Xue Za Zhi 2018;98:3711-5. [PubMed]
- Hoogland T, Schubert M, Miklitz B, et al. Transforaminal posterolateral endoscopic discectomy with or without the combination of a low-dose chymopapain: a prospective randomized study in 280 consecutive cases. Spine (Phila Pa 1976) 2006;31:E890-7. [Crossref] [PubMed]
- Ruetten S, Komp M, Merk H, et al. Recurrent lumbar disc herniation after conventional discectomy: a prospective, randomized study comparing full-endoscopic interlaminar and transforaminal versus microsurgical revision. J Spinal Disord Tech 2009;22:122-9. [Crossref] [PubMed]
- Sengupta DK, Herkowitz HN. Lumbar spinal stenosis. Treatment strategies and indications for surgery. Orthop Clin North Am 2003;34:281-95. [Crossref] [PubMed]
- Kambin P, Casey K, O’Brien E, et al. Transforaminal arthroscopic decompression of lateral recess stenosis. J Neurosurg 1996;84:462-7. [Crossref] [PubMed]
- Yeung A, Roberts A, Zhu L, et al. Treatment of Soft Tissue and Bony Spinal Stenosis by a Visualized Endoscopic Transforaminal Technique Under Local Anesthesia. Neurospine 2019;16:52-62. [Crossref] [PubMed]
- Huskisson EC, Jones J, Scott PJ. Application of visual-analogue scales to the measurement of functional capacity. Rheumatol Rehabil 1976;15:185-87. [Crossref] [PubMed]
- Fairbank JC, Pynsent PB. The Oswestry Disability Index. Spine (Phila Pa 1976) 2000;25:2940-52; discussion 2952. [Crossref] [PubMed]
- Mundell BF, Gates MJ, Kerezoudis P, et al. Does patient selection account for the perceived cost savings in outpatient spine surgery? A meta-analysis of current evidence and analysis from an administrative database. J Neurosurg Spine 2018;29:687-95. [Crossref] [PubMed]
- Phan K, Maharaj M, Assem Y, et al. Review of early clinical results and complications associated with oblique lumbar interbody fusion (OLIF). J Clin Neurosci 2016;31:23-9. [Crossref] [PubMed]
- Calvo-Echenique A, Cegoñino J, Chueca R, et al. Stand-alone lumbar cage subsidence: A biomechanical sensitivity study of cage design and placement. Comput Methods Programs Biomed 2018;162:211-9. [Crossref] [PubMed]