Virtual reality in spinal endoscopy: a paradigm shift in education to support spine surgeons
Introduction
Simulation by means of virtual reality (VR) in neurosurgery and orthopaedic surgery for educational, preoperative planning, and intra-operative utilization continues to improve with technological advances in computer processing. Simulation is endorsed by numerous organizations including the American College of Surgeons, American Academy of Orthopaedic Surgeons, Authorité Hauté Santé in France, Congress of Neurological Surgeons, and Accreditation Council for Graduate Medical Education (ACGME) (1). Simulators for educational purposes continue to develop along the six core competencies laid by the ACGME to replicate clinical training scenarios in the face of flexible duty hours, heterogeneity of experience, and changing resident education. The number of publications dedicated to simulation in the fields of neurosurgery and orthopaedics has continued to dramatically rise in the preceding decade, with transition from bench top and low fidelity models to contemporary VR (2). VR utilizes a computer processing unit with a head-mounted display (HMD) to provide visual and auditory cues coupled with controllers containing position trackers and force feedback, or haptics, to provide an immersive, multisensory experience.
Additional areas of simulation include augmented reality (AR) or mixed reality (MR). AR combines computer processing and a see-through display that projects a virtual construct onto real-world imagery. VR and AR exist on a continuous spectrum of MR, from the completely digitized and simulated environment present in VR to real and simulated environments of AR, and combinations between referred to as MR. VR in spine surgery has seen greatest use in educational simulation or pre-operative planning (3). Spinal anatomy is complex and variable, and the ability to visualize and interact with a virtual patient’s spine prior to surgical intervention has benefit in rehearsal and planning. The focus of AR in spine surgery has been for intraoperative visualization and instrumentation (4). Most of these technologies rely on software capable of receiving patient CT scans of adequate resolution (approximately 1.25-mm slice width or smaller) and producing interactive volume renderings in a three-dimensional (3D) environment. VR for educational purposes or pre-operative planning in spine surgery and orthopaedic surgery has lagged behind other surgical subspecialties as well as commercial industry, with multiple reviews delineating the lack of standardized measures, low levels of evidence and levels of recommendation despite consensus statements from working directives and organizations (5-8). Furthermore, cost-effectiveness of these VR constructs has not been conclusively demonstrated in spine surgery, though has been alluded to in other areas of orthopaedic surgery.
Paralleling the rise in popularity of VR is minimally invasive spine surgery (MISS) and endoscopic spine surgery for treatment of spinal stenosis, degenerative disk disease, compression fractures, tumor or lesion ablation, and most recently for adult spinal deformity (ASD) correction (9). In a period of 1997 to 2017, the number of articles relating to endoscopic spine surgery increased 41 times (10). The rate of MISS procedures is approaching that of open procedures given increasing surgeon familiarity and potential benefits including faster recovery, reduced blood loss, shorter hospital stays, and outpatient settings (10,11). Endoscopic spine surgery requires correct localization, cannulation, and continuous irrigation while directly visualizing structures. Depending on the region of pathology, various portal techniques or endoscope docking and free-hand techniques may be employed (12). Endoscopic techniques require significant surgeon skill, and learning curves have been described as steep (13). Multi-centre, multi-year studies of percutaneous endoscopic lumbar discectomy (PELD) demonstrate continuous improvements in surgical time (14). Complication rates from dural tear, infection, epidural hematoma, and dysesthesias have been described as increased in early periods of endoscopic surgical adoption (15-17). Similarly, the pedicle screw placement learning curve has been estimated at 80 screws, or 25 cases to reach an asymptote of technique skill (18). Fluoroscopic time and radiation exposure are significantly higher with novice surgeons (10).
Further advances in technology with increasing evidence of MISS and endoscopic spine surgical benefit will promote incorporation of these procedures into regular practice. Despite the use of more technological instrumentation, surgeon recognition of the importance of clinical outcomes and patient safety is paramount. VR, AR, and MR simulators stand to provide uninhibited practice of surgical techniques for training purposes, and may benefit surgeons clinically for preoperative planning, and intraoperative use. Ultimately, VR, AR, and MR simulators could improve adoption of endoscopic spine surgery by traditionally trained spine surgeons, who often struggle with the adoption of spinal endoscopy due to its technical difficulties and expensive non-portable stand-alone simulators. Therefore, we sought to determine the current evidence of the use of VR, AR, and MR simulators in MISS and spinal endoscopic surgery, including study quality, level of evidence (LoE), and outcomes. Furthermore, we wished to include recommendations for future studies, and considerations for forthcoming research and simulator development.
Methods
A systematic review of current available literature pertaining to VR and endoscopic/MISS was performed according to the guidelines for reporting systematic reviews (PRISMA).
Search strategy
The search strategy incorporated databases Medline OVID, Embase, PsychINFO, Cochrane reviews, and Google Scholar. Search strategy was completed in stages and combined using Boolean operators. Stage 1 incorporated terms relating to VR, MR or AR or computer-assisted surgery. Stage 2 incorporated educational terms or simulation terms. Stage 3 incorporated terms relating to neurosurgery or spine surgery. Stage 4 incorporated terms for minimally invasive, percutaneous, or endoscopic surgery. Lastly, Stage 5 incorporated all stage terms so that only articles containing these subjects were retained for initial review. Terms used included Medical Subject Headings (MeSH) for comprehensiveness. Secondary searches were conducted specifically for known commercially available VR suites including “PrecisionOS Technology”, “PHANToM”, “Dextroscope”, “Surgical Rehearsal Platform”, “Falcon”, “Procedicus VIST Simulator”, “ImmersiveTouch”, and “Perk Tutor”.
Inclusion criteria included English language and studies with primary data reporting of educational or simulation in minimally invasive/endoscopic/percutaneous spinal surgery with measurable outcomes. All relevant empirical study designs were included including randomized controlled trials, non-randomized controlled trials, prospective cohort studies, retrospective studies or cross-sectional studies. All percutaneous/endoscopic/minimally invasive manual surgical spine procedures were included including discectomy, fusion, foraminotomy and nerve decompression, and vertebroplasty. Pedicle screw insertion studies were included. Studies included required mention of preoperative planning or for educational purposes using VR/AR/MR simulators, or for intraoperative use. Incorporated participants in studies included all levels of training and all simulation devices pertaining to VR, MR, or AR. Exclusion criteria included neurosurgical studies incorporating brain or soft tissue surgery simulators, non-manual studies such as radiotherapy application, non-MISS studies, or studies not incorporating VR, AR, or MR.
Following initial search, title screening was performed by a single study member (R Lohre) for appropriateness of inclusion. Additional records identified through other sources were added to the initial search pool. Duplicates were screened and removed prior to abstract review. Abstract review was then performed by a single study member (R Lohre). Full articles retained were reviewed by study members (R Lohre and DP Goel) and any discrepancy on inclusion resolved by consensus. After full text review, the studies incorporated were searched for additional references manually. PubMed search term notifications were set up, as well as through the Mendeley platform for any new articles published during the manuscript draft period up to time of submission (November 2019).
Data extraction
Data extraction was performed by a single study member (R Goel). Study demographic data including authors, location of study, publication, simulated MISS procedure, simulator type and brand if available were extracted. Next, study parameters were extracted including type of study, aims or hypothesis, participants, validity assessments if any, outcome measures and main conclusions. Retained studies were examined for methodological quality using the validated Medical Education Research Study Quality Instrument (MERSQI). Scoring was provided via the MERSQI range of 5–18, with higher scores denoting higher quality study design, sampling, data type, assessment, validity measurements, data analysis and outcome measures and reporting. If assessments of each characteristic were not possible for a study, scores remained in keeping with standard MERSQI reporting with a highest value of 18. MERSQI scores reported are of the primary authors interpretation. LoE was provided for each study and was gathered either through publication reporting, or determined by study member (R Lohre) based on the modified Oxford Centre for Evidence-Based Medicine (OCEBM) guidelines proposed by Carter et al. for VR surgical simulators (19). A meta-analysis was not performed given the heterogeneity in study design, outcome measures and reporting variability.
Results
Following PRISMA guidelines, 2,287 unique publications were screened to produce 57 for full text review. Nineteen articles were further excluded prior to qualitative synthesis. Articles were excluded for not involving VR, AR, or MR (n=7), incorporating open procedures rather than MISS (n=5), incorporating other soft tissue structures such as brain (n=4), or not providing outcomes (n=3). Thirty-eight studies were included in the review, while a meta-analysis was not performed due to heterogeneity of study designs and reported outcomes. Figure 1 depicts the screening and selection process for the systematic review.
Table 1 depicts characteristics of representative included studies including journal of publication, location of experiment, type of MISS or pedicle insertion procedure simulated, spectrum of simulator type, brand names of simulators and availability if described, and MERSQI and LoE scoring. For a full list of studies, please see Tables S1,S2. The earliest study was seen to occur in 2009 (33). Though no publication year cut-off was used during searching, the majority of articles (n=20; 52.6%) were published within 2 years prior to time of review writing. Eleven studies (28.9%) were conducted in China, 7 (18.4%) in the USA, 7 (18.4%) in Germany, 4 (10.5%) in Canada, 3 (7.9%) in Sweden, 2 (5.3%) in the UK, 2 (5.3%) in Japan, 1 (2.6%) in Finland, and 1 (2.6%) in Italy. Ten (90.9%) of the articles published in China were within 2 years of review writing. Fourteen (36.8%) of the studies specified VR simulators, 11 (28.9%) utilized AR simulators, and 10 (26.3%) utilized a combination of components designating MR. The majority (n=8; 57.1%) of VR simulators were unspecified, or of proprietary design and not commercially available. Other VR simulators used included Simulation and Visualization Research Group (n=1; 7.1%), NeurosimVR (Calgary, AB, Canada) (n=1; 7.1%), ImmersiveTouch (San Francisco, CA, USA) (n=2; 14.3%), and Boholo (Fengsuan Inc., Shanghai, China) (n=1; 7.1%). Three (27.3%) of the AR simulators used were unspecified. Two (18.2%) studies utilized Microsoft HoloLens (Redmond, WA, USA) for AR visualization and proprietary software, 2 (18.2%) utilized ImmersiveTouch (San Francisco, CA, USA), 1 utilized Virtual Protractor with Augmented Reality (VIPAR) though is not commercially available, one utilized Medtronic StealthStation, one utilized Perk Station (The Perk Lab, Queen’s University, Canada), and one utilized a proprietary tracking system using Micron Tracker2 and a graphics user interface. Studies utilizing MR were varied in utilized simulators, with 2 (20.0%) not specifying simulators used. The most frequently used MR simulator was that of the Novint Falcon (Novint Technologis, Inc., USA), utilized in 3 (30.0%) of MR studies.
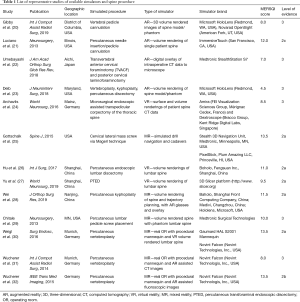
Full table
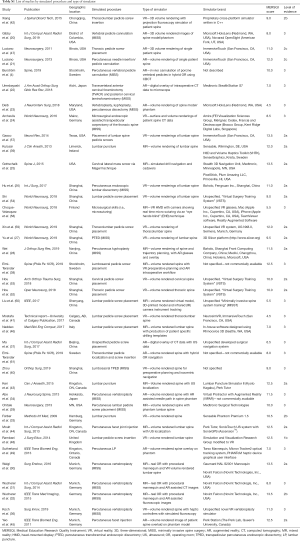
Full table
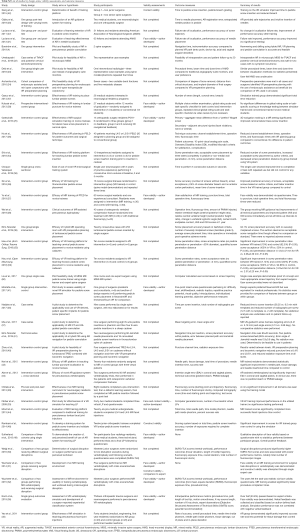
Full table
Pedicle screw insertion and cannulation for percutaneous approaches were both included in this review. Eighteen (47.4%) of studies involved localization of pedicles, and cannulation or insertion of pedicle screws, in general. Of these studies, the predominant location was that of lumbar vertebrae, seen in 10 (55.6%) studies. Two (11.1%) pedicle screw insertion studies involved the thoracic spine (24,34), 1 (5.6%) study involved cervical pedicle screw insertion (35), 1 (5.6%) involved cervical lateral mass screw insertion (36), 1 (5.6%) involved thoracolumbar pedicle screw insertion (27), and 1 (5.6%) was not specified and utilized a sheep cadaver (37). Seven studies (18.4%) pertained to vertebroplasty or kyphoplasty simulation (20,27,29,31,32,38,39). Two (5.3%) studies utilized simulators for transpedicular percutaneous endoscopic discectomy (TPED) (40). One (2.6%) study examined both vertebroplasty/kyphoplasty and discectomy using an AR system (27). Seven (18.4%) studies examined simulator use for needle localization of lumbar puncture (LP) or facet injections (25,33,41-45), with five studies utilizing AR or MR, and a single using a VR system. One (2.6%) study examined an AR simulator for use in transvertebral anterior cervical foraminotomy (TVACF) and posterior cervical laminoforaminotomy (30). Another single study (2.6%) examined the use of VR in microsurgical endoscopic assisted transpedicular corpectomy of the thoracic spine (26).
Table 2 illustrates design, hypotheses, aims, and outcomes of retained studies. Seven (18.4%) studies involved use of simulators in patients (22,24,26,28,34,38,46). A total composite of patients involved in VR/AR/MR trials was n=123. The longest series of follow-up was to 20 months following a case series of two patients receiving TVACF and posterior cervical laminoforaminotomy (22). Follow-up was regarding symptom recurrence without validated patient outcome metrics. Two studies presented patient interval follow-up with recording of patient outcome metrics including Visual Analog Scale (VAS), Oswestry Disability Index (ODI), and modified McNab criteria (26,28). One of the aforementioned studies pertained to percutaneous lumbar discectomy procedure (PLED) of L4/5 and L5/S1 in 40 patients using a VR system for preoperative planning. The use of VR planning showed reduced technical times with equivalent patient outcomes at 6-month follow-up (26). The other aforementioned study pertained to MR use during percutaneous kyphoplasty. Forty patients similarly received randomization and treatment, with the MR group showing improvement in all technical parameters [operating time, fluoroscopy time, polymethylmethacrylate (PMMA) used, anterior/posterior (A/P) height ratios, kyphotic angle (KA) change, cement-both-endplates-contact, as well as VAS and ODI scores immediately post-op and at follow-up intervals to 1 year] (28).
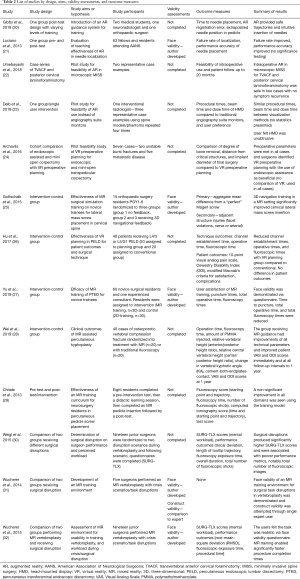
Full table
Study aims or hypotheses varied considerably. Broad categories included determination of effectiveness of simulator platforms for training use, a pilot study for use in training or clinical scenarios, and clinical effectiveness and intervention studies. Twenty-four (63.2%) studies involved determination of simulator effectiveness in training, with 11 (45.8%) of these studies utilizing VR training systems, 8 (33.3%) utilizing MR training systems, and 5 (20.8%) studies utilizing AR training systems. Eleven (28.9%) studies specified pilot studies in introduction of VR/AR/MR systems for training or clinical use. Three (7.9%) studies specifically sought to compare clinical effectiveness of VR/AR/MR. Twenty-three (60.5%) studies were performed using an intervention-control design. These studies compared the use of VR (n=12, 52.2%), AR (n=3, 13.0%), and MR (n=8, 34.8%) use in preoperative planning and intra-operative use relative to established standards of training. Three intervention-control studies utilized disruption scenarios in an MR simulator operating room (OR) and did not specifically compare MR to other simulation methods (30-32). Four studies (10.5%) utilized a pre- and post-test design scheme (20,21,29,47)), three of which utilized AR systems (20,21,47), and one MR system (29). The mean number of study participants in all studies was 16.4 (SD =17.5; range, 1–63). Study participants ranged from design technicians, undergraduate students, medical students, residents, orthopaedic surgeons, and neurosurgeons.
Twelve studies (31.6%) (21,25,27,31-33,36,39,41,42,48,49) attempted to establish validity in simulator use. Validity measures included demonstration of face, construct, and content validity. There were no studies that demonstrated transfer validity of educational or training simulators of VR, AR, or MR design to real OR scenarios. Ten of the 12 studies (83.3%) demonstrated face validity of the simulators via non-validated questionnaire responses of varying number of questions and Likert-responses (21,25,27,31-33,36,39,41,49). Two of the 10 studies also examined construct and content validity (31,33). One study examined the construct validity of a VR simulator for pedicle screw insertion, while another examined content validity of a VR simulator for pedicle screw insertion (42,48). All studies examining face validity demonstrated positive outcomes regarding realism of the system used compared to the simulated task. Three studies examined handling and functionality of simulator systems via non-validated questionnaires (32,33,49).
Quality of included studies was examined using the MERSQI score and LoE. There was a variation in MERSQI scores in included studies, with an average M =9.71 (SD =2.60; range, 4.5–13.5) out of 18. Individual MERSQI domains of “sampling” M =0.59 (SD =0.25; range, 0.5–1) and “validity” M=0.65 (SD =1.03; range, 0–3) had the lowest mean scores. Comparison of individual MERSQI domains is limited given variability in scale ranges and study characteristics. The LoE reported was based on Carter et al. modification of OCEBM guidelines, published by the Work Group for Evaluation and Implementation of Simulators and Skills Training Programmes, European Association of Endoscopic Surgeons (EAES). Eighteen (47.4%) studies achieved an LoE of 3 based on nonrandomized, noncomparative, descriptive trials. Fourteen (36.8%) studies were designated a LoE of 2a, for providing reasonable quality randomized trials, though that did not meet or provide sample size calculations. One (2.6%) article achieved an LoE of 1b, and was the highest LoE study included in the review for providing an adequate powered, randomized trial (48). A correlation of MERSQI score to LoE was not completed for included studies.
Discussion
The use of simulators in orthopaedic surgery training has continued to increase with support of regulatory bodies and mounting evidence of knowledge retention and skill improvement. MISS and spinal endoscopic surgery have particular reliance on complicated trajectories and uncoupling of hand-eye cues similar to that of arthroscopic procedures. Reports of high complication rates in early adoption may adversely steer established surgeons from performing these procedures. As evidence grows for simulation in endoscopic surgical training, production of simulators that are portable, cost-effective, and enjoyable to use cannot be underscored. Our systematic review highlighted 38 studies involving VR, AR, and MR simulation in MISS including pedicle screw insertion. These studies were performed globally and were relatively equivalent in terms of volume of VR, AR, or MR system used. There was a clear lack of commercially available simulators, with the majority being developed using proprietary instrumentation or software.
Intervention-control studies were predominantly utilized for VR, AR and MR simulator studies. In these studies, the digital simulator trained groups routinely outperformed the control groups in measured parameters except in one (39). Though a meta-analysis could not be performed to aggregate data, descriptive analysis demonstrates that simulator training improved both knowledge and technical skill of learners, including novice and expert (orthopaedic and neurosurgeons) populations. This was gathered through objective outcomes analysis of user error rates, improvement of technical skills, time to completion of tasks, or fluoroscopic usage.
Additional identified research presented pilot studies, cross-sectional studies, or clinical outcome studies for the use of VR, AR, and MR on patients. Two studies, incorporating follow-up of a total of 80 patients at 6 months and 1 year following VR and MR use in planning and implementation of PLED and kyphoplasty demonstrated superiority in the VR/MR groups over controls (26,28). There were no intraoperative or immediate complications of any patient highlighted in the clinical use publications. Currently there are few publications pertaining to the use of VR, AR, and MR clinically for MISS and spinal endoscopy and evidence remains limited, however as more centres become familiar with available technology, the number of clinical outcome studies is likely to rise. Future studies should attempt standardization of clinical outcomes, supporting well-conducted randomized trials of VR, AR, and MR use in spinal endoscopy and MISS. These outcomes should combine radiographic parameters with patient-reported outcome measures at regular intervals with minimum follow-up of 2 years. Standardized reporting of these trials is also encouraged to allow for direct comparison to other studies in systematic reviews and meta-analyses as research continues.
Overall, study quality was demonstrated as low-to-medium based on MERSQI scores. The mean score was lower than other reviews attempting the same scoring system, and may be attributed to scope of review and inclusion criteria. Our study highlights a greater number of papers than other reviews, and is novel in presenting simulator application for MISS surgery. Furthermore, LoE was determined for all included papers based on a modified OCEBM framework. Overall LoE was low based on descriptive studies. Most intervention-control study designs were of moderate quality based on this modified framework. Studies presented had a number of objective limitations including small population sizes, lack of clearly defined outcomes, absent statistical analyses, limited validity breadth including demonstration of transfer validity, and lack of clinical application. As is the case in other areas of orthopaedics, VR, AR, and MR simulators in MISS education have not demonstrated clear transfer validity to real OR environments. Studies in other surgical disciplines have employed robust study design to accomplish this, and it is likely that this will be attempted in MISS given the surge in recent publications and simulator availability. Moving forward, consensus documents should be produced and adhered to for development and validation of MISS immersive simulators. A standardized framework would be effective in providing clear research protocols to multiple centres, precipitating larger and better-quality studies. For intervention-control, and randomized trials comparing simulator modalities, we advocate for attempting to produce higher quality trials with power and sample size calculations and well-defined hypotheses. The outcomes should be supported by appropriate descriptive statistics. Study designs should allow for prospective, long-term follow-up of skill retention, in multiple levels of trainee or established expert, to establish efficacy of training. As validity of simulators in MISS is lacking, we also recommend adherence to guidelines of establishing face, content, construct, and transfer validity. Transfer validity to real operative scenarios would definitively establish simulator training effectiveness in MISS and spinal endoscopy (50). Finally, reporting requires standardization to allow for aggregate data collection to provide higher levels of evidence and support meta-analyses and we recommend the recently updated reporting guidelines for health care simulation research (51).
There are a number of identifiable limitations of the presented review. First, only English articles were collected and presented. There is an inherent bias to published outcomes of simulators presented in this article, with no identified negative outcomes of simulator use, and only one equivalency study presented (39). Further sources of unaccounted for bias include financial disclosures and vested interest in simulator products by authors, and reporting of multiple experiments with the same group of learners. These articles were included in the review as they presented different data or aspects of the experiment, however impart bias with experimental repetition and thus affected MERSQI scoring (30,31,52-54). Following search strategy, data aggregation and scoring via MERSQI and LoE was performed by a single reviewer, thus potentially incorporating bias and preventing inter-rater reliability calculations. Evaluation of study quality with MERSQI has previously been used in spine simulation studies, however has inconsistencies based on reviewer and interpretation of criteria, thus our use of a single reviewer potentially expounds these inconsistencies in reporting (8). Additional use of the Newcastle-Ottawa Scale-Education (NOS-E) may be considered in future analyses as this has been validated previously (35).
Beyond novice surgeons, tracking of user data, individualization and updating cases, and ability to perform surgeries virtually prior to a real environment stand to benefit practicing and expert surgeons. Regarding development of simulators, efforts should be made to produce multi-use, adaptable designs to replicate real world OR scenarios in spinal endoscopy and MISS. Adaptability in software modules, hardware, and control schemes will allow for the growing indication of spinal endoscopic procedures and allow for growth within the specialty. New avenues of research with VR, AR, and MR systems include collection of large data with artificial intelligence applications to develop expert sourced recommendations for trajectory of implants or implant types. This may be adaptable to endoscopic approach, pedicle trajectories, or osteotomy for deformity correction.
Sample surgical training workflow
We provide a sample iterative workflow for established spine surgeons wishing to train in contemporary endoscopic spine surgery. PrecisionOS Technology™ as a representative example, provides an entirely immersive, multi-sensory OR environment for training. Figure 2 provides images of the virtual suite once the surgeon places the heads up display (HUD) on themselves. Complementing the sensory experience is ability to practice both clinical decision-making and real, technical skill on representative stock cases, or import additional cases (available in 2020). Computing software allows for tracking of progress through stock cases, both in error and success rates, and technical outcomes utilizing a well-known concept in education referred to as deliberate practice (55).
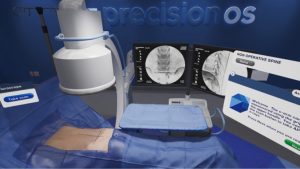
A surgeon using the HUD and haptic controllers would select one of several representative cases appropriately indicated for endoscopic surgery and subsequently perform this case in immersive VR (iVR). The equipment is free of wires and can be used in any environment. Uninhibited repetition with variation, also referred to as interleaving is one of several paramount learning nuances created in this technology (56). The degrees of freedom of the techniques, ability to fail and a guide-mode with direct feedback is an available option to aid in early learning, which may be turned on, or off as experience grows. Akin to other avenues of training in orthopaedics or neurosurgery, or high-risk industries such as aviation, the surgeon can practice numerous cases with realistic anatomy, localization of tool or implant trajectories, and receive immediate feedback. Figure 3 demonstrates needle localization in a virtual patient. The surgeon can then perform the real operation knowing a clear plan, having performed a similar operation in a realistic, multi-sensory manner as many times as desired preoperatively. As the surgeon increases his/her endoscopic caseload, the iVR system may be tailored to increasing complexity of cases, and aggregate user outcome data in virtual cases can be compared to real-life patient outcomes. Our review has demonstrated evidence in the use of VR systems for training of both novices and established surgeons in endoscopic procedures as well as clinical cases. Following the above outline for endoscopic procedural adoption may provide a cost-effective, enjoyable, and safe avenue of changing practice. We have previously performed a blinded randomized controlled trial demonstrating 570% efficiency in training time for those using iVR when compared to didactic or pre-surgical planning using written surgical techniques (R Lohre and DP Goel, submitted) and superior technical skills with equivalent verbal and written knowledge scoring. Furthermore, a unique option available in iVR, is that of “multiplayer”. This feature permits an expert surgeon to educate novice surgeons (regardless of geographical location) within the same virtual OR and within the convenience of their home city. The time and cost efficiency imparted through its use is further augmented by the ability to research, collaborate, and educate through this modality.
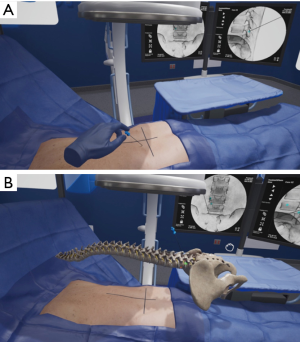
Conclusions
The presented review highlights 38 studies incorporating VR, AR, and MR in MISS and spinal endoscopic surgery. Published studies have utilized this technology for surgical training and clinical application for less than 10 years. Virtual simulators routinely outperformed traditional methods of training for MISS procedures including pedicle screw insertion in both novice and expert cohorts. Surgical simulators have been used to improve clinical results in early follow-up for both vertebroplasty and PLED with reported patient outcome measures. Overall included study quality was low to medium, with limited LoE and no direct transfer validity to real scenarios demonstrated for training studies. Higher quality, randomized studies with clear objectives, longer term results, and standardized reporting are required to more clearly demonstrate the benefit of virtual simulators in MISS and spinal endoscopic surgery. VR, AR, and MR simulators in spinal endoscopic surgery stand to complement surgical training programs, preoperative planning and intra-operative use. Strong consideration to evaluate and use iVR for a comprehensive evaluation including patient positioning, variation in cases and patient specific planning is currently available and may provide a cost efficient and scalable method to introduce simulation internationally with cross collaboration and case sharing.
Acknowledgments
None.
Footnote
Conflicts of Interest: Dr. DP Goel is the CEO of PrecisionOS Technology, a virtual reality company. The other authors have no conflicts of interest to declare.
Ethical Statement: The authors are accountable for all aspects of the work in ensuring that questions related to the accuracy or integrity of any part of the work are appropriately investigated and resolved.
References
- Aïm F, Lonjon G, Hannouche D, et al. Effectiveness of Virtual Reality Training in Orthopaedic Surgery. Arthroscopy 2016;32:224-32. [Crossref] [PubMed]
- Vaughan N, Dubey VN, Wainwright TW, et al. A review of virtual reality based training simulators for orthopaedic surgery. Med Eng Phys 2016;38:59-71. [Crossref] [PubMed]
- Guha D, Alotaibi NM, Nguyen N, et al. Augmented Reality in Neurosurgery: A Review of Current Concepts and Emerging Applications. Can J Neurol Sci 2017;44:235-45. [Crossref] [PubMed]
- Burström G, Nachabe R, Persson O, et al. Augmented and Virtual Reality Instrument Tracking for Minimally Invasive Spine Surgery: A Feasibility and Accuracy Study. Spine 2019;44:1097-104. [Crossref] [PubMed]
- Bernardo A. Virtual Reality and Simulation in Neurosurgical Training. World Neurosurg 2017;106:1015-29. [Crossref] [PubMed]
- Konakondla S, Fong R, Schirmer CM. Simulation training in neurosurgery: advances in education and practice. Adv Med Educ Pract 2017;8:465-73. [Crossref] [PubMed]
- Oliveira LM, Figueiredo EG. Simulation Training Methods in Neurological Surgery. Asian J Neurosurg 2019;14:364-70. [Crossref] [PubMed]
- Pfandler M, Lazarovici M, Stefan P, et al. Virtual reality-based simulators for spine surgery: a systematic review. Spine J 2017;17:1352-63. [Crossref] [PubMed]
- Wewel JT, Godzik J, Uribe JS. The utilization of minimally invasive surgery techniques for the treatment of spinal deformity. J Spine Surg 2019;5:S84-S90. [Crossref] [PubMed]
- Iprenburg M, Wagner R, Godschalx A, et al. Patient radiation exposure during transforaminal lumbar endoscopic spine surgery: a prospective study. Neurosurg Focus 2016;40:E7. [Crossref] [PubMed]
- Butler AJ, Alam M, Wiley K, et al. Endoscopic Lumbar Surgery: The State of the Art in 2019. Neurospine 2019;16:15-23. [Crossref] [PubMed]
- Hwa Eum J, Hwa Heo D, Son SK, et al. Percutaneous biportal endoscopic decompression for lumbar spinal stenosis: a technical note and preliminary clinical results. J Neurosurg Spine 2016;24:602-7. [Crossref] [PubMed]
- Jacquot F, Gastambide D. Percutaneous endoscopic transforaminal lumbar interbody fusion: is it worth it?. Int Orthop 2013;37:1507-10. [Crossref] [PubMed]
- Shi R, Wang F, Hong X, et al. Comparison of percutaneous endoscopic lumbar discectomy versus microendoscopic discectomy for the treatment of lumbar disc herniation: a meta-analysis. Int Orthop 2019;43:923-37. [Crossref] [PubMed]
- Marappan K, Jothi R, Paul Raj S. Microendoscopic discectomy (MED) for lumbar disc herniation: comparison of learning curve of the surgery and outcome with other established case studies. J spine Surg 2018;4:630-7. [Crossref] [PubMed]
- Nowitzke AM. Assessment of the learning curve for lumbar microendoscopic discectomy. Neurosurgery 2005;56:755-62. [Crossref] [PubMed]
- Soliman HM. Irrigation endoscopic discectomy: a novel percutaneous approach for lumbar disc prolapse. Eur Spine J 2013;22:1037-44. [Crossref] [PubMed]
- Gonzalvo A, Fitt G, Liew S, et al. The learning curve of pedicle screw placement: how many screws are enough? Spine (Phila Pa 1976) 2009;34:E761-5. [Crossref] [PubMed]
- Carter FJ, Schijven MP, Aggarwal R, et al. Consensus guidelines for validation of virtual reality surgical simulators. Simul Healthc 2006;1:171-9. [Crossref] [PubMed]
- Gibby JT, Swenson SA, Cvetko S, et al. Head-mounted display augmented reality to guide pedicle screw placement utilizing computed tomography. Int J Comput Assist Radiol Surg 2019;14:525-35. [Crossref] [PubMed]
- Luciano CJ, Banerjee PP, Sorenson JM, et al. Percutaneous spinal fixation simulation with virtual reality and haptics. Neurosurgery 2013;72 Suppl 1:89-96. [Crossref] [PubMed]
- Umebayashi D, Yamamoto Y, Nakajima Y, et al. Augmented Reality Visualization-guided Microscopic Spine Surgery: Transvertebral Anterior Cervical Foraminotomy and Posterior Foraminotomy. J Am Acad Orthop Surg Glob Res Rev 2018;2:e008. [PubMed]
- Deib G, Johnson A, Unberath M, et al. Image guided percutaneous spine procedures using an optical see-through head mounted display: proof of concept and rationale. J Neurointerv Surg 2018;10:1187-91. [Crossref] [PubMed]
- Archavlis E, Schwandt E, Kosterhon M, et al. A Modified Microsurgical Endoscopic Assisted Transpedicular Corpectomy of the Thoracic Spine Based on Virtual 3D Planning, Technical Note. World Neurosurg 2016;91:424-33. [Crossref] [PubMed]
- Gottschalk MB, Yoon ST, Park DK, et al. Surgical training using three-dimensional simulation in placement of cervical lateral mass screws: a blinded randomized control trial. Spine J 2015;15:168-75. [Crossref] [PubMed]
- Hu Z, Li X, Cui J, et al. Significance of preoperative planning software for puncture and channel establishment in percutaneous endoscopic lumbar DISCECTOMY: A study of 40 cases. Int J Surg 2017;41:97-103. [Crossref] [PubMed]
- Yu H, Zhou Z, Lei X, et al. Mixed Reality-Based Preoperative Planning for Training of Percutaneous Transforaminal Endoscopic Discectomy: A Feasibility Study. World Neurosurg 2019;129:e767-75. [Crossref] [PubMed]
- Wei P, Yao Q, Xu Y, et al. Percutaneous kyphoplasty assisted with/without mixed reality technology in treatment of OVCF with IVC: a prospective study. J Orthop Surg Res 2019;14:255. [Crossref] [PubMed]
- Chitale R, Ghobrial GM, Lobel D, et al. Simulated Lumbar Minimally Invasive Surgery Educational Model With Didactic and Technical Components. Neurosurgery 2013;73:107-10. [PubMed]
- Weigl M, Stefan P, Abhari K, et al. Intra-operative disruptions, surgeon's mental workload, and technical performance in a full-scale simulated procedure. Surg Endosc 2016;30:559-66. [Crossref] [PubMed]
- Wucherer P, Stefan P, Weidert S, et al. Task and crisis analysis during surgical training. Int J Comput Assist Radiol Surg 2014;9:785-94. [Crossref] [PubMed]
- Wucherer P, Stefan P, Abhari K, et al. Vertebroplasty Performance on Simulator for 19 Surgeons Using Hierarchical Task Analysis. IEEE Trans Med Imaging 2015;34:1730-7. [Crossref] [PubMed]
- Färber M, Hummel F, Gerloff C, et al. Virtual reality simulator for the training of lumbar punctures. Methods Inf Med 2009;48:493-501. [Crossref] [PubMed]
- Abe Y, Sato S, Kato K, et al. A novel 3D guidance system using augmented reality for percutaneous vertebroplasty: technical note. J Neurosurg Spine 2013;19:492-501. [Crossref] [PubMed]
- Cook DA, Reed DA. Appraising the quality of medical education research methods: the Medical Education Research Study Quality Instrument and the Newcastle-Ottawa Scale-Education. Acad Med 2015;90:1067-76. [Crossref] [PubMed]
- Koch A, Pfandler M, Stefan P, et al. Say, What Is on Your Mind? Surgeons' Evaluations of Realism and Usability of a Virtual Reality Vertebroplasty Simulator. Surg Innov 2019;26:234-43. [Crossref] [PubMed]
- Ma L, Zhao Z, Chen F, et al. Augmented reality surgical navigation with ultrasound-assisted registration for pedicle screw placement: a pilot study. Int J Comput Assist Radiol Surg 2017;12:2205-15. [Crossref] [PubMed]
- Elmi-Terander A, Burström G, Nachabe R, et al. Pedicle Screw Placement Using Augmented Reality Surgical Navigation With Intraoperative 3D Imaging: A First In-Human Prospective Cohort Study. Spine (Phila Pa 1976) 2019;44:517-25. [Crossref] [PubMed]
- Kulcsár Z, O'Mahony E, Lövquist E, et al. Preliminary evaluation of a virtual reality-based simulator for learning spinal anesthesia. J Clin Anesth 2013;25:98-105. [Crossref] [PubMed]
- Zhou Z, Hu S, Zhao YZ, et al. Feasibility of Virtual Reality Combined with Isocentric Navigation in Transforaminal Percutaneous Endoscopic Discectomy: A Cadaver Study. Orthop Surg 2019;11:493-9. [Crossref] [PubMed]
- Mostafa AE, Ryu WHA, Chan S, et al. Designing NeuroSimVR: A Stereoscopic Virtual Reality Spine Surgery Simulator. 2017. doi: http://dx.doi.org/. [Crossref]
- Xiang L, Zhou Y, Wang H, et al. Significance of preoperative planning simulator for junior surgeons' training of pedicle screw insertion. J Spinal Disord Tech 2015;28:E25-9. [Crossref] [PubMed]
- Keri Z, Sydor D, Ungi T, et al. Computerized training system for ultrasound-guided lumbar puncture on abnormal spine models: a randomized controlled trial. Can J Anaesth 2015;62:777-84. [Crossref] [PubMed]
- Moult E, Ungi T, Welch M, et al. Ultrasound-guided facet joint injection training using Perk Tutor. Int J Comput Assist Radiol Surg 2013;8:831-6. [Crossref] [PubMed]
- Yeo CT, Ungi T. The effect of augmented reality training on percutaneous needle placement in spinal facet joint injections. IEEE Trans Biomed Eng 2011;58:2031-7. [Crossref] [PubMed]
- Naddeo F, Cataldo E, Naddeo A, et al. An automatic and patient - specific algorithm to design the optimal insertion direction of pedicle screws for spine surgery templates. Med Biol Eng Comput 2017;55:1549-62. [Crossref] [PubMed]
- Luciano CJ, Banerjee PP, Bellotte B, et al. Learning retention of thoracic pedicle screw placement using a high-resolution augmented reality simulator with haptic feedback. Neurosurgery 2011;69:ons14-9; discussion ons19.
- Rambani R, Ward J, Viant W. Desktop-based computer-assisted orthopedic training system for spinal surgery. J Surg Educ 2014;71:805-9. [Crossref] [PubMed]
- Sutherland C, Hashtrudi-Zaad K, Sellens R, et al. An augmented reality haptic training simulator for spinal needle procedures. IEEE Trans Biomed Eng 2013;60:3009-18. [Crossref] [PubMed]
- Van Nortwick SS, Lendvay TS, Jensen AR, et al. Methodologies for establishing validity in surgical simulation studies. Surgery 2010;147:622-30. [Crossref] [PubMed]
- Cheng A, Kessler D, Mackinnon R, et al. Reporting Guidelines for Health Care Simulation Research: Extensions to the CONSORT and STROBE Statements. Simul Healthc 2016;11:238-48. [Crossref] [PubMed]
- Hou Y, Lin Y, Shi J, et al. Effectiveness of the Thoracic Pedicle Screw Placement Using the Virtual Surgical Training System: A Cadaver Study. Oper Neurosurg (Hagerstown) 2018;15:677-85. [Crossref] [PubMed]
- Hou Y, Shi J, Lin Y, et al. Virtual surgery simulation versus traditional approaches in training of residents in cervical pedicle screw placement. Arch Orthop Trauma Surg 2018;138:777-82. [Crossref] [PubMed]
- Shi J, Hou Y, Lin Y, et al. Role of Visuohaptic Surgical Training Simulator in Resident Education of Orthopedic Surgery. World Neurosurg 2018;111:e98-104. [Crossref] [PubMed]
- Ericsson KA. Deliberate practice and the acquisition and maintenance of expert performance in medicine and related domains. Acad Med 2004;79:S70-81. [Crossref] [PubMed]
- Henz D, Schöllhorn WI. Differential Training Facilitates Early Consolidation in Motor Learning. Front Behav Neurosci 2016;10:199. [Crossref] [PubMed]
- Gasco J, Patel A, Ortega-Barnett J, et al. Virtual reality spine surgery simulation: an empirical study of its usefulness. Neurol Res 2014;36:968-73. [Crossref] [PubMed]
- Choque-Velasquez J, Colasanti R, Collan J, et al. Virtual Reality Glasses and "Eye-Hands Blind Technique" for Microsurgical Training in Neurosurgery. World Neurosurg 2018;112:126-30. [Crossref] [PubMed]
- Xin B, Chen G, Wang Y, et al. The Efficacy of Immersive Virtual Reality Surgical Simulator Training for Pedicle Screw Placement: A Randomized Double-Blind Controlled Trial. World Neurosurg 2018;124:e324-30. [Crossref] [PubMed]
- Liu X, Bai H, Song G, et al. Augmented reality system training for minimally invasive spine surgery. 2017 IEEE International Conference on Robotics and Biomimetics (ROBIO); 5-8 Dec. 2017; Macau, China. IEEE, 2017.
- Elmi-Terander A, Nachabe R, Skulason H, et al. Feasibility and Accuracy of Thoracolumbar Minimally Invasive Pedicle Screw Placement With Augmented Reality Navigation Technology. Spine (Phila Pa 1976) 2018;43:1018-23. [Crossref] [PubMed]