An evaluation of the host response to an interspinous process device based on a series of spine explants: Device for Intervertebral Assisted Motion (DIAM®)
Introduction
Lumbar spinal stenosis (LSS) is a common clinical scenario with a prevalence of 20–25% in the general population (1). Clinically, LSS presents as back or leg pain that is primarily related to position or posture and is caused by a reduction in the volume of the spinal canal and compression on the thecal sac or exiting nerve roots. LSS is the most common reason for back surgery in people over the age of 65 in the United States (2). Surgical treatment for LSS is considered when conservative treatments fail to relieve pain and improve function. The current surgical standard is decompression via posterior decompressive laminectomy with or without fusion. The success rate of this procedure varies in the literature; however, recent clinical trials have suggested surgical treatment to be superior to non-operative management (3). As an alternative to wide decompressive laminectomy, interspinous process devices (IPDs) have been designed to treat patients with LSS by limiting extension.
IPDs are a type of dynamic stabilization (non-fusion) devices that are widely used in Europe for the treatment of LSS. In contrast to laminectomy with fusion or disc arthroplasty devices, IPDs can be implanted using a minimally invasive approach. After removal of a portion of the interspinous ligament, IPDs are surgically implanted into the interspinous space in order to induce local distraction of the posterior elements. The purposes of these devices are to provide stabilization after decompression, to effect local segmental lumbar kyphosis, to restore foraminal height, and to unload the facet joints (4). While they primarily limit extension at the treated level, IPDs allow for the preservation of range of motion (ROM) in other directions at the implanted level. Multiple authors have undertaken biomechanical studies and performed finite element analyses to assess the effect of IPDs on spine segment range of motion, local kyphosis, and intradiscal pressure (5-9). Biomechanical studies and finite element analysis of IPDs have shown a decrease in intradiscal pressure both at the implanted level as well as at adjacent levels, thus avoiding increased biomechanical loads being transmitted to adjacent segments (7-8,10).
The DIAM® (Device for Intervertebral Assisted Motion) spinal stabilization system (Medtronic, Ltd.) is an X-shaped elastomeric IPD. The literature indicates that the DIAM® device was developed by Dr. Jean Taylor (11). In 2007, Taylor et al. suggested three indications for the DIAM® device: “The first indication is for discogenic disease, either primary or recurrent, with or without discectomy. The second indication is for posterior disease resulting in central stenosis, foraminal stenosis, facet disease, or ligamentous instability leading to no more than a Grade I spondylolisthesis. The third indication is to protect from junction disease by implanting a DIAM® above a fresh or existing lumbar fusion.” (11) The thought behind IPDs as dynamic stabilization devices revolves around the biomechanical change of load transmission in the spine with the IPD functioning as a load-sharing device within the spinal column. In addition, by securing the spinous processes of the posterior aspect of the functional spinal unit, they provide distraction of the segment and are meant to reduce extension of the treated segment. In providing distraction of the spinal segment, the literature suggests that these devices may also increase the neuroforaminal space (10,12,13).
There have been multiple investigations into the clinical outcomes associated with the DIAM® device (11,14-19). Taylor et al. conducted a retrospective chart review and follow-up questionnaire for 104 patients who received the DIAM® device during 2001 (11). The pain level showed improvement in 88.5% of the patients (11). Devices are deemed to be efficacious if their use is associated with a significant palliative effect. In this regard, pain levels as measured by visual analog scale (VAS) have been shown to decrease significantly following implantation of DIAM® devices (12,14,18,19). Buric et al. studied 52 consecutive patients implanted with DIAM® devices for low back pain due to degenerative disc disease (14). In their series, 88% of patients were considered as success (defined as a ≥30% of improvement as calculated on the Roland Morris Disability Questionnaire scale comparing the 24 months values to the baseline values) (14). Fabrizi examined a series of 1,315 consecutive patients who underwent DIAM® implantation (15). Using the modified Macnab criteria, symptoms resolution or improvement was rated as excellent or good in 89% of the patients (15). When comparing DIAM® implantation to traditional decompressive surgery, Wu et al. found no significant difference between the groups for low back pain, leg pain, or complications (20). The traditional decompressive group had a significantly lower incidence of reoperation (20). Wu et al. concluded that although patients may obtain some benefits from interspinous spacers implanted through a minimally invasive technique, interspinous spacer use is associated with a higher incidence of reoperation and higher cost (20).
All of these data suggest that the DIAM® device does participate in the biomechanical load share in the spine and can partially unload the intervertebral disc. In nonclinical animal and cadaver studies as well as clinical studies, multiple authors have shown decrease in range of motion of the treated level with the DIAM® device, especially in extension, without significantly increased ranges of motion at adjacent levels. This decreased range of motion at the index level appears to be maintained at least 2 years post-operatively, and the concomitant restriction of motion with unloading of the disc is likely the biomechanical benefit of the device. The literature also shows that the DIAM® device can realign facet joints and increase neuroforaminal space in the post-operative period, although some studies have shown a decrease in posterior disc height and neuroforaminal height as a function of time. Most authors also reported statistically significant long-term decreased pain (VAS) scores and Oswestry Disability Index (ODI) scores associated with implantation of the DIAM® device, with post-operative pain decreasing significantly in the immediate post-operative timeframe (1–2 weeks) and then continuing at the low levels for the duration of the study. When using the tethers as part of the surgical protocol associated with the DIAM® device, the literature supports that the lordotic angle seems to be flattened in the immediate post-operative timeframe (1–2 weeks) although this may not be maintained long term. Despite this, long-term pain (VAS) scores associated with implantation of the DIAM® device are decreased, which would seem to indicate that changes to lordotic angle alone are not associated with patient pain. Based on these studies, it seems that decreased patient pain correlated the best with decreased range of motion. Reporting of adverse events or secondary surgery associated with the DIAM® device varied widely, with some studies reporting little to no incidences and other studies reporting up to 20%.
While the clinical performance of the DIAM® is summarized above, little is known about the host response to this device. It is known that cyclic loading of the spine can cause wear of a dynamic stabilization system, of which DIAM® is an example. None of the existing literature characterizes the particulate debris from these devices. The objective of this study was to evaluate the host response to the DIAM® device based on a series of nine spine explants with a mean post-operative explant time of 35 months. Quantitative histomorphometry was also performed to characterize polymeric particulate debris in periprosthetic tissues.
Methods
The DIAM® device
The DIAM® device is an X-shaped elastomeric interspinous spacer implant made of a polyester [polyethylene terephthalate (PET)] fabric jacket and a silicone (polydimethylsiloxane) core and secured in place with two braided polyester (PET) tethers with titanium crimps. Although used in Europe, the DIAM® device is not currently approved for use in the United States. Outside the United States, the indications for use listed by the device manufacturer include: LSS, herniated and degenerated lumbar disc, facet joint pain syndrome and minor degenerative spondylolisthesis. During the clinical trial in the US, the proposed indication for use of the DIAM device was “for skeletally mature patients that have moderate low back pain (with or without radicular pain) with current episode lasting less than one year in duration secondary to lumbar degenerative disc disease (DDD) at a single symptomatic level from L2-L5”. Figure 1A shows the DIAM® device and tethers.
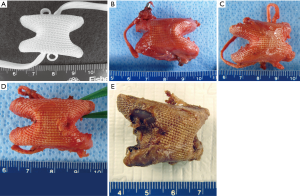
Explant analysis
The primary purpose of our explant study is to evaluate the host response to retrieved spine devices by performing histological analyses on peri-prosthetic tissues. This analysis is covered under the expedited IRB Protocol entitled, “Histologic Evaluation of Retrieved Spine Devices” (HRRC 239-03). Our retrieval analysis study is designed to evaluate the type of tissues adjacent to retrieved devices, look for the presence or absence of wear debris, and characterize the inflammatory response adjacent to the device and debris (if present). Retrieval studies supplement what is learned from the patient’s pathology report, but are not in lieu of pathology (21). Retrieval studies are a unique opportunity to assess the host response to implanted biomaterials.
Over a 10-year period, we received a total of ten accessions from DIAM® patients as summarized in Table 1. The reason for device explantation is also summarized in Table 1. The accession date was used as the identifier on all records, histology slides, and digital images. The DIAM® accessions were from patients in two US clinical trials sponsored by Medtronic Spine (8) or accessions from patients outside the US (2) where the device is approved. Medtronic Spine obtained patient consent for all explant cases. In addition, the principles set forth in the Declaration of Helsinki as well as the US Code of Federal Regulations, Title 45, Part 46, on Protection of Human Subjects were followed.
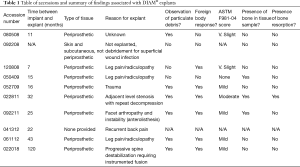
Full table
Of the ten accessions associated with the DIAM® devices, one accession consisted of a wound debridement with skin and subcutaneous tissues without an explanted device and without periprosthetic tissues. In one additional accession, just the device was received without periprosthetic tissues. Therefore, a total of eight accessions with periprosthetic tissues and DIAM® devices ranging between seven and 120 post-operative months were received. The mean time between implantation and explantation of the DIAM® device and periprosthetic tissue samples was 35 months. The determination of the materials response (material properties of the implant material as defined in ASTM F561-05) was not performed in our laboratory (22).
Histologic evaluation
Decalcified/paraffin histological analyses was used to assess the host response to the explanted IPDs. Routine H&E staining was performed. In addition, Oil Red O special staining was performed. Oil Red O staining is frequently used for the demonstration of polymeric particulate debris in periprosthetic tissues associated with joint arthroplasty (23). Additional special staining included Mallory Aniline Blue Connective tissue stain and Wright-Giemsa staining. The degree of inflammatory host response to the device and particulate debris was graded per ASTM F981-04 (24).
Quantitative histomorphometry of particulate debris
In three of the patients in which particulate debris was observed in periprosthetic histology, quantitative histomorphometry of particulate debris was conducting using image analysis software (Image Pro Premier 9.04, Media Cybernetics, Silver Spring, MD, USA). Briefly, spatial calibration was conducted using a micrometer slide at a consistent magnification of 200×. This consistent magnification allowed for sufficient sampling of numerous particles in the periprosthetic histology. Microscopic fields for particle size analysis were not randomly selected. Seven to 11 microscopic fields per explant patient were selected for analysis if they contained large numbers of birefringent polymeric particulate for characterization. Measurements included: minimum caliper width, maximum caliper length, mean caliper length, polygonal area of the particle, particle aspect ratio, particle width, and particle length. Particle ranges (measurement filters) were set between 0.5 and 200 µm for most of these parameters. Particle size and shape measurements were transferred from the image analysis software to Excel where averages and standard deviations were calculated.
Results
Gross appearance
The gross appearance of the explanted DIAM® devices is seen in Figure 1B,C,D,E. Six of the nine explanted DIAM® devices had tears in the fabric jacket, and in two instances, the silicone core was separated from the PET fabric jacket. It is not known if this occurred during explantation of the device, or during service in vivo. When exposed, the polydimethylsiloxane elastomeric core of the device had a purplish discoloration at gross. In two of the nine explanted DIAM® devices, the polydimethylsiloxane elastomer core of the device was fragmented, and in both instances, this was observed as a separation of one of the wings from the core of the silicone device. It is not known if this occurred during explantation or during service in vivo.
Radiographic
The radiographic appearance of the explanted DIAM® devices is seen in Figure 2. Four out of the five explanted DIAM® devices had radiopacity of the fabric jacket consistent with calcification or bone on the fabric jacket at the intersection of the superior and inferior wings, as seen in Figure 2A,B.
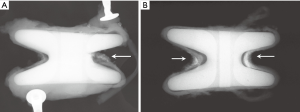
Histologic results
Most of the periprosthetic tissue samples consisted of a mix of connective tissues, with fibrous tissues and bone predominating. At early time points and in some microscopic fields, fibroblasts and fibrous connective tissues surrounded the PET fibers of the fabric jacket of the DIAM® device. As seen in Figure 3A, a foreign body response to the fabric jacket is not observed at seven post-operative months. PET fibers did not stain red with Oil Red O when viewed with transmitted light as seen in Figure 3B. In Figure 3B, red stained silicone particulate debris is observed intracellularly within macrophages and foreign body giant cells at 43 post-operative months. When viewed with polarized light, PET fibers were birefringent as seen in Figure 3C. The red stained silicone particulate was not birefringent when viewed with polarized light, as seen in Figure 3C. Since the silicone used in the DIAM® device is an amorphous (non-crystalline) polymer, and the PET used in the DIAM® device is a crystalline polymer, polarized light microscopy can readily distinguish these two polymers in periprosthetic histology. In addition to the foreign body host response to the PET fibers, a fibrous capsule was observed to surround the PET fibers of the DIAM® device in the histology.
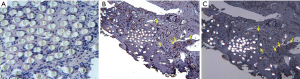
Six of the 8 accessions which included periprosthetic tissue samples showed particulate debris in peri-implant tissues as seen in Table 1. The nonpolarizable silicone particulate debris was surrounded by macrophages as well as foreign body giant cells in the histology. Oil Red O stained sections demonstrated red foamy macrophages with intracellular silicone particulate in periprosthetic tissues as seen in Figure 4A. In Figure 4B, red stained silicone particulate is observed intracellularly within macrophages and foreign body giant cells at 25 post-operative months. As seen in Figure 4B, PET particulate debris was birefringent when viewed with polarized light. The red stained silicone particulate was not birefringent when viewed with polarized light, as seen in Figure 4B. In addition to being found adjacent to the implant, foamy macrophages and foreign body giant cells were also found in intertrabecular spaces as seen in Figure 4C. The same microscopic field from an adjacent serial section viewed with polarized light is seen in Figure 4D, and birefringent PET particulate is observed intracellularly within these foamy macrophages and foreign body giant cells. Despite the presence of this particulate debris, osteoclastic resorption of bone is not observed on adjacent bony trabeculae in Figure 4C.
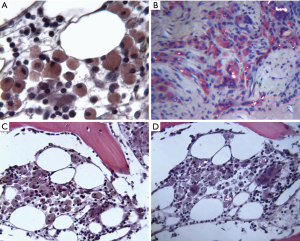
In two of the eight explants with periprosthetic tissues, a foreign body response was not observed in the histology. In six of the eight explants, histology of the periprosthetic tissues demonstrated a non-specific foreign body response. Figure 5A shows a sample micrograph demonstrating a foreign body response to the PET fibers and polymeric debris with several foreign body giant cells at 25 post-operative months. Figure 5B,C show sample micrographs demonstrating a foreign body response at 16 and 120 post-operative months to the non-birefringent silicone debris. The host response was composed of macrophages with foreign body giant cells predominating. As seen in Figure 5D, asteroid bodies within foreign body giant cells was observed in one patient at 32 post-operative months. No plasma cells or neutrophils were observed in the explant histology. Typically, less than five eosinophils and less than five lymphocytes per high power field were observed in periprosthetic tissues. There was no evidence of toxicity, necrosis, device related infection, or an immune response in the histology of periprosthetic tissues. Fluid accumulation was not observed adjacent to the explanted DIAM® devices in the current series of explants. Between 0 to 25 macrophages and between 0 to 15 foreign body giant cells per high power field (400–500×) were observed adjacent to the polymeric device and polymeric particulate debris in peri-prosthetic tissues (24). The ASTM F981-04 scores for inflammation by accession and post-operative time point are seen in Table 1 (24). Per ASTM F981-04, a “very slight” to “mild” to “moderate” chronic inflammatory response was observed to the biomaterials in the explant histology (24).
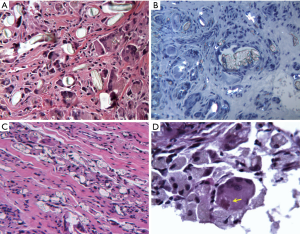
The presence of bony tissue and the presence of bone resorption observed in periprosthetic tissues by explant accession and post-operative time point is seen in Table 1. Three accession contained bone for analysis, and bone resorption (not osteolysis) was noted in only one of these three accessions. As seen in Figure 6A, quiescent bone with hematopoietic marrow in intertrabecular spaces was observed adjacent to the IPD at 25 post-operative months. Osteoclastic resorption of bone was not observed in this explant case. Figure 6B shows bony remodeling consisting of osteoblastic activity (yellow arrows) and a small amount of osteoclastic resorption (green arrows) of bone adjacent to the IPD at 32 post-operative months. Osteolysis was not observed in any of the histology sections from any of the explant accessions.
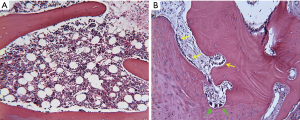
Quantitative histomorphometry of particulate debris results
Approximately 3,000 particles were characterized by quantitative histomorphometry as each of the three accessions had 10 different microscopic fields measured with approximately 100 particles within ranges per microscopic field. Mean measurements of particle size and shape by accession as measured by quantitative histomorphometry as well as mean and standard deviations for all three patients is seen in Table 2. Particle sizes were consistent amongst the three patients with mean particle size on the order of several microns. Similarly, the aspect ratio of particles was consistent amongst the three patients with a mean aspect ratio of 1.98±0.21 for all three patients. The particle sizes and shape were consistent with what was qualitatively observed in the histology as seen in Figures 4,5. Qualitatively, particulate shape of the silicone particles in the histology would be best characterized as globular per ASTM standard F1877-05 [2010] (25). Qualitatively, particulate shape of the polyester particles would be best characterized as threadlike fibers or fibrillar per ASTM standard F1877-05 [2010] (25).
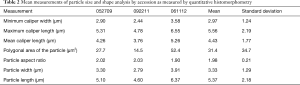
Full table
Discussion
Jerosch and Moursi reported a foreign body reaction (FBR) after implantation of a DIAM® device. They incorrectly attribute the foreign body reaction “due to polyethylene’s wear” even though the DIAM® device is not fabricated from polyethylene (26). Histology revealed particulate debris as well as a FBR with macrophages (26). The patient’s pain resolved after device removal. Seo et al. report a case of FBR to a DIAM® device implanted in a 72-year-old female patient who presented with lower back pain and radiating pain in both legs 5 years after receiving the DIAM® device for spinal stenosis at L4-L5 (27). Removal of the device and granulation tissue with subsequent L3-L5 fusion relieved her back pain (27). The authors conclude, “In patients with a DIAM® who complain of back and radiating pain, a FBR must be considered, and the patients should be monitored long term.” (27). As seen in Table 1, back or leg pain was the reason for DIAM® device explantation in five of our eight explant patients; thus, it is possible that the foreign body reaction was the cause of this pain. Tamburrelli et al. carried out a critical analysis of the causes of failure in a series of 19 patients whose IPDs (5 DIAM® devices in 4 patients) were explanted between 2007 and 2009 (28). With respect to the DIAM® device, Tamburrelli et al. reported, “In one patient (DIAM®), we found a foreign body reaction to the device material. In particular, at surgery, we identified a major local tissue reaction surrounding the device. The polyethylene [sic] lining of the silicone core was broken. The microscopic examination of tissue samples showed the classical features of foreign body reaction.” (28). Histology of the periprosthetic tissues adjacent to the explanted DIAM® devices demonstrated a non-specific foreign body response consisting of macrophages and foreign body giant cells in a majority of our series of explant patients. The chronic inflammatory host response composed of macrophages as well as foreign body giant cells is consistent with the generation of particulate debris by wear mechanisms in spine arthroplasty devices (21,29,30).
According to pathologist James Anderson, “Most biomaterials of potential clinical interest typically elicit the foreign-body reaction (FBR), a special form of non-specific inflammation. The most prominent cells in the FBR are macrophages… Multinucleated giant cells in the vicinity of a foreign body are generally considered evidence of a more severe FBR.” (29). Anderson further states, “Relatively rough surfaces such as those found on the outer surfaces of expanded PTFE or Dacron vascular prostheses have a foreign body reaction composed of macrophages and foreign body giant cells at the surface. Fabric materials generally have a surface response composed of macrophages and foreign body giant cells…” (29). It should be pointed out here that patients may not be aware of any symptoms associated with a FBR to a deeply implanted device, and the observed FBR was not the stated reason for device explantation in any of our series of explant patients as seen in Table 1.
Fluid accumulation was not observed adjacent to the explanted DIAM® devices in the current series of explants. There have been three journal articles that described fluid accumulation associated with DIAM® devices (17,26,31). Two of the three articles were single case reports. The third article mentioned fluid accumulation in a series of patients (17). Out of the thousands of DIAM’s implanted, the reporting of this phenomenon in the literature would appear to be very low. Lastly, from the cardiovascular literature, the incidence of fluid accumulations adjacent to PET vascular grafts is reported in less than 1% of patients (32).
Stefanovic et al. describe a single patient’s two DIAM® implants and report thick aseptic fluid around the DIAM® implants as well as a mineralized pseudocyst around the DIAM® devices and adjacent spinous process osteolysis (31). Laboratory analyses showed that the patient’s sedimentation increased to 47/h, CRP was 11, and there was no change in the patient’s white blood cell count (31). Despite these laboratory tests, osteolysis of the spinous processes might be evidence of infective osteolysis in this case, as it is possible the abscesses could have been caused by an infection. In fact, the clinicians suspected an infection as they treated the patient with 3 courses of antibiotics (31). They indicate that the patient’s “pain subsided” after the course of antibiotics (31). Although both DIAM® devices were explanted, no histopathology of periprosthetic tissues was provided in the paper that could indicate an infection, a FBR, or particulate debris. Particulate debris of any material can show osteolysis. Even though large numbers of DIAM® devices have been implanted, osteolysis associated with the DIAM® device has not been reported in any other cases in the scientific literature. Although not all of our explants included bony tissue samples, none of the periprosthetic tissue samples associated with our series of explants showed evidence of osteolysis.
We found asteroid bodies within foreign body giant cells as part of a foreign body response to polymeric particulate debris in one patient at 32 post-operative months. Asteroid bodies within foreign body giant cells have been reported in the literature for medical devices made from silicone biomaterials (33-39). With respect to asteroid bodies, Lasso et al. indicate, “Although their presence is not specific, they are usually associated with sarcoidosis, tuberculosis, and the reaction of foreign bodies.” (35).
The long-term durability of polyester (PET) as an implantable biomaterial is in question because of degradation and hydrolysis of polyesters that occurs in physiological conditions (40). This degradation is increased under mechanical stresses (40). Both in vivo and in vitro studies have shown that the cumulative effects of such slow degradation processes will ultimately lead to PET vascular implant failure via mechanical breakdown (40-42). With respect to the lifetime of PET for medical applications, Rahman states, “Due to the physical and chemical variability within the PET, it is very difficult to estimate how long PET implants will last in the physiological conditions. One suggestion is that when using PET medical devices for human spare parts, the surgeons must be committed to a follow-up of such a patient for his/her remaining life. Only by this means will the safety and effectiveness of the spare part become known.” (40).
Much of the polymeric particulate debris in the series of DIAM® explants was on the order of several microns in size, but some smaller particles could have been agglomerated or clumped. We used a consistent magnification of 200× to conduct the analysis of particle size. Therefore, particles smaller than 0.5 µm, if present, were not measured by our quantitative histomorphometry technique. While light microscopy is not the best tool for evaluating particle size and shape, this method nevertheless provided a good estimate of particle size, particle area, and aspect ratio. The particle sizes and shape as measured by quantitative histology were consistent with what was qualitatively observed in the histology as seen in Figures 4,5. Therefore, particle characterization and measurements for mean particle size, aspect ratio, and particle area were reported with these discussed limitations in mind.
Conclusions
Six of the nine explanted DIAM® devices had tears in the fabric jacket, and in two instances, the silicone core was separated from the PET fabric jacket. In two of the nine explanted DIAM® devices, the polydimethylsiloxane elastomer core of the device was fragmented, and in both instances, this was observed as a separation of one of the wings from the core of the silicone device. It is not known if these tears and fractures occurred during explantation or during service in vivo. Four out of the five explanted DIAM® devices had radiopacity of the fabric jacket consistent with calcification or bone on the fabric jacket at the intersection of the superior and inferior wings. Long-term integrity of the fabric jacket may be in question as PET can undergo degradation by hydrolysis which can be accelerated by mechanical stresses. Similarly, the silicone core component may fracture after numerous cycles of loading.
Histology of the periprosthetic tissues adjacent to the explanted DIAM® devices demonstrated a non-specific foreign body response consisting of macrophages and foreign body giant cells in most of our series of explant patients. The foreign body reaction consisting of macrophages and foreign body giant cells is most likely associated with: (I) the fabric nature of the polyester jacket of the DIAM® device, and (II) the generation of particulate debris by wear mechanisms. Back or leg pain was the reason for DIAM® device explantation in 5 of our 8 explant patients; thus, it is possible that the foreign body reaction was the cause of this pain. However, the literature suggest that patients may not be aware of symptoms associated with a foreign body reaction to a deeply implanted device, and in our series of explants, the observed foreign body reaction was not the stated reason for device explantation. Nevertheless, surgeons should be aware of the host response to this device as it may be a possible source of pain.
Cyclic loading of the spine can cause the generation of wear debris in dynamic stabilization systems such as DIAM®. Particle sizes were consistent amongst the explant patients with mean particle size on the order of several microns. The particle sizes and shape were consistent with what was qualitatively observed in the histology. None of the periprosthetic tissue samples showed evidence of osteolysis in our series of explants.
Acknowledgments
The authors wish to thank and acknowledge Tatunya Buford, HT(ASCP), Amy Rizzo, MT(ASCP), and Sharath Chedella, BDS, MS, for assistance with histologic sectioning and special staining.Institutional Research support for this study was provided by Medtronic Spine. Active participation in the work was not undertaken by members of this funding source.
Footnote
Conflicts of Interest: JM Toth serves as scientific consultant to Medtronic but has received no remuneration in the past 2 years. JD Bric has no conflicts of interest to declare.
Ethical Statement: The authors are accountable for all aspects of the work in ensuring that questions related to the accuracy or integrity of any part of the work are appropriately investigated and resolved. Medtronic Spine obtained patient consent for all explant cases. In addition, the principles set forth in the Declaration of Helsinki as well as the US Code of Federal Regulations, Title 45, Part 46, on Protection of Human Subjects were followed.
References
- Kalichman L, Cole R, Kim D, et al. Spinal stenosis prevalence and association with symptoms: the Framingham Study. Spine J 2009;9:545-50. [Crossref] [PubMed]
- Deyo RA, Gray DT, Kreuter W, et al. United States trends in lumbar surgery for degenerative conditions. Spine (Phila Pa 1976) 2005;30:1441-5. [Crossref] [PubMed]
- Weinstein JN, Tosteson TD, Lurie JD, et al. Surgical versus non-operative treatment for lumbar spinal stenosis four-year results of the spine patient outcomes research trial (SPORT). Spine (Phila Pa 1976) 2010;35:1329-38. [Crossref] [PubMed]
- Lee SH, Seol A, Cho TY, et al. A systematic review of interspinous dynamic stabilization. Clin Orthop Surg 2015;7:323-9. [Crossref] [PubMed]
- Anasetti F, Galbusera F, Aziz HN, et al. Spine stability after implantation of an interspinous device: an in vitro and finite element biomechanical study. J Neurosurg Spine 2010;13:568-75. [Crossref] [PubMed]
- Bellini CM, Galbusera F, Raimondi MT, et al. Biomechanics of the lumbar spine after dynamic stabilization. J Spinal Disord Tech 2007;20:423-9. [Crossref] [PubMed]
- Hirsch C, Breque C, Ragot S, et al. Biomechanical study of dynamic changes in L4-L5 foramen surface area in flexion and extension after implantation of four interspinous process devices. Orthop Traumatol Surg Res 2015;101:215-9. [Crossref] [PubMed]
- Lee CH, Kim YE, Lee HJ, et al. Biomechanical effects of hybrid stabilization on the risk of proximal adjacent-segment degeneration following lumbar spinal fusion using an interspinous device or a pedicle screw based dynamic fixator. J Neurosurg Spine 2017;27:643-9. [Crossref] [PubMed]
- Phillips FM, Voronov LI, Gaitanis IN, et al. Biomechanics of posterior dynamic stabilizing device (DIAM) after facetectomy and discectomy. Spine J 2006;6:714-22. [Crossref] [PubMed]
- Wilke HJ, Drumm J, Häussler K, et al. Biomechanical effect of different lumbar interspinous implants on flexibility and intradiscal pressure. Eur Spine J 2008;17:1049-56. [Crossref] [PubMed]
- Taylor J, Pupin P, Delajoux S, et al. Device of intervertebral assisted motion: technique and initial results. Neurosurg Focus 2007;22:E6. [Crossref] [PubMed]
- Lee CH, Hyun SJ, Kim KJ, et al. The efficacy of lumbar hybrid stabilization using the DIAM® to delay adjacent segment degeneration: an intervention comparison study with a minimum 2-year follow-up. Neurosurgery 2013;73:ons224-31; discussion ons231-2.
- Lin JF, Tsai CC. Surgical role of interspinous distraction devices (Coflex, DIAM) for the treatment of neurologic pain and intermittent claudication due to lumbar spinal stenosis. Int J Gerontol 2013;7:226-30. [Crossref]
- Buric J, Pulidori M, Sinan T, et al. DIAM® device for low back pain in degenerative disc disease: 24 months follow-up. Acta Neurochir Suppl 2011;108:177-82. [Crossref] [PubMed]
- Fabrizi AP, Maina R, Schiabello L. Interspinous spacers in the treatment of degenerative lumbar spinal disease: our experience with DIAM® and Aperius devices. Eur Spine J 2011;20:S20-6. [Crossref] [PubMed]
- Gazzeri R, Galarza M, Alfieri A. Controversies about interspinous process devices in the treatment of degenerative lumbar spine diseases: past, present, and future. Biomed Res Int 2014;2014:975052. [PubMed]
- Gazzeri R, Galarza M, Neroni M, et al. Failure rates and complications of interspinous process decompression devices: a European multicenter study. Neurosurg Focus 2015;39:E14. [Crossref] [PubMed]
- Ha KY, Seo JY, Kwon SE, et al. Posterior dynamic stabilization in the treatment of degenerative lumbar stenosis: validity of its rationale. J Neurosurg Spine 2013;18:24-31. [Crossref] [PubMed]
- Kim KA, McDonald M, Pik JH, et al. Dynamic intraspinous spacer technology for posterior stabilization: case-control study on the safety, sagittal angulation, and pain outcome at 1-year follow-up evaluation. Neurosurg Focus 2007;22:E7. [Crossref] [PubMed]
- Wu AM, Zhou Y, Li QL, et al. Interspinous spacer versus traditional decompressive surgery for lumbar spinal stenosis: a systematic review and meta-analysis. PLoS One 2014;9:e97142. [Crossref] [PubMed]
- Anderson PA, Kurtz S, Toth JM. Explant analysis of total disc replacement. Semin Spine Surg 2006;18:109-16. [Crossref]
- ASTM Standard Practice F561-13. Standard Practice for Retrieval and Analysis of Medical Devices, and Associated Tissues and Fluids. West Conshohocken, PA: ASTM International, Vol 13.01, 2014:170-89.
- Schmalzried TP, Jasty M, Rosenberg A, et al. Histologic identification of polyethylene wear debris using Oil Red O stain. J Appl Biomater 1993;4:119-25. [Crossref] [PubMed]
- ASTM Standard Practice F981-04 (Reapproved 2010). Standard Practice for Assessment of Compatibility of Biomaterials for Surgical Implants with Respect to Effect of Materials on Muscle and Bone. West Conshohocken, PA: ASTM International, Vol 13.01, 2014:396-400.
- ASTM Standard Practice F1877-05 (Reapproved 2010). Standard Practice for Characterization of Particles. West Conshohocken, PA: ASTM International, Vol 13.01, 2014:797-811.
- Jerosch J, Moursi MG. Foreign body reaction due to polyethylene's wear after implantation of an interspinal segment. Arch Orthop Trauma Surg 2008;128:1-4. [Crossref] [PubMed]
- Seo JY, Ha KY, Kim YH, et al. Foreign body reaction after implantation of a device for intervertebral assisted motion. J Korean Neurosurg Soc 2016;59:647-9. [Crossref] [PubMed]
- Tamburrelli FC, Proietti L, Logroscino CA. Critical analysis of lumbar interspinous devices failures: a retrospective study. Eur Spine J 2011;20:S27-35. [Crossref] [PubMed]
- Anderson JM, Cook G, Costerton B, et al. Host reactions to biomaterials and their evaluation. In: Ratner BD, Hoffman AS, Schoen FJ, et al. editors. Biomaterials Science: An Introduction to Materials in Medicine. 2nd edition. New York: Elsevier Academic Press, 2004:293-304.
- Anderson PA, Rouleau JP, Toth JM, et al. A comparison of simulator tested and retrieved cervical disc prostheses. J Neurosurg Spine 2004;1:202-10. [Crossref] [PubMed]
- Stefanovic I, Radisavljevic M, Stojanov D. Aseptic intrafascial and extrafascial abscesses 10 years after a 2-level DIAM® insertion procedure. J Neurosurg Spine 2015;23:647-51. [Crossref] [PubMed]
- Vollmar JF, Guldner NW, Mohr W, et al. Perigraft-reaction after implantation of vascular prostheses. pathogenesis, clinical picture and treatment. Int Angiol 1987;6:287-93. [PubMed]
- Balco MT, Ali SZ. Asteroid bodies in silicone lymphadenitis on fine-needle aspiration. Diagn Cytopathol 2007;35:715-6. [Crossref] [PubMed]
- Kircher T. Silicone lymphadenopathy: a complication of silicone elastomer finger joint prostheses. Hum Pathol 1980;11:240-4. [Crossref] [PubMed]
- Lasso JM, Toledo G, Idoate MA, et al. Asteroid bodies in a patient with oil breast implants. Plast Reconstr Surg 2001;108:1832-3. [Crossref] [PubMed]
- Lemperle G, Morhenn V, Charrier U. Human histology and persistence of various injectable filler substances for soft tissue augmentation. Aesthetic Plast Surg 2003;27:354-66. [Crossref] [PubMed]
- Murakata LA, Rangwala AF. Silicone lymphadenopathy with concomitant malignant lymphoma. J Rheumatol 1989;16:1480-3. [PubMed]
- Péoc’h M, Duprez D, Grice G, et al. Silicone lymphadenopathy mimicking a lymphoma in a patient with a metatarsophalangeal joint prosthesis. J Clin Pathol 2000;53:549-51. [Crossref] [PubMed]
- van Diest PJ, Beekman WH, Hage JJ. Pathology of silicone leakage from breast implants. J Clin Pathol 1998;51:493-7. [Crossref] [PubMed]
- Rahman M. Degradation of Polyesters in Medical Applications. doi: 10.5772/47765, 2012. Available online: https://www.intechopen.com/books/polyester/degradation-of-polyesters-in-medical-applications (Accessed April 18, 2019). [Crossref]
- Riepe G, Loos J, Imig H, et al. Long-term in vivo alterations of polyester vascular grafts in humans. Eur J Vasc Endovasc Surg 1997;13:540-8. [Crossref] [PubMed]
- Zaikov GE. Quantitative aspects of polymer degradation in the living body. JMS-Rev Macromol Chem Phys 1985;C25:551-97. [Crossref]