Clinical experience with the use of a spherical bioactive glass putty for cervical and lumbar interbody fusion
Introduction
Degenerative conditions of the spine are common clinical issues that often result in axial mechanical pain as well as neurocompressive radiculopathy of the upper and lower extremities. Following unsuccessful multi-modality non-operative treatments, surgical intervention is often required. The primary goal of most of these procedures is to establish decompression of compromised neural elements. If there is instability or need to correct segmental loss of height and alignment, then a reconstructive fusion procedure is a usual further alternative. Interbody fusion procedures are common and effective means of exacting the above. In this procedure, the degenerated disc is resected and replaced with an interbody spacer. The targeted spinal segment is also stabilized with additional implants that may include an anterior plate construct in the cervical spine or posterior pedicle screw fixation in the lumbar spine. In interbody fusion, the hardware provides initial mechanical support to the spine and helps maintain normal interbody height and multi-axial alignment. However, the long-term goal is to create a durable osseous fusion that bridges the operated vertebral space. This is accomplished by the use of bone grafts in the interbody space and along the posterior spine (when used in the lumbar spine). During the healing process, the bone graft aids in new bone formation and fosters biologic bridging of the intervertebral space with new bone.
Historically, bone grafts were obtained by harvesting bone from a donor site within the patient. This type of autograft bone is typically taken from the patient’s iliac crest or local bone found at the fusion site. However, since autograft is taken from the patient’s own bone, it is both limited in available volume and also has the potential for associated donor site morbidity (1,2). Due to these limitations, a variety of alternatives to autogenous graft have been developed. These alternatives include cadaveric bone grafts (allograft) and a range of synthetic materials such as ceramics, polymers and bioactive glasses. In the synthetic bone graft category, bioactive glasses represent a unique (and uniquely potent) class of products due to several defining material characteristics that are particularly well-suited for bone graft applications (3).
The original form of bioactive glass was discovered and described by Larry Hench, Ph.D. and was specifically formulated as an implantable and resorbable bone graft material (4). Bioactive glasses are typically composed of 4 different oxide materials: SiO2, CaO, Na2O, and P2O5. The main clinically-used bioactive glass formulations include the 45S5 and S53P4 compositions. These glasses have the same oxide composition with slight changes in the relative weight percentage of the components. Biologically and clinically, the glasses have the same properties and perform the same function. Additionally, both glasses have a long history of successful clinical use as a bone graft material in both the spine (5-8) and general orthopedics (9-12).
One of the key properties of bioactive glasses is the ability to form an in vivo layer of bone-like mineral [hydroxy-carbano-apatite (HCA)] on surface of the glass material. As the glass resorbs through in vivo exposure to water, ions from the glass combine with ions in body fluid to form a bioactive surface layer. This bioactive layer enables the glass to chemically bind with adjacent bone and improves the overall ability of the material to support bone growth on its surface (osteoconductivity) (4,13). Originally, the improved bone healing seen with bioactive glasses was attributed to this bioactive property. However, further research revealed that the ionic by-products of glass dissolution had positive effects on the surrounding cells.
In these studies, it was discovered that the dissolution ions resulting from bioactive glass resorption were having a direct positive effect on the local osteoblasts and bone marrow stem cells. Over the years, a variety of studies have shown that 45S5 bioactive glass dissolution products are osteostimulative and improve bone formation by increasing the proliferation, protein expression, and osteoblast differentiation of surrounding stem cells (14-18). Based on this cell stimulation data, the theory behind the bone forming ability of bioactive glass evolved. Although the bioactive property of the glass was confirmed to be effective in promoting bone attachment, it was further recognized that the ion release effect (and resulting cell stimulation) was mainly responsible for the improved bone healing associated with bioactive glasses (13).
Due to the intrinsic bioactive and osteostimulatory properties of bioactive glass, it has become an increasingly favored synthetic bone graft material in clinical applications. Bioactive glass is currently used in a dry particulate form, in a putty form (particles mixed with a moldable carrier), and in a sheet matrix form (particles mixed with a collagen sponge). Although bioactive glass bone grafts have become increasingly popular, some products continue to use an older configuration of the glass that consists of irregular particles with a broad particle size range (typically 32–710 µm). Based on the impact that the bioactive glass dissolution ions have in bone healing, optimization of the particle shape and size can greatly refine and improve the bone healing properties of bioactive glass.
In this context, a new format of bioactive glass bone graft putty is now available (BioSphere® Putty). This product utilizes 45S5 bioactive glass particles with a unique, spherical shape. The spherical glass particles in BioSphere Putty are specifically sized and engineered to selectively control the ion release profile during glass dissolution. By using a uniform spherical shape and a very specific size range of bioactive glass particles, the dissolution and ion release from the particles can be precisely controlled in order to optimize biologic effects and bone healing. Since ion release is directly affected by particle size, an in vivo study was conducted to pinpoint the optimal spherical particle size for ideal bone formation. Results from this early work demonstrated that a bimodal size range that included both small (90–180 µm) and large spheres (355–500 µm) significantly increased the bone forming ability of 45S5 bioactive glass compared to broadly sized, irregular particles (19). In addition, the in vivo testing showed that the spherical shape allowed the particles to pack in a three-dimensional arrangement that resulted in a uniform geometry with a consistent open porosity interposed between the particles. This greatly improved the particle spacing and resulted in fully interconnected bone in-growth throughout the entire implant site.
In order to evaluate the clinical effects of BioSphere Putty on bone healing, a retrospective clinical study was conducted on patients undergoing instrumented spinal fusion procedures.
Methods
Implant materials
BioSphere Putty (Synergy Biomedical, Wayne, PA, USA) consists of 45S5 bioactive glass spheres (80% w/w) mixed with a phospholipid carrier (20% w/w) to aid in intraoperative placement. The spheres have a bimodal size distribution (10% 90–180 µm and 90% 355–500 µm) that has been optimized for bone formation.
Patients and methods
Retrospective clinical data was compiled and reviewed from patients with cervical and lumbar neurocompressive disorders who were surgically treated with an interbody fusion technique. In this case series study, patients were treated by a single surgeon. The patients’ medical records and radiographic imaging were compiled and reviewed for clinical and radiographic outcomes as well as for any documentation of infection, revision surgery, or device related adverse events. Plain film X-ray imaging (static anteroposterior, dynamic lateral flexion/extension) was the primary radiographic imaging technique used. Occasionally, CT imaging was used to determine the integrity of interbody fusion when standard plain film X-rays were not conclusive. Radiographic fusion was determined by the following criteria:
- No motion or <3 degrees of intersegment position change on lateral flexion and extension views;
- Absence of a lucent margin around the implants;
- Absence of interval settling/loss of intervertebral height;
- Structural integrity of the implants and vertebrae themselves;
- Absence of sclerotic changes around the interbody spacer or involving endplates of the vertebrae adjacent to a spacer;
- Readily visible bridging osseous union that is seen around a spacer or posterolateral area without visible cleft, gap, or discontinuity.
A summary designation of union (fusion) or non-union was applied to each patient based on meeting all the criteria above. This assessment was focused on the interbody space and did not assess posterolateral fusion in the patients receiving posterolateral bone grafts.
Additionally, standard peri-operative visual analog scale (VAS) scoring was collected to quantify patient pain at pre-operative (pre-op), 1-year post-operative (1-year post-op), and 2-year post-operative (2-year post-op) timepoints. Average VAS scores were calculated and a t test (paired two sample for means) was used to compared pre-op and post-op averages with a P<0.05. Data was also sorted by the number of operated levels, and the average VAS scores per level were calculated. Additionally, individual patient VAS scores were reviewed to determine the specific change from pre-op to post-op levels. Individual VAS scores changes were classified as a decrease, even (no change), or increase. The individual VAS score change along with the radiographic fusion assessment were used to determine clinical outcome. Successful outcomes were defined by a VAS score decrease at both 1- and 2-year follow-up periods, and the presence of radiographic fusion. Unsuccessful outcomes were defined by an even or increase in VAS scores, or lack of radiographic fusion.
Procedural data
Patient records were reviewed and a total of 248 patients were identified with 1-year or 1- and 2-year follow-up. A summary of the procedural data is shown in Table 1. The patient data review resulted in the identification of 115 cervical fusion cases [anterior cervical decompression and fusion (ACDF)]. Out of these 115 ACDF patients, 23% were single level fusions (n=27), 49% were 2 levels (n=56), 22% were 3 levels (n=25), and 6% were 4 levels (n=7). The patient data review also identified 133 lumbar fusion cases. Lumbar fusion cases were further grouped by procedural approach with 103 patients undergoing transforaminal lumbar interbody fusion procedures (TLIF) and 30 patients undergoing anterior lumbar interbody fusion (ALIF) procedures. Out of the 133 lumbar procedures (TLIF/ALIF), 70% were 1-level (n=93), 29% were 2 levels (n=38) and 2% were 3 levels (n=2).
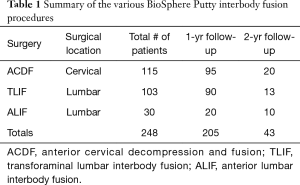
Full table
Surgical technique
Standardized surgical techniques and instrumentation were used in all patients. All patients were implanted with a synthetic PEEK interbody spacer appropriate for the type of surgery being performed (ACDF, TLIF, and ALIF implants). BioSphere Putty was used to fill the graft area of the interbody spacers and also used in the posterolateral spine in lumbar procedures. For the ACDF procedures, BioSphere Putty was used with an integrated interbody plate/spacer implant. BioSphere Putty was used in combination with cancellous allograft in a 1:1 ratio. Three patients (n=3) were implanted with BioSphere Putty only. In the TLIF procedures, a TLIF spacer was implanted along with posterior fixation. A 1:1 volume mixture of BioSphere Putty and local bone autograft was used in the interbody space and the posterolateral space. In the ALIF procedures, an integrated plate/spacer implant was used with no posterior fixation. A bilateral Wiltse approach was utilized for decompression and the placement of posterolateral, inter-transverse bone graft. BioSphere Putty was used in a 1:1 volume combination with cancellous allograft and placed in the interbody space and posterolateral area. One patient had BioSphere Putty only in the interbody space with a 1:1 combination of BioSphere Putty and cancellous allograft in the posterolateral area.
Follow-up
Patients were seen post-operatively at 2 weeks, 6 weeks, 3 months, 6 months, 1 year, and 2 years. Radiographic assessments were made in all patients at the 6-week, 3-month, 6-month, 1-year, and 2-year follow-up periods. Radiographic VAS scores were collected pre-operatively, and at the 1- and 2-year follow-up periods.
Statement of ethics approval
The surgical and data collection methods described in this retrospective study represent the surgeon’s standard practice for treating patients requiring interbody fusion. Therefore, ethics committee approval was not required.
Results
Overall, the results showed excellent clinical outcomes. Plain film X-ray assessments were conducted on A/P and lateral flexion/extension images. Confirmatory CT imaging was required on 5 out of 115 ACDF patients and 7 out of 133 lumbar patients. Radiographically, all patients showed fusions with continuous bridging bone, bone graft endplate integration, and lack of motion and subsidence. Patient chart review showed that there were no adverse events, infections, or graft related complications in any of the patients. VAS results showed a decrease in VAS scores in 93% of the ACDF patients (n=107) and 89% of the TLIF/ALIF patients (n=118). Based on both the radiographic fusion assessment and VAS score analysis, a successful clinical outcome was seen in 93% of the ACDF patients, 88% of the TLIF patients, and 90% of the ALIF patients. A summary of the clinical results is shown in Table 2.
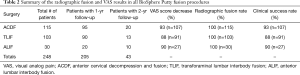
Full table
ACDF results
The patient chart review identified a total of 115 ACDF patients with 95 patients with 1-year follow-up and 20 patients with 1- and 2-year follow-up. ACDF patients were implanted with an integrated plate/spacer and a 1:1 combination of BioSphere Putty with cancellous bone allograft (n=112). A small sub-group of patients (n=3) did receive BioSphere Putty alone with no allograft. All BioSphere only patients resulted in successful clinical outcomes. The number of operated levels in the ACDF group ranged from 1-4 levels with 23% of the patients receiving a 1-level fusion (n=27), 49% receiving a 2-level fusion (n=56), 22% receiving a 3-level fusion (n=25), and 6% receiving a 4-level fusion (n=7). Additionally, there were no post-operative infections or product related adverse events in any of the ACDF patients. Radiographic fusion assessments at 1- and 2-years showed stable osseous fusions in all patients with 5 patients requiring confirmatory CT scans. The radiographic assessment showed evidence of complete fusion in all patients. Bone formation was seen within the cervical spacer graft area and was fully integrated with the inferior and superior cervical endplates. There were no signs of subsidence and flexion/extension films showed no notable motion. In addition to the radiographic data, VAS scores were reviewed for all patients. A summary of the ACDF VAS data is shown in Table 3. At both the 1- and 2-year follow-up periods, the data showed a reduction in the average VAS scores from pre-op levels. The pre-op average for the 1-year patients (n=95) was 6.3 with a 1-year post-op average score of 1.4. This represented a statistically significant reduction in average VAS scores (78% decrease; P<0.5). For the 2-year patients (n=20), the average pre-op level was 6.8 and the average 2-year level was 0.3. This also represented a statistically significant reduction in average VAS scores (96% decrease; P<0.05). The analysis of average VAS score by level showed a similar reduction at 1-year for the 1-, 2-, and 3-level groups (77-80%) and a lower % reduction seen in the 4-level patients (66%). By 2 years, average VAS score decrease ranged from 86% (2-level fusion) to 100% (1- and 4-level fusion). Additionally, an individual analysis was conducted to evaluate the ACDF VAS score change in each patient. In the patients with 1-year follow-up (n=95), 92% of the patients (n=87) showed a decrease in VAS scores while 6% had even scores (n=6) and 2% showed an increase (n=2). For the patients with 2-year follow-up (n=20), 100% of the patients showed a decrease in VAS scores. Based on the criteria of evident radiographic fusion and a VAS score decrease, the overall clinical success rate for the ACDF patients was 93% (107 out of 115 patients).
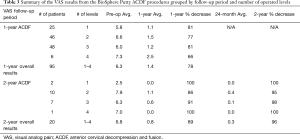
Full table
An example of a successful ACDF case is shown in Figure 1. This shows radiographic images from an ACDF patient who had a 2-level fusion implanted with BioSphere alone. This patient example was chosen to demonstrate the bone generation properties of BioSphere Putty without additional cancellous bone. The results were representative of both the BioSphere alone and BioSphere + cancellous ACDF patients. This ACDF patient presented with two-level cervical degeneration and foraminal stenosis at C5-C7 (pre-op VAS 6.5/10). The patient was surgically treated with a two-level fusion using an integrated plate-spacer filled with BioSphere Putty. Post-operatively, atypical early bone formation through the graft area was evident as early as 6 weeks and was progressing at the 3- and 6-month follow-up periods. By 1-year, the patient was completely asymptomatic (VAS 0/10) and the fusion mass was characterized as complete and without an osseous gap or discontinuity, and with readily visible bridging bone within and around the synthetic interbody spacers. These results were consistent within the overall ACDF group.
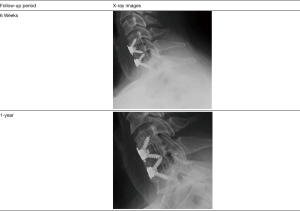
TLIF results
Analysis of the TLIF patients identified a total of 103 patients with 90 patients with 1-year follow-up and 13 with 1- and 2-year follow-up. TLIF patients were implanted with an interbody spacer and posterior fixation. A 1:1 combination of BioSphere Putty and local bone autograft was placed within the spacer and in the posterolateral space. The number of fusion levels in the TLIF patients ranged from 1–3 levels with 69% of the patients receiving a 1-level fusion (n=71), 30% receiving a 2-level fusion (n=31), and 1% receiving a 3-level fusion (n=1). There was one particularly thin elderly female patient who returned to surgery at the one-year post-operative mark for removal of painful, prominent posterior lumbar fixation. During the revision surgery, intra-operative assessment of the fusion confirmed a robust, mature osseous union that was consistent with the radiographic assessment. There were no post-operative infections or product related adverse events in any of the TLIF patients. At the 1- and 2-year follow-up time points, all TLIF patients demonstrated successful radiographic fusion (100% fusion) with 6 patients requiring a confirmatory CT scan. Results showed that all patients had progressed to readily visible radiographic osseous union that was continuous across the operated levels with no signs of subsidence. Flexion/extension analyses indicated stable fusion segments with no notable motion at the fused levels. The VAS scores for the 1- and 2-year TLIF patients are shown in Table 4. At both follow-up periods, the data showed a reduction in the average VAS scores from pre-op levels. The pre-op average for the 1-year patients (n=90) was 6.7 with a 1-year post-op average score of 2.3. This represented a statistically significant reduction in the average VAS scores (66% decrease; P<0.05). For the 2-year patients (n=13), the VAS scores continued to decrease from the average pre-op level of 6.6 to a 2-year average of 1.2 (82% decrease). This also represented a statistically significant decrease (P<0.05). The analysis of average VAS score by level (1–3 levels) for the 1-year TLIF patients showed a 71% decrease for the 1-level patients, 54% decrease for the 2-level patients, and 100% decrease for the 3-level patients. At the 2-year follow-up, a 76% decrease was seen in the 1-level patients, and a 93% decrease was seen in the 2-level patients. There were no 3-level TLIF patients with 2-year follow-up. The individual VAS score analysis evaluated the change in each TLIF patient. In the patients with 1-year follow-up (n=90), 87% of the patients (n=78) showed a decrease in VAS scores while 9% had no change (n=8) and 4% showed an increase (n=4). For the patients with 2-year follow-up (n=13), 100% of the patients showed a decrease in VAS scores. Based on the criteria of evident radiographic fusion and a VAS score decrease, the overall clinical success rate for the TLIF patients was 88% (91 out of 103).
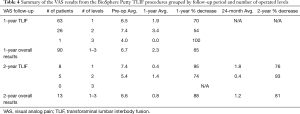
Full table
An example of a successful TLIF case is shown in Figure 2. This patient was diagnosed with baseline degenerative stenosis with compounding large herniated disc at L4/L5 with lumbar stenosis and left sciatica. The initial VAS score was 8/10. The patient was surgical treated with a single level L4/L5 TLIF with posterior fixation. A 1:1 mixture of BioSphere Putty and autograft was used in the interspace and posterolateral area. At the 6-week follow-up, early bone formation was seen in the interspace and was progressing from both endplates. At the 1-year follow-up, radiographic fusion was seen with complete and continuous bone formation across the fusion area without any osseous gaps. The patient had complete resolution of pre-operative symptoms and had a 1-year VAS of 0. These results were consistent with the overall TLIF group.
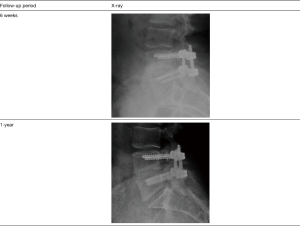
ALIF results
In the lumbar patient group, the ALIF group represented a smaller sub-set with 30 patients total. Out of these patients, 20 patients had 1-year follow-up and 10 had both 1- and 2-year follow-up. ALIF patients were implanted with an integrated plate/spacer with no posterior fixation. Posterolateral decompression and graft placement were conducted through a bilateral Wiltse approach. The interbody space and posterolateral areas were grafted with a 1:1 combination of BioSphere Putty and cancellous allograft. One patient had BioSphere Putty only in the interbody space with a 1:1 combination of BioSphere Putty and cancellous allograft in the posterolateral area. This patient resulted in a successful clinical outcome. The number of operated levels in the ALIF patients ranged from 1–3 levels with 73% of the patients receiving a 1-level fusion (n=22), 23% receiving a 2-level fusion (n=7), and 3% receiving a 3-level fusion (n=1). One patient did return to surgery for an unrelated junction balloon vertebroplasty for an osteoporotic compression fracture. Additionally, there were no post-operative infections or graft related adverse events in any of the ALIF patients. Similar to the TLIF group, all patients in the ALIF group had radiographically fused (100% fusion) and had progressed to stable radiographic osseous unions. One (1) of the ALIF patients required confirmatory CT imaging. Based on the radiographic images, there were no signs of subsidence in the AP X-rays and the flexion/extension images showed no notable motion. The VAS scores for the 1- and 2-year ALIF patients are shown in Table 5. At both follow-up periods, the data showed a reduction in the average VAS scores from pre-op levels. The pre-op average for the 1-year patients (n=20) was 7.0 with a 1-year post-op average score of 2.3. This represented a statistically significant reduction in average VAS scores (67% decrease; P<0.05). For the 2-year patients (n=10), the average pre-op level was 7.0 and the average 2-year level was 1.4. This also represented a statistically significant reduction in average VAS scores (80% decrease; P<0.05). The VAS analysis by level showed an average 1-year decrease of 65% for the 1-level patients (n=14), a 94% decrease for the 2-level patients (n=5), and a 60% increase for a single patient receiving a 3-level fusion (n=1). By 2 years, the average 2-year VAS decrease was 76% for the 1-level patients (n=8) and 100% for the 2-level patients (n=2). There were no 2-year ALIF patients with a 3-level fusion. The individual VAS score analysis evaluated the change in each ALIF patient. At the 1-year follow-up, 85% of the ALIF patients (n=17) showed a reduction in VAS scores while 5% had no change (n=1), and 10% showed an increase (n=2). In the patients with 2-year follow-up, 100% of the patients (n=10) showed a decrease in VAS scores. Based on the criteria of evident radiographic fusion and a VAS score decrease, the overall clinical success rate for the ALIF patients was 90% (27 out of 30).
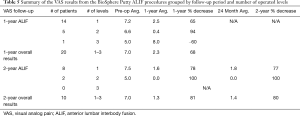
Full table
Figure 3 shows an example of a successful outcome for a patient who had a prior unsuccessful remote laminectomy and L5/S1 fusion followed by hardware removal that was performed by an outside surgeon. During the diagnosis, an MRI of the lumbar spine confirmed severe stenosis at L5/S1, and radiographs confirmed dynamic spondylolisthesis at L5/S1 which was indicative of psuedoarthrosis of the prior attempted fusion procedure. The patient presented with a VAS score of 7. The patient was treated by the study surgeon with a single level L5/S1 ALIF procedure using an integrated plate/spacer with posterolateral grafting through a bilateral Wiltse approach. At the 1-year follow-up, X-rays showed bridging fusion of the operated segment within visible bone spanning the interbody space and well-integrated into the endplates. The patient had near complete resolution of symptoms and a VAS score of 1.
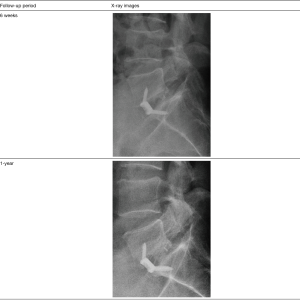
Discussion
The goal of interbody fusion surgery is to facilitate restoration of stability with maintained anatomic alignment at the index vertebral segment. Long-term clinical success in this setting necessarily depends on establishing a stable and robust osseous fusion mass between the vertebrae. While hardware is used to maintain restored alignment and provide mechanical stability during osseous healing, bone graft materials are used to facilitate biologically durable bone formation across the vertebral segment. The 1- and 2-year radiographic data showed that utilization of BioSphere Putty in the described interbody applications resulted in successful osseous fusion in both cervical and lumbar patients. This was seen radiographically in all patients and was independent of whether the Putty was used in combination with autograft or cancellous allograft or by itself. All patients met the radiographic acceptance criteria with visible bone in the fusion area spanning across the vertebral segment, lack of subsidence, and lack of motion in flexion/extension images.
The successful radiographic outcome was further supported by a detailed analysis of the 1- and 2-year VAS scores. One-year average VAS scores showed substantial decreases in pre-operative pain for both ACDF patients (78% decrease) and lumbar patients (66% decrease TLIF/ALIF). By 2-years, the average VAS scores continued to drop with a 96% decrease for the ACDF patients, an 82% decrease for TLIF patients, and an 80% decrease for ALIF patients. The VAS analysis by level showed a general trend in the 1-year patients that the 1-level procedures had larger decreases than the 2-level patients. This would be expected due to the increasing complexity with treating more than one vertebral segment. In patients treated with 3–4 operated levels and with the whole 2-year data groups, there didn’t appear to be any consistent trends of VAS decrease by level due to lower patient sample numbers.
In addition to a VAS level analysis, individual VAS scores for each patient were evaluated to assess whether there was a VAS decrease (positive clinical outcome) or a flat or increase in VAS (negative clinical outcome). This analysis showed that out of the 205 patients (ACDF, TLIF, and ALIF) with 1-year follow-up 88% of the patients (n=181) showed a decrease in VAS scores while 8% showed no change (n=16) and 4% showed an increase (n=8). This individual VAS assessment was combined with the radiographic assessment to determine a clinical outcome for each patient. The data showed a 93% success rate for the ACDF patients, and an 89% success rate for the lumbar patients (TLIF/ALIF).
Overall, the clinical success rates were generally consistent with expected clinical norms for ACDF and lumbar patients. With a 100% radiographic fusion rate, the clinical success rate was directly driven by the change in pre-op to post-op VAS scores. VAS is a semi-quantitative pain score that will capture any pain occurring in the patient (e.g., spinal pain, radicular pain, etc.). In this study, a conservative assessment approach was taken to categorize the small % of patients with no change or an increase in VAS as an unsuccessful outcome in spite of their positive radiographic fusion. This was considered a conservative assessment since certain patients with no change or an increase in VAS can still be satisfied with their outcome (e.g., resolved radicular pain) while still feeling pain in their spine.
Overall, this study demonstrated highly successful outcomes from the use of BioSphere Putty in both cervical and lumbar interbody fusions. While the data was consistently positive with substantial VAS score reductions and successful clinical outcomes, there are inherent limitations with a retrospective case series of this type. In particular, the study only reported on a relatively small number of 2-year patients (43 out of 248 patients). While 1-year data can provide an indication of clinical success, fusion assessments at 2-years can be more accurate due to the maturity of the fusion mass seen in the radiographic images. Additionally, the radiographic fusion rate was determined from the surgeon’s standard follow-up practices that included an X-ray fusion assessment with only the occasional CT, when needed. While plain film X-rays can be effectively used to support other diagnostic assessments or identify issues, direct radiographic measurement of the fusion mass can be challenging (and surely less sensitive than CT in detecting occult asymptomatic pseudoarthrosis). Although the patients in this study with a VAS score decrease were asymptomatic, there may have been some radiographically undetected pseudoarthroses which would have decreased the clinical success rate. The most refined and sensitive method of radiographic fusion assessment is CT imaging. However, this is not commonly used as a routine standard follow-up practice due to patient radiation exposure and hospital cost.
Additionally, this study focused expressly on spinal interbody fusion which is known to be a less challenging bone grafting environment as compared to some other settings (i.e., posterolateral fusion). Over the years, surgeons have been increasingly able to achieve very high levels of fusion due to the available combination of present generation fixation, advanced biologic solutions, and interbody surgical techniques used in well-selected patients. As a result, the high fusion rates seen in this study are consistent with biologically active graft materials such as BMP-2 and bioactive glass products.
Comparatively, studies on the use of a BMP-2 bone graft in lumbar interbody fusion procedures showed similar radiographic fusion rates ranging from 92–100% at 2-years (20-23). In particular, Boden et al. conducted a preliminary study in 11 patients undergoing a single-level anterior lumbar interbody fusion (20). All patients were implanted with a BMP-2 sponge and two threaded anterior lumbar interbody spacers per level. Radiographic fusion assessments showed solid radiographic fusions at the 2-year follow-up (100% fusion rate). Haid et al. conducted a similar study with the BMP-sponge and anterior lumbar threaded spacers (21). In this study, the implants were packed with either a BMP-2 sponge (34 patients) or iliac crest autograft (33 patients). Two-year radiographic assessments showed a 92% fusion for the BMP-2 group and 78% for the autograft group. In a study by Sasso et al., the BMP-2 sponge-threaded spacer combination was compared against a femoral ring allograft (22). One hundred forty patients were randomized into either the BMP-2 group (n=78) or the allograft group (n=62). At the two-year follow-up, the BMP-2 group had a 97% radiographic fusion rate compared to 52% for the allograft group. Slosar et al. also conducted a BMP-2/allograft study in patients undergoing 1–3 level anterior lumbar interbody fusion (23). In this study, femoral ring allografts were used in the interbody space and were implanted with either BMP-2 sponges or allograft chips in the central graft region. At 2 years, the BMP-2 group showed a 100% fusion rate compared to 89% for the allograft chips.
In addition to BMP-2 clinical studies, the results of this study are also consistent with other studies evaluating the effectiveness of bioactive glass products in spinal fusion surgery. Ilharreborde et al. conducted a retrospective study in 88 consecutive patients undergoing spinal fusions to treat adolescent idiopathic scoliosis (5). The study compared a 45S5 bioactive glass granule product against iliac crest autograft at 2-years of follow-up. Although a fusion assessment was not conducted, radiographic measurements quantifying the loss of correction showed that the bioactive glass group had a lower loss of correction (11.0%) than the autograft group (15.5%) which was statistically significant (P=0.025). This was also seen in a similar adolescent scoliosis study by Ameri et al. (6). In this 40-patient retrospective study, 45S5 bioactive glass granules mixed with autograft were compared to an autograft control group. The results showed a 90% fusion rate for the bioactive glass + autograft group and an 85% fusion rate for autograft only.
Conclusions
In the current study, a retrospective review was conducted on spinal interbody fusion patients implanted with a novel, spherical form of bioactive glass (BioSphere Putty). Bioactive glass is a unique material with bioactive and osteostimulatory properties that allow it to take an active role in bone healing. As seen from the BioSphere Putty clinical data in the current study, bioactive glass in a unique, spherical form is an effective and proven bone graft material for spine fusion surgery. Successful patient outcomes were achieved in single to multi-level fusions in both the cervical and lumbar spine. Analysis demonstrated positive results in all patients with reduction in VAS scores and evidence of radiographic fusion. The BioSphere Putty clinical data demonstrates fusion rates that are comparable to other studies evaluating biologically active bone graft products. The consistently good outcomes observed using BioSphere Putty in this present interbody fusion case series serves as a positive foundation for continued clinical application as well as for further and more rigorous investigation of the use of BioSphere Putty in spine surgery.
Acknowledgments
Funding: None.
Footnote
Conflicts of Interest: Dr. Borden is an employee and has ownership interest in the manufacturer of BioSphere Putty (Synergy Biomedical). Dr. Borden has a patent 8,871,235 issued, and a patent 8,506,981 issued. Dr. Westerlund has no conflicts of interest to declare.
Ethical Statement: The authors are accountable for all aspects of the work in ensuring that questions related to the accuracy or integrity of any part of the work are appropriately investigated and resolved.
Data Sharing Statement: No additional data available.
Open Access Statement: This is an Open Access article distributed in accordance with the Creative Commons Attribution-NonCommercial-NoDerivs 4.0 International License (CC BY-NC-ND 4.0), which permits the non-commercial replication and distribution of the article with the strict proviso that no changes or edits are made and the original work is properly cited (including links to both the formal publication through the relevant DOI and the license). See: https://creativecommons.org/licenses/by-nc-nd/4.0/.
References
- Summers BN, Eisenstein SM. Donor site pain from the ilium. A complication of lumbar spine fusion. J Bone Joint Surg Br 1989;71:677-80. [Crossref] [PubMed]
- Younger EM, Chapman MW. Morbidity at bone graft donor sites. J Orthop Trauma 1989;3:192-5. [Crossref] [PubMed]
- Oonishi H, Kushitani S, Yasukawa E, et al. Particulate bioactive glass compared with hydroxyapatite as a bone graft substitute. Clin Orthop Rel Res 1997.316-25.
- Hench LL, Splinter RJ, Allen WC, et al. Bonding mechanisms at the interface of ceramic prosthetic materials. J Biomed Mater Res 1971;5:117-41. [Crossref]
- Ilharreborde B, Morel E, Fitoussi F, et al. Bioactive glass as a bone substitute for spinal fusion in adolescent idiopathic scoliosis: a comparative study with iliac crest autograft. J Pediatr Orthop 2008;28:347-51. [Crossref] [PubMed]
- Ameri E, Behtash H, Mobini B, et al. Bioactive glass versus autogenous iliac crest bone graft in adolescent idiopathic scoliosis surgery. Acta Medica Iranica 2009;47:41-5.
- Rantakokko J, Frantzén J, Heinanen J, et al. Posterolateral spondylodesis using bioactive glass S53P4 and autogenous bone in instrumented unstable lumbar spine burst fractures. A prospective 10-year follow-up study. Scand J Surg 2012;101:66-71. [Crossref] [PubMed]
- Frantzén J, Rantakokko J, Aro HT, et al. Instrumented spondylodesis in degenerative spondylolisthesis with bioactive glass and autologous bone: a prospective 11-year follow-up. J Spinal Disord Tech 2011;24:455-61. [Crossref] [PubMed]
- Heikkilä JT, Kukkonen J, Aho AJ, et al. Bioactive glass granules: a suitable bone substitute material in the operative treatment of depressed lateral tibial plateau fractures: a prospective, randomized 1-year follow-up study. J Mater Sci Mater Med 2011;22:1073-80. [Crossref] [PubMed]
- Pernaa K, Koski I, Mattila K, et al. Bioactive glass S53P4 and autograft bone in treatment of depressed tibial plateau fractures - a prospective randomized 11-year follow-up. J Long Term Eff Med Implants 2011;21:139-48. [Crossref] [PubMed]
- Sun JY, Hao SC, Sun RB, et al. Treatment of high-energy tibial shaft fractures with internal fixation and early prophylactic Novabone grafting. Orthop Surg 2009;1:17-21. [Crossref] [PubMed]
- Lindfors NC, Heikkilä J, Koski I, et al. Bioactive glass and autogenous bone as bone graft substitutes in benign bone tumors. J Biomed Mater Res B Appl Biomater 2009;90:131-6. [PubMed]
- Hench LL. The story of bioactive glass. J Mater Sci Mater Med 2006;17:967-78. [Crossref] [PubMed]
- Xynos ID, Hukkanen MV, Batten JJ, et al. Bioactive glass 45S5 stimulates osteoblast turnover and enhances bone formation in vitro: implications and applications for bone tissue engineering. Calcif Tissue Int 2000;67:321-9. [Crossref] [PubMed]
- Xynos ID, Edgar AJ, Buttery LD, et al. Gene expression profiling of human osteoblasts following treatment with the ionic products of bioactive glass 45S5 dissolution. J Biomed Mater Res 2001;55:151-7. [Crossref] [PubMed]
- Jell G, Stevens M. Gene activation by bioactive glasses. J Mater Sci: Mater Med 2006;17:997-1002. [Crossref] [PubMed]
- Bosetti M, Zanardi L, Hench L, et al. Type I collagen production by osteoblast-like cells in contact with different bioactive glasses. J Biomed Mater Res A 2003;64:189-95. [Crossref] [PubMed]
- Gao T, Aro H, Ylanen H, et al. Silica-based bioactive glasses modulate expression of bone morphogenetic protein-2 in SAOS-2 osteoblasts in vitro. Biomaterials 2001;22:1475-83. [Crossref] [PubMed]
- Synergy Biomedical. Internal study on file.
- Boden SD, Zdeblick TA, Sandhu HS, et al. The use of rhBMP-2 in interbody fusion cages. Definitive evidence of osteoinduction in humans: a preliminary report. Spine (Phila PA 1976) 2000;25:376-81. [Crossref] [PubMed]
- Haid RW Jr, Branch CL Jr, Alexander JT, et al. Posterior lumbar interbody fusion using recombinant human bone morphogenetic protein type 2 with cylindrical interbody cages. Spine J 2004;4:527-38; discussion 538-9. [Crossref] [PubMed]
- Sasso RC, Kitchel SH, Dawson EG. A prospective, randomized controlled clinical trial of anterior lumbar interbody fusion using a titanium cylindrical threaded fusion device. Spine (Phila Pa 1976) 2004;29:113-22. [Crossref] [PubMed]
- Slosar PJ, Josey R, Reynolds J. Accelerating lumbar fusions by combining rhBMP-2 with allograft bone: a prospective analysis of interbody fusion rates and clinical outcomes. Spine J 2007;7:301-7. [Crossref] [PubMed]