Clinical outcomes for anterior cervical discectomy and fusion with silicon nitride spine cages: a multicenter study
Introduction
Anterior cervical discectomy and fusion (ACDF) is performed to restore intervertebral height and alignment and to relieve neck and radicular pain. The procedure uses either allogenic spacers or abiotic cages (containing a central lumen for bone graft) to facilitate fusion. Autograft is still considered to be the gold standard for spacers due to rapid bone healing, but it is limited by harvest site morbidities (1). Structural allograft is also commonly used as a spacer, but it lacks curative effectiveness and has the finite possibility for disease transmission (1). Due to these limitations, synthetic cage materials including titanium, polyetheretherketone (PEEK), tantalum, and silicon nitride (Si3N4) have largely displaced allogenic spacers (2-4). The BAK cage, which was subjected to extensive clinical trials in the 1990s, is often cited as the first truly abiotic spacer that was successfully employed in cervical and lumbar fusion (5,6). However, it is not well known that porous silicon nitride (Si3N4) actually predated the BAK cage by about 6 years (7). Si3N4 spacers were introduced in a small human clinical trial in Australia beginning in 1986. They now have the longest clinical history of any spacer—exceeding 30 years (8). Partially based on these results, Si3N4 was cleared by the US FDA as intervertebral devices in 2008. Although it now has a 10-year history in the USA and EU, only a limited number of case reports and small single-center retrospective studies, along with one randomized controlled trial, have been published (9-13).
The purpose of this study was to augment these data with a significant retrospective review consisting of 860 patients at four US surgical centers. Preoperative patient demographics, pain scores, comorbidity data along with post-operative last follow-up pain scores, complications, adverse events, and secondary surgical interventions (SSI) were extracted from patients’ records. Results were compared with a meta-analysis of nine studies, consisting of 13 cohorts and 736 patients who underwent similar ACDF procedures using other commonly accepted spacers or cages. The hypothesis of this comparative analysis was that Si3N4 cages would produce similar clinical outcomes to the other allogenic spacers or synthetic cages.
Methods
Retrospective chart review of patients receiving Si3N4 spacers
Following required reviews of the study protocol, an independent trained evaluator reviewed the medical charts of all patients who underwent ACDF by each of four surgeons working at different medical centers between November 2017 and June 2018. Patient information and data remained anonymous and in compliance with IRB standards. A total of 860 patients had ACDF procedures. Inclusion criteria are listed Table 1. Data were recorded from both digital and active or archival hard copy files.
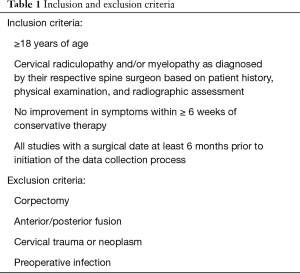
Full table
The Si3N4 cervical spacers used in these procedures are shown in Figure 1. The spacers were produced in two footprints—14 mm × 12 mm and 16 mm × 14 mm—with heights ranging from 5 to 12 mm and two lordotic angles—0° and 6°. A total of 1,482 Si3N4 devices were implanted. Figure 2 provides a breakdown of the number of single and multilevel operations for each center. Overall, 50.0% of the patients had single-level, 35.6% 2-level, 12.8% 3-level, and 1.6% four-level procedures. Figure 3 shows the number of implants placed at each segmental level from C2/C3 through C7/T1. Most of the patients (86.7%) received implants at C4/C5 through C6/C7.
Surgical procedures
Each surgeon used a standard Smith-Robinson surgical approach for ACDF (14). After complete discectomy with release of the posterior longitudinal ligament (PLL) and decompression of the uncinate process, vertebral endplates were prepared while maintaining their structural integrity. Bone graft consisting of local morselized autograft, sometimes supplemented with morselized allograft or a synthetic bone extender, was placed into the lumen of the spacer. Appropriately-sized Si3N4 cages were then implanted and anterior plate/screw fixation was used according to surgeon preference. Patients were mobilized soon after surgery without cervical orthoses. Upon discharge, they were instructed to restrict lifting to less than ~4.5 kg (<10 lbs.) during the initial recovery period of 6 weeks and no more than ~11.3 kg (25 lbs.) between 6 and 12 weeks. They were also instructed to avoid repetitive bending or twisting of the neck for at least 3 months.
Data acquisition
Preoperative demographic data (age, gender, height, weight, BMI, and diagnoses), comorbidity conditions (smoking, diabetes, hypertension, osteoporosis, osteopenia, tumor, and other), and post-operative results (days to last follow-up, pain scores, complications, adverse events, and secondary surgical interventions) were extracted from the medical charts. Pain scores were assessed using the visual analog scale, zero being “no pain” and ten being the “worst pain imaginable.” Pain scores were taken as the maximum of either neck, arm, or bodily pain at each follow-up visit. For consistency with the metadata, scores were converted to a zero to 100-point scale. Complications and adverse events included hematomas, hoarseness, infection, nerve damage, pseudarthrosis, or recurrent symptoms. Secondary surgical interventions were compiled for patients experiencing screw migration, adjacent-level disease (ALD), and pseudarthrosis (i.e., delayed, or non-union).
Meta-analysis
A meta-analysis was performed to quantitatively assess and compare differential changes in pain scores, complications, adverse events, and SSI for patients implanted with Si3N4 cages versus other commonly used ACDF spacers or cages. MEDLINE/PubMed was searched for relevant publications using a human clinical query with the search terms of “(Anterior Cervical Discectomy and Fusion) AND (Pain) AND (VAS)” along with filters for years (2000 to 2019), abstract and full text in English, and Adults (≥19 years of age). The output was augmented by a Google Scholar search with the added terms of “(Standard Deviation) OR (Confidence Interval)”. Article titles and abstracts were then compared, and duplicates removed. Additional ACDF clinical papers were identified from a number of published systematic reviews and meta-analyses (15-23) and by manual searches. Papers were excluded if the reported studies were for follow-up periods of <6 months or if they lacked quantifiable statistical data for pre- and follow-up pain scores. Of the remaining articles, those selected for inclusion had statistically similar pre-op demographics. This resulted in the inclusion of nine studies which consisted of 13 cohorts and 736 patients (24-32). The PRISMA (Preferred Reporting Items for Systematic Reviews and Meta-Analyses) flow diagram for included articles is shown in Figure 4 (33).
Statistical analysis
Statistical analyses including metadata comparisons were performed using MedCalc Ver. 18.6 – 64 bit (Ostend, Belgium). Ordinal data were analyzed using Student’s t-tests whereas nominal results used proportionality assessments including Chi-squared and Fisher’s exact tests. Significance was set at P values of <0.05. Biomedical Statistical Consulting (Wynnewood, PA USA) assisted in performing the meta-analysis.
Results
Pre-op diagnoses, demographics, and comorbidities
Admission records showed that most patient diagnoses were either spondylosis (35.9%), spinal stenosis (32.6%), or disc herniation (20.1%). Complete etiological data are shown in Table 2. Of the 860 patient records included in the study, the average age was 57.9±12.2 years, 46.5% were female, and the average BMI score was 30.0±6.3. There were no statistical differences between the four centers for gender or BMI. However, patients in Center 4 were statistically younger than the remaining three centers (i.e., 52.8 versus 57.9 years, P<0.01). Pre-op comorbidities are presented in Table 3. The patient count in this and subsequent tables or charts does not total to the original enrollment due to the fact that some data were missing from patients’ records. There was a smaller proportion of patients from Center 3 that were smokers (7.7% versus 20.3%, cf., Table 3, P<0.01) and there was also a higher percentage of patients from Center 4 with hypertension (36.1% versus 24.1%, cf., Table 3, P=0.04). Heterogeneity tests were conducted for differences in these demographic and pre-op comorbidities. Based on these calculations, the centers were considered to have homogeneous statistics for gender (I2=18.4%, P=0.30), BMI (I2=13.7%, P=0.32), and diabetes (I2=51.5%, P=0.10) but heterogeneous data for age (I2=78.7%, P<0.01), smoking (I2=92.4%, P<0.01), and hypertension (I2=71.2%, P=0.02). Also, as shown in Figures 2 and 3, each center performed a significant number of multilevel surgeries. This ranged from ~95% of the patients in Center 2 to ~25% for Center 1. There was also considerable heterogeneity between the centers with respect to single versus multilevel operations (I2=96.2%, P<0.01). Only Centers 2 and 4 were statistically equivalent in the number of multilevel implantations (P=0.19). Combined, these data suggest that the patients comprising this study were drawn from a broad range of pre-op cervical maladies which are typical of conditions generally encountered by spine surgeons in the US population.
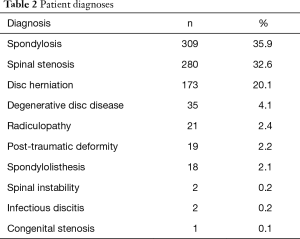
Full table
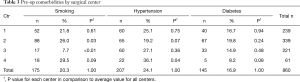
Full table
Clinical outcomes
Average time to last-follow-up for each of the four surgical centers is presented in Table 4. Significant differences were noted in last follow-up periods with Center 3 having the shortest period (209±264 days, 7.0±8.8 months) and Center 2 having the longest (430±397 days, 14.3±13.2 months). The overall longest follow-up also occurred for Center 2 at 2,351 days (~6.5 y). Clinical results for changes in VAS pain scores for the four centers are provided in Table 5. Patients from each center experienced significant reductions in VAS pain scores (P<0.01) from pre-op to last follow-up. A summary of VAS pain scores for each center along with their statistical significance is shown in Figure 5. Patients from Center 1 had the largest reductions in pain (42.6 points) with patients from Center 4 showing the smallest change (32.8 points). Overall, 81.7% of the patients from the four centers reported an improvement in pain at ≤2-year follow-up. Of this total, 20.4% showed minimal reductions of less than 20 points, while 50.4% had >40-point change at their last-follow-up. These data were also heterogeneous between the four centers: pre-op (I2=74.8%, P=0.01), last follow-up (I2=71.8%, P=0.01), and ΔVAS (I2=71.0%, P=0.02). Separate correlation analyses indicated no observable trends associated with any of the pre-op demographics, comorbidities, or number of surgical levels.
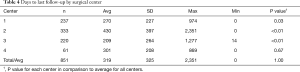
Full table
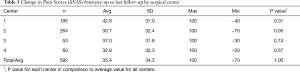
Full table
Box and whisker plots for pain scores are provided in Figure 6 as a function of last follow-up period. The largest reduction in pain occurred in the post-operative periods up to two years. Mean values dropped from 71-points for pre-op to 34.3-, 31.1-, 30.6-, and 28.8-points for the periods of <3, 3-6, 6-9, and 9-12 months, respectively. Thereafter, pain scores moderately increased for the remaining patients at 1−2 years (38.4 points) and >2 years (52.8-point), but they never returned to their pre-op levels. The increase in the later follow-up periods is not surprising because these patients had a higher percentage of diabetes (24.8% versus 16.9%, P<0.01) and hypertension (31.6% versus 21.4%, P=0.02), or had undergone a greater number of multilevel procedures (60.4% versus 50.0%, P<0.01). There were two revision surgeries associated with this group as well.
Meta-analysis
Clinical results from nine ACDF studies which included 13 cohorts and 736 patients were selected for comparison with the Si3N4 data. Although a significant number of studies were originally considered, the majority failed to meet the inclusion criteria of single to multilevel ACDF procedures with similar demographics. Most studies had much younger patient populations (i.e., typically >10-year differential). The nine included studies contained seven retrospective reviews and two prospective trials for various ACDF materials. Brief synopses of the selected papers are provided in Table 6. Two of the studies compared 2-level ACDF with 1-level anterior cervical corpectomy and fusion (ACCF). An additional two studies examined 3- or 4-level ACDF using PEEK cages, with and without anterior plating. One prospective study compared multilevel ACDF with multilevel posterior laminoplasty and a second prospective study examined the effect of racial ethnicity on ACDF outcomes. The remaining studies examined either the clinical important methodologies for assessing ACDF outcomes and the effect of preoperative depression management, or tracheal retraction exercises on ACDF results.
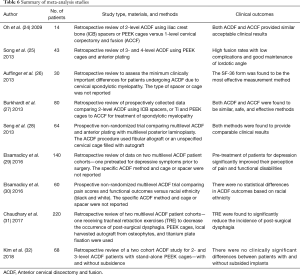
Full table
Table 7 provides a comparison of the pre-op demographics and comorbidities for the Si3N4 group and the metadata. There were no statistical differences in gender, age, and smoking for the two groups (i.e., P=0.06 to 0.55). However, BMI values for the Si3N4 patients were significantly higher than the metadata (i.e., 30.0 versus 28.1, P<0.01). Remarkably, this difference was universally found for most of the studies initially reviewed for inclusion in the meta-analysis. In fact, only 7 of the 69 studies considered for inclusion had BMI similar values to the Si3N4 group, yet most failed to have appropriate demographics (i.e., age, gender) and only three were eventually included in the final meta-analysis. This observation suggests that on average the Si3N4 patients of this study bordered on being morbidly obese as compared with most other published ACDF trials.
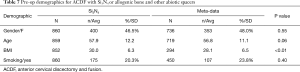
Full table
Table 8 shows the clinical results for the Si3N4 group and the compiled metadata. VAS pain scores for the Si3N4 patients were higher at both pre-op and last follow-up when compared with the metadata, but the overall changes in pain scores (ΔVAS) were statistically equivalent. The higher pre-op and last follow-up pain scores for the Si3N4 patients of this study may have been a consequence of patient hypertension, diabetes, and related comorbidities. A heterogeneity test for this metadata is provided in the funnel plot of Figure 7. This test compares mean and 95% confidence intervals for changes in VAS pain scores for the 13 meta-analysis cohorts to the mean and pooled 95% confidence interval from this Si3N4 study. The test indicates reasonable homogeneity of the data (I2 =42.6%, P=0.052). A subsequent forest plot, using the same comparative data, is given in Figure 8. The results of the forest plot compliment the statistical analysis of Table 8 and suggest that changes in pain scores between the two groups were essentially equivalent under either fixed or random effects assumptions.
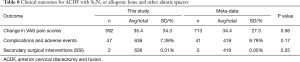
Full table
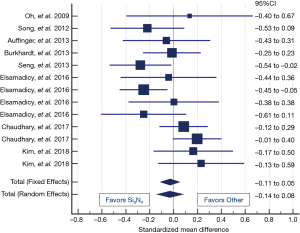
Table 8 also shows that complications or adverse events and secondary surgical interventions (SSI) were also statistically equivalent. The complication rate of the Si3N4 patients was ~7.4% compared to ~9.8% for the metadata (P=0.17). There were two SSI incidents for the Si3N4 patients and none for patients included in the meta-analysis (P=0.25). Additional details on complications, adverse events, and SSI are provided in Table 9. A recurrence of symptoms was the most common complication within the Si3N4 group (n=20, 3.1%), followed by additional diagnoses of adjacent level disease (n=13, 2.0%). Revision surgeries were performed on two patients for the malposition and back-out of a screw and an unresolved hematoma. Although the complication rate for the metadata was slightly higher than for Si3N4, the incidence of adverse events was broader ranging from respiratory discomfort and dysphagia to hematomas and urinary tract infections. There were no reported revisions for the metadata.
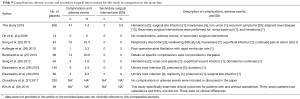
Full table
Discussion
Clinical effectiveness of Si3N4
Although Si3N4 has a long and valued history as an industrial ceramic (34), it has only been approved for clinical use during the past decade. The material exhibits many qualities desired in spine fusion cages, such as mechanical integrity (35), enhanced osteoconductivity (36-38), bacteriostasis (39-42), and improved radiolucency (12,43). There is a misperception among surgeons that all ceramics are brittle; but in fact, Si3N4 is one of the strongest and toughest biomaterials known (44,45). Si3N4 has ten-times the strength and toughness of PEEK and can withstand failure loads equivalent to medical titanium (46). Recent studies have shown that Si3N4 is no more prone to subsidence than any other biomaterial used in cervical spinal fusion (47). In pre-clinical studies, its unique surface chemistry, topography, and hydrophilicity not only upregulate osteogenic activity to achieve faster fusion, but also simultaneously prevent bacterial adhesion and biofilm formation (48-53). Thus, while some claim that the ideal spine biomaterial has yet to be identified (3), others suggest that Si3N4 may be the obvious future choice (54,55).
The present study is the largest one of its kind; reporting multi-center clinical evidence of the safety and efficacy of Si3N4 in cervical fusion. Si3N4 was the first synthetic material used in spinal arthrodesis, with a 30-patient cohort undergoing fusion in the mid-1980s (8). While that study addressed lumbar fusion, the VAS pain scores were similar to those observed in the present study. Initial reductions of up to 47 points were seen during the first 5-years post-operatively with lower reductions further out (i.e., 35 points at 10 years). Complication rates were higher (n=11, 36.7%) in the lumbar study, reflecting a rudimentary design of the spinal implants used at the time.
Following this seminal work, several additional studies have described the performance of Si3N4 in cervical fusion. For instance, Ball et al. published a 36-month retrospective clinical comparison of the osseointegration and fusion performance of Si3N4 versus PEEK spacers (9). Those data showed no differences in 36-month outcomes, but early fusion and pain score results suggested the superiority of Si3N4 over PEEK cages. A subsequent retrospective study by Smith et al. compared Si3N4 cages to fibula-based allograft spacers (10). In their 92-patient study, the implants were assessed for fusion, osseous integration, and subsidence for up to 24-month. The results demonstrated that both materials were effective in achieving acceptable arthrodesis at ≥12 months, but earlier periods favored the use of the Si3N4. At 3-, 6-, and 12-month follow-up, Si3N4 patients showed faster fusion, greater osseous integration, and lower subsidence. Lastly, a 24-month prospective randomized clinical trial of ~100 patients compared PEEK and Si3N4 spacers in ACDF (56). The PEEK cages were packed with autograft while the Si3N4 spacers had porous ceramic cores (i.e., no bone graft was used). Both groups had comparable clinical and radiographic outcomes at 24-month, indicating the non-inferiority of Si3N4 when compared to PEEK. The study also showed that Si3N4 was highly effective in generating solid fusion even without added autograft bone chips.
Preoperative demographics and comorbidities
The patients of the present study were older and had higher BMI values than comparative metadata. A significant percentage of the Si3N4 patients were diabetic, hypertensive, or smokers. Successful bone fusion can be challenging with age-related comorbidities, but reports from at least two studies suggest that reduction in pain scores in elderly patients may be equivalent to younger counterparts (57,58). This finding is consistent with the present study. Additionally, three studies by Sielatycki et al. (59), Narain et al. (60), and Srinivasan et al. (61) correlated the effect of obesity on patient reported outcomes for elective ACDF. Although VAS pain scores were not monitored in these studies, other clinical measures suggested that obese patients were no more likely to experience greater perioperative complications or post-operative functional disabilities than patients with normal body mass indices. Similarly, Mayo et al. found that preoperative smoking did not affect post-operative pain scores (62).
Diabetes and hypertension are common comorbidities in the general population, but have increased coincidence for older patients undergoing cervical spine surgery (63). The present study suggests that patients with these comorbidities were more likely to have poorer clinical outcomes. This finding is consistent with other ACDF studies suggesting that these conditions lead to longer hospital stays (64), higher complication rates and adverse events (65), more revisions (66), and higher odds ratios for readmission and/or multilevel surgeries (66).
Minimum clinical important differences in pain scores
In the present study, the average reduction in pain scores (ΔVAS) with Si3N4 spacers was between 30.7- to 42.6-points. Several studies have attempted to address the minimum clinically important difference (MCID) for changes in VAS pain associated with ACDF surgery (26,67-69). The concept of MCID can be simply stated as “the smallest change in pain that is important to the patient” (67). There is a considerable range in MCID values (0 to 100-point scale) from these studies, including a 21.4- to 26-point spread for neck pain, 25- to 41-point for arm pain, and 19.5 for general bodily pain. An additional ACDF review by Kersten et al. suggested MCID for pain ranges between 25- to 26-points for neck, 25- to 46-points for arm, and 15 to 25-points for general bodily pain (70). MCID values can vary greatly based on patients’ diagnoses, the specific surgical procedure, and the MCID calculation method itself (71). Because of this, definitive MCID values for patients undergoing ACDF surgery have yet to be clearly established (68). Nevertheless, although the present study did not differentiate between anatomical pain locations, an estimate of ≥40-points appears to be a conservative MCID benchmark.
A large multi-surgeon study by Carreon et al. attempted to clearly define the MCID for ACDF (67). They followed self-reported pain scores on 505 patients for one-year. Although the demographics of the patients were different than the present study (i.e., younger and with a higher percentage of females), the indications for treatment and pre-op neck pain were similar. Conversely, their average change in VAS pain from pre-op to follow-up was only 23-points compared to 35.4-points in the present study (cf., Table 8). Despite this smaller reduction, they calculated the MCID to be ≥25-points for either neck or arm pain. Using this standard, only 44.6% and 45.5% of their patients had perceptible neck and arm pain reductions, respectively. In contrast, in the present study, 50.4% of the patients reported pain reductions of ≥40-point. This direct comparison further supports Si3N4’s effectiveness as an arthrodesis device in the cervical spine.
Limitations
The present study is limited by its retrospective design. While patient data was collected from medical charts using a prescribed protocol by an independent examiner, the prior recording of each patient’s data was not. Therefore, the use of a consistent set of clinical evaluation tools including validated pain or disability indices and questionnaires was not possible. The simple patient reported outcome of VAS pain was the only clinical measure, but even so, neck, arm, and bodily pain were not clearly separated and categorized. Nevertheless, zero to 10 (or zero to 100) pain scales are simple to administer and evaluate. An additional limitation is the lack of a consistent last follow-up period between the four centers. The study was also limited by a lack of contemporaneous controls, (i.e., no corresponding ACDF data with other cage materials were available from the four clinical sites). Instead, previously reported metadata were used for comparison of clinical outcomes. Lastly, although the data were acquired by an unbiased medical records contractor, subsequent analyses were done by the study authors who believe in the benefits of Si3N4 spinal spacers. Nonetheless, the statistical analyses and comparison to previously-published metadata fairly represent expected outcomes by other practitioners and surgical centers.
Conclusions
In this large-scale clinical review of patient demographics, comorbidities, and clinical outcomes, a total of 1,428 Si3N4 spacers were implanted during single- and multi-level ACDF. Of 860 patients studied, the majority received multilevel procedures. Patient follow-up averaged 10.6±10.8 months. When compared to other published ACDF studies, the Si3N4 patients of the present study were older, with a higher incidence of comorbidities, particularly those related to obesity, hypertension, and diabetes.
The nine studies that were selected for a comparative meta-analysis had similar demographics and multilevel procedures. The metadata consisted of 13 ACDF cohorts and 736 patients. The analyses suggest that ACDF with Si3N4 cages was at least as safe and effective as similar procedures that relied on structural bone autograft, PEEK, or titanium alloy fusion cages. Although the four centers in this study were heterogeneous in pre-op patient demographics, comorbidities, and pre- and post-op clinical outcomes, the compiled ordinal and nominal data were similar to the selected meta-analysis, suggesting overall equivalence. Lastly, comparative MCID analyses demonstrated that Si3N4 cages were equivalent, if not superior to other commonly used ACDF devices in pain reduction.
Acknowledgments
Donald W. Guthner of Orgenix, LLC is acknowledged for the collection and initial analysis of patient data from the four surgical centers. Appreciation is expressed for assistance from medical, office, and administrative personnel at each of the surgical centers. Biomedical Statistical Consulting is acknowledged for their review and recommendations in performing the meta-analysis.
Footnote
Conflicts of Interest: Drs. GC Calvert, GV Huffmon 3rd, WM Rambo Jr, and MW Smith were consulting surgeons to Amedica Corporation during the course of this study. The study was designed and funded by Amedica Corporation (now SINTX Technologies), Salt Lake City, UT USA, of which Dr. Bryan J. McEntire and Dr. B. Sonny Bal are officers and employees.
Ethical Statement: The authors are solely accountable for all aspects of the work and in ensuring that questions related to the accuracy or integrity of any part of the work are appropriately investigated and resolved. Due to the retrospective nature of the study, informed consent by the respective IRBs of each surgical center was not required. However, none of the patients’ personal data was disclosed, and their records remain fully secured and in compliance with IRB standards.
References
- Giannoudis PV, Dinopoulos H, Tsiridis E. Bone Substitutes: An Update. Injury 2005;36:S20-7. [Crossref] [PubMed]
- Chong E, Pelletier MH, Mobbs RJ, et al. The Design Evolution of Interbody Cages in Anterior Cervical Discectomy and Fusion: A Systematic Review. BMC Musculoskelet Disord 2015;16:99. [Crossref] [PubMed]
- Jain S, Eltorai AEM, Ruttiman R, et al. Advances in Spinal Interbody Cages. Orthop Surg 2016;8:278-84. [Crossref] [PubMed]
- Phan K, Mobbs RJ. Evolution of Design of Interbody Cages for Anterior Lumbar Interbody Fusion. Orthop Surg 2016;8:270-7. [Crossref] [PubMed]
- Hacker RJ, Cauthen JC, Gilbert TJ, et al. A Prospective Randomized Multicenter Clinical Evaluation of an Anterior Cervical Fusion Cage. Spine (Phila Pa 1976) 2000;25:2646-54. [Crossref] [PubMed]
- Kuslich SD, Ulstrom CL, Griffith SL, et al. The Bagby and Kuslich Method of Lumbar Interbody Fusion. Spine (Phila Pa 1976) 1998;23:1267-78. [Crossref] [PubMed]
- Sorrell CC, Hardcastle PH, Druitt RK, et al. Results of 15-Year Clinical Study of Reaction Bonded Silicon Nitride Intervertebral Spacers. Proc 7th World Biomaterials Congress 2004;1872.
- Mobbs RJ, Rao PJ, Phan K, et al. Anterior Lumbar Interbody Fusion Using Reaction Bonded Silicon Nitride Implants: Long Term Case Series of the First Synthetic ALIF Spacer Implanted in Humans. World Neurosurg 2018;120:256-64. [Crossref] [PubMed]
- Ball HT, McEntire BJ, Bal BS. Accelerated Cervical Fusion of Silicon Nitride versus PEEK Spacers: A Comparative Clinical Study. J Spine 2017;6:1000396. [Crossref]
- Smith MW, Romano DR, McEntire BJ, et al. A Single Center Retrospective Clinical Evaluation of Anterior Cervical Discectomy and Fusion Comparing Allograft Spacers to Silicon Nitride Cages. J Spine Surg 2018;4:349-60. [Crossref] [PubMed]
- Rambo WM. Treatment of Lumbar Discitis using Silicon Nitride Spinal Spacers: A Case Series and Literature Review. Int J Surg Case Rep 2018;43:61-8. [Crossref] [PubMed]
- Youssef JA, Myhre SL, Bal BS. Radiographic Follow-up of Transforaminal Lumbar Fusion with Silicon Nitride Spacers: A Case Report of Two Patients. J Musculoskelet Disord Treat 2016;2:1-8. [Crossref]
- Arts MP, Wolfs JFC, Corbin TP. Porous Silicon Nitride Spacers versus PEEK Cages for Anterior Cervical Discectomy and Fusion: Clinical and Radiological Results of a Single-Blinded Randomized Controlled Trial. Eur Spine J 2017;26:2372-9. [Crossref] [PubMed]
- Aronson N, Filtzer DL, Bagan M. Anterior Cervical Fusion by the Smith-Robinson Approach. J Neurosurg 1968;29:396-404. [Crossref] [PubMed]
- Fallah A, Akl EA, Ebrahim S, et al. Anterior Cervical Discectomy with Arthroplasty versus Arthrodesis for Single-Level Cervical Spondylosis: A Systematic Review and Meta-Analysis. PLoS One 2012;7:e43407. [Crossref] [PubMed]
- Huang ZY, Wu AM, Li QL, et al. Comparison of Two Anterior Fusion Methods in Two-Level Cervical Spondylosis Myelopathy: A Meta-Analysis. BMJ Open 2014;4:e004581. [Crossref] [PubMed]
- Gao F, Mao T, Sun W, et al. An Updated Meta-Analysis Comparing Artificial Cervical Disc Arthroplasty (CDA) versus Anterior Cervical Discectomy and Fusion (ACDF) for the Treatment of Cervical Degenerative Disc Disease (CDDD). Spine (Phila Pa 1976) 2015;40:1816-23. [Crossref] [PubMed]
- Hu Y, Lv G, Ren S, et al. Mid- to Long-Term Outcomes of Cervical Disc Arthroplasty versus Anterior Cervical Discectomy and Fusion for Treatment of Symptomatic Cervical Disc Disease: A Systematic Review and Meta-Analysis of Eight Prospective Randomized Controlled Trials. PLoS One 2016;11:e0149312. [Crossref] [PubMed]
- Zhang J, Meng F, Ding Y, et al. Hybrid Surgery Versus Anterior Cervical Discectomy and Fusion in Multilevel Cervical Disc Diseases. Medicine (Baltimore) 2016;95:e3621. [Crossref] [PubMed]
- Ma Z, Ma X, Yang H, et al. Anterior Cervical Discectomy and Fusion Versus Cervical Arthroplasty for the Management of Cervical Spondylosis: A Meta-Analysis. Eur Spine J 2017;26:998-1008. [Crossref] [PubMed]
- Zadegan SA, Abedi A, Jazayeri SB, et al. Demineralized Bone Matrix in Anterior Cervical Discectomy and Fusion: A Systematic Review. Eur Spine J 2017;26:958-74. [Crossref] [PubMed]
- Wu TK, Wang BY, Meng Y, et al. Multilevel Cervical Disc Replacement Versus Multilevel Anterior Discectomy and Fusion: A Meta-Analysis. Medicine (Baltimore) 2017;96:e6503. [Crossref] [PubMed]
- Oliver JD, Goncalves S, Kerezoudis P, et al. Comparison of Outcomes for Anterior Cervical Discectomy and Fusion with and Without Anterior Plate Fixation. Spine (Phila Pa 1976) 2018;43:E413-22. [Crossref] [PubMed]
- Oh MC, Zhang HY, Park JY, et al. Two-Level Anterior Cervical Discectomy versus One-Level Corpectomy in Cervical Spondylotic Myelopathy. Spine (Phila Pa 1976) 2009;34:692-6. [Crossref] [PubMed]
- Song KJ, Yoon SJ, Lee KB. Three- and Four-Level Anterior Cervical Discectomy and Fusion with a PEEK Cage and Plate Construct. Eur Spine J 2012;21:2492-7. [Crossref] [PubMed]
- Auffinger BM, Lall RR, Dahdaleh NS, et al. Measuring Surgical Outcomes in Cervical Spondylotic Myelopathy Patients Undergoing Anterior Cervical Discectomy and Fusion: Assessment of Minimum Clinically Important Difference. PLoS One 2013;8:e67408. [Crossref] [PubMed]
- Burkhardt JK, Mannion AF, Marbacher S, et al. A Comparative Effectiveness Study of Patient-Rated and Radiographic Outcome after 2 Types of Decompression with Fusion for Spondylotic Myelopathy: Anterior Cervical Discectomy versus Corpectomy. Neurosurg Focus 2013;35:E4. [Crossref] [PubMed]
- Seng C, Tow BPB, Siddiqui MA, et al. Surgically Treated Cervical Myelopathy: A Functional Outcome Comparison Study between Multilevel Anterior Cervical Decompression Fusion with Instrumentation and Posterior Laminoplasty. Spine J 2013;13:723-31. [Crossref] [PubMed]
- Elsamadicy AA, Adogwa O, Cheng J, et al. Pretreatment of Depression before Cervical Spine Surgery Improves Patients’ Perception of Postoperative Health Status: A Retrospective, Single Institutional Experience. World Neurosurg 2016;87:214-9. [Crossref] [PubMed]
- Elsamadicy A, Adogwa O, Reiser E, et al. The Effect of Patient Race on Extent of Functional Improvement after Cervical Spine Surgery. Spine (Phila Pa 1976) 2016;41:822-6. [Crossref] [PubMed]
- Chaudhary SK, Yu B, Pan F, et al. Manual Preoperative Tracheal Retraction Exercise Decreases the Occurrence of Postoperative Oropharyngeal Dysphagia after Anterior Cervical Discectomy and Fusion. J Orthop Surg (Hong Kong) 2017;25:2309499017731446. [Crossref] [PubMed]
- Kim YS, Park JY, Moon BJ, et al. Is Stand Alone PEEK Cage the Gold Standard in Multilevel Anterior Cervical Discectomy and Fusion (ACDF)? Results of a Minimum 1-Year Follow Up. J Clin Neurosci 2018;47:341-6. [Crossref] [PubMed]
- Moher D, Shamseer L, Clarke M, et al. Preferred Reporting Items for Systematic Review and Meta-analysis Protocols (PRISMA-P) 2015 Statement. Syst Rev 2015;4:1. [Crossref] [PubMed]
- Riley FL. Silicon Nitride and Related Materials. J Am Ceram Soc 2000;83:245-65. [Crossref]
- Bal BS, Rahaman MN. Orthopedic Applications of Silicon Nitride Ceramics. Acta Biomater 2012;8:2889-98. [Crossref] [PubMed]
- Pezzotti G, Marin E, Adachi T, et al. Bioactive Silicon Nitride: A New Therapeutic Material for Osteoarthropathy. Sci Rep 2017;7:44848. [Crossref] [PubMed]
- Pezzotti G, McEntire BJ, Bock RM, et al. In Situ Spectroscopic Screening of Osteosarcoma Living Cells on Stoichiometry-Modulated Silicon Nitride Bioceramic Surfaces. ACS Biomater Sci Eng 2016;2:1121-34. [Crossref]
- Pezzotti G, McEntire BJ, Bock R, et al. Silicon Nitride: A Synthetic Mineral for Vertebrate Biology. Sci Rep 2016;6:31717. [Crossref] [PubMed]
- Webster TJ, Patel AA, Rahaman MN, et al. Anti-Infective and Osteointegration Properties of Silicon Nitride, Poly (Ether Ether Ketone), and Titanium Implants. Acta Biomater 2012;8:4447-54. [Crossref] [PubMed]
- Gorth DJ, Puckett S, Ercan B, et al. Decreased Bacteria Activity on Si3N4 Surfaces Compared with PEEK or Titanium. Int J Nanomedicine 2012;7:4829-40. [PubMed]
- Bock RM, Jones EN, Ray DA, et al. Bacteriostatic Behavior of Surface-Modulated Silicon Nitride in Comparison to Polyetheretherketone and Titanium. J Biomed Mater Res A 2017;105:1521-34. [Crossref] [PubMed]
- Pezzotti G. A Spontaneous Solid-State NO Donor to Fight Antibiotic Resistant Bacteria. Mater Today Chem 2018;9:80-90. [Crossref]
- Anderson M, Bernero J, Brodke D. Medical Imaging Characteristics of Silicon Nitride Ceramic A New Material for Spinal Arthroplasty Implants. In: 8th Annual Spine Arthroplasty Society Global Symposium on Motion Preservation Technology. Miami, FL, 2008:547.
- McEntire BJ, Lakshminarayanan R, Thirugnanasambandam P, et al. Processing and Characterization of Silicon Nitride Bioceramics. Bioceram Dev Appl 2016;6:1000093. [Crossref]
- McEntire BJ, Enomoto Y, Zhu W, et al. Surface Toughness of Silicon Nitride Bioceramics: II, Comparison with Commercial Oxide Materials. J Mech Behav Biomed Mater 2016;54:346-59. [Crossref] [PubMed]
- McEntire BJ, Bal BS, Rahaman MN, et al. Ceramics and Ceramic Coatings in Orthopaedics. J Eur Ceram Soc 2015;35:4327-69. [Crossref]
- Suh PB, Puttlitz C, Lewis C, et al. The Effect of Cervical Interbody Cage Morphology, Material Composition, and Bone Density on Subsidence Risk. J Am Acad Orthop Surg 2017;25:160-8. [Crossref] [PubMed]
- Bock RM, McEntire BJ, Bal BS, et al. Surface Modulation of Silicon Nitride Ceramics for Orthopaedic Applications. Acta Biomater 2015;26:318-30. [Crossref] [PubMed]
- Bock RM, Marin E, Rondinella A, et al. Development of a SiYAlON Glaze for Improved Osteoconductivity of Implantable Medical Devices. J Biomed Mater Res B Appl Biomater 2018;106:1084-96. [Crossref] [PubMed]
- Pezzotti G, Oba N, Zhu W, et al. Human Osteoblasts Grow Transitional Si/N Apatite in Quickly Osteointegrated Si3N4 Cervical Insert. Acta Biomater 2017;64:411-20. [Crossref] [PubMed]
- Pezzotti G, Bock RM, McEntire BJ, et al. In vitro Antibacterial Activity of Oxide and Non-Oxide Bioceramics for Arthroplastic Devices: I. In situ Time-Lapse Raman Spectroscopy. Analyst 2018;143:3708-21. [Crossref] [PubMed]
- Boschetto F, Toyama N, Horiguchi S, et al. In vitro Antibacterial Activity of Oxide and Non-Oxide Bioceramics for Arthroplastic Devices: II. In situ Time-Lapse Fourier Transform Infrared Spectroscopy. Analyst 2018;143:2128-40. [Crossref] [PubMed]
- Boschetto F, Fainozzi D, Marin E, et al. Monitoring Metabolic Reactions in Staphylococcus epidermidis Exposed to Silicon Nitride using in situ Time-Lapse Raman Spectroscopy. J Biomed Opt 2018;23:1-10. [Crossref] [PubMed]
- Mazzocchi M, Bellosi A. On the Possibility of Silicon Nitride as a Ceramic for Structural Orthopaedic Implants. Part I: Processing, Microstructure, Mechanical Properties, Cytotoxicity. J Mater Sci Mater Med 2008;19:2881-7. [Crossref] [PubMed]
- Mazzocchi M, Gardini D, Traverso PL, et al. On the Possibility of Silicon Nitride as a Ceramic for Structural Orthopaedic Implants. Part II: Chemical Stability and Wear Resistance in Body Environment. J Mater Sci Mater Med 2008;19:2889-901. [Crossref] [PubMed]
- Coelho PG, Jimbo R, Tovar N, et al. Osseointegration: Hierarchical Designing Encompassing the Macrometer, Micrometer, and Nanometer Length Scales. Dent Mater 2015;31:37-52. [Crossref] [PubMed]
- Chotai S, Parker SL, Sielatycki JA, et al. Impact of Old Age on Patient-Report Outcomes and Cost Utility for Anterior Cervical Discectomy and Fusion Surgery for Degenerative Spine Disease. Eur Spine J 2017;26:1236-45. [Crossref] [PubMed]
- Omidi-Kashani F, Ghayem Hasankhani E, Ghandehari R. Impact of Age and Duration of Symptoms on Surgical Outcome of Single-Level Microscopic Anterior Cervical Discectomy and Fusion in the Patients with Cervical Spondylotic Radiculopathy. Neurosci J 2014;2014:808596. [Crossref] [PubMed]
- Sielatycki JA, Chotai S, Kay H, et al. Does Obesity Correlate with Worse Patient-Reported Outcomes Following Elective Anterior Cervical Discectomy and Fusion? Neurosurgery 2016;79:69-74. [Crossref] [PubMed]
- Narain AS, Hijji FY, Haws BE, et al. Impact of Body Mass Index on Surgical Outcomes, Narcotics Consumption, and Hospital Costs Following Anterior Cervical Discectomy and Fusion. J Neurosurg Spine 2018;28:160-6. [Crossref] [PubMed]
- Srinivasan D, La Marca F, Than KD, et al. Perioperative Characteristics and Complications in Obese Patients Undergoing Anterior Cervical Fusion Surgery. J Clin Neurosci 2014;21:1159-62. [Crossref] [PubMed]
- Mayo B, Massel DH, Narain AS, et al. The Effect of Smoking Status on Inpatient Pain Scores following Anterior Cervical Discectomy and Fusion. Spine J 2016;16:S359. [Crossref]
- Marawar S, Girardi FP, Sama AA, et al. National Trends in Anterior Cervical Fusion Procedures. Spine (Phila Pa 1976) 2010;35:1454-9. [Crossref] [PubMed]
- Arnold PM, Rice L, Anderson K, et al. Factors Affecting Hospital Length of Stay Following Anterior Cervical Discectomy and Fusion. Evid Based Spine Care J 2011;2:11-8. [Crossref] [PubMed]
- Epstein NE. Predominantly Negative Impact of Diabetes on Spinal Surgery: A Review and Recommendation for Better Preoperative Screening. Surg Neurol Int 2017;8:107. [Crossref] [PubMed]
- Veeravagu A, Cole T, Jiang B, et al. Revision Rates and Complication Incidence in Single- and Multilevel Anterior Cervical Discectomy and Fusion Procedures: An Administrative Database Study. Spine J 2014;14:1125-31. [Crossref] [PubMed]
- Carreon LY, Glassman SD, Campbell MJ, et al. Neck Disability Index, Short Form-36 Physical Component Summary, and Pain Scales for Neck and Arm Pain: The Minimum Clinically Important Difference and Substantial Clinical Benefit After Cervical Spine Fusion. Spine J 2010;10:469-74. [Crossref] [PubMed]
- Parker SL, Godil SS, McGirt MJ. Determination of Minimum Clinically Important Difference in Pain, Disability and Quality of Life After Anterior Cervical Discectomy and Fusion. J Neurosurg Spine 2013;18:154-60. [Crossref] [PubMed]
- MacDowall A, Skeppholm M, Robinson Y, et al. Validation of the Visual Analog Scale in the Cervical Spine. J Neurosurg Spine 2018;28:227-35. [Crossref] [PubMed]
- Kersten RFMR, van Gaalen SM, de Gast A, et al. Polyetheretherketone (PEEK) Cages in Cervical Applications: A Systematic Review. Spine J 2015;15:1446-60. [Crossref] [PubMed]
- Zannikos S, Lee L, Smith HE. Minimum Clinically Important Difference and Substantial Clinical Benefit: Does One Size Fit All Diagnoses and Patients? Semin Spine Surg 2014;26:8-11. [Crossref]