Two-year results of a double-blind multicenter randomized controlled non-inferiority trial of polyetheretherketone (PEEK) versus silicon nitride spinal fusion cages in patients with symptomatic degenerative lumbar disc disorders
Introduction
Chronic low-back pain (LBP) is a condition that adversely affects the quality of life for millions of individuals worldwide (1). The resulting healthcare burden for LPB in industrialized societies is estimated to be between 0.2% to 3.9% of their gross domestic products (GDP) (2-4). While the etiology of LBP varies (i.e., stenosis, herniation, facet degeneration, spondylolysis, and spondylolisthesis), surgical intervention is recommended only after conservative management has failed (5). Intervertebral fusion is considered to be the standard of care, with a 30+ year record of relieving pain from these symptomatic disorders (6-10). Historically, bone grafts were used to facilitate fusion, but they were associated with pseudarthrosis, collapse, and donor site morbidity (11,12). Today, synthetic interbody cages have largely supplanted allogenic spacers in restoring sagittal alignment and maintaining disc and foraminal height while facilitating bony fusion via the inclusion of autograft and other bone substitutes (13,14). There are several synthetic cage materials including titanium (Ti), polyetheretherketone (PEEK), tantalum (Ta), and silicon nitride (Si3N4) (15). Of these, Ti and PEEK implants are commonly used by surgeons based on their positive clinical performance (16).
With the development of the Bagby and Kuslich (BAK) cage in the 1980s and 1990s, Ti became one of the first biomaterials to be used in spinal fusion surgery (17). Ti is effective in osseous integration due primarily to its oxide surface layer which promotes osteoblast adhesion and proliferation (18), but it is X-ray radiopaque and produces imaging artifacts on computed tomography (CT) and magnetic resonance imaging (MRI) (15). Its elastic modulus is also seen as a limitation because it is significantly higher (E =105 to 120 GPa) than either cancellous or cortical bone (E =5.0 to 25.8 GPa) (19). In contrast, PEEK’s favorable elastic modulus (E =4.0 GPa) (19) and radiolucent (15) nature have made it the preferred biomaterial for interbody fusion, having high arthrodesis rates and good clinical outcomes (20,21). However, PEEK also has its disadvantages. Its petrochemical nature is prone to bacterial colonization and biofilm formation, and its hydrophobic surface discourages direct appositional bone growth by inhibiting protein adsorption and cell adhesion. This leads to the formation of a fibrous layer around PEEK implants (22-27). Even so, a recent meta-analysis found that both Ti and PEEK implants were equally effective in spine fusion although Ti has a slightly increased risk for subsidence (28). However, other studies suggest that size and geometry (i.e., footprint) determine mechanical stability and subsidence risk rather than elastic properties (29-31).
Cage design has also focused on combining two materials to optimize fusion (13). For example, Chong et al. demonstrated early osseointegration and fusion by using a composite device consisting of a PEEK body with Ti-coated endplates (32). Another study suggested that porous PEEK was associated with improved osteogenic differentiation in vitro and greater implant fixation in vivo when compared to Ti-coated PEEK cages (33). Hydroxyapatite-coated PEEK has also been shown to improve osseous integration (34,35). Despite these innovations, there are few differences in clinical outcomes and fusion rates for PEEK, Ti, or other materials including carbon-fiber-reinforced interbody cages (29). This likely explains why PEEK remains the favored biomaterial for spinal fusion (36).
Like other implant materials, Si3N4 also has advantages and limitations. It is a non-oxide ceramic with high strength, toughness (37-39), and elastic modulus (E =296 to 313 GPa) (19). Its non-ferrous nature minimizes scatter and artifacts on CT and MRI, and it is partially X-ray radiolucent (40). Due to its surface chemistry, it decreases bacterial adhesion as compared to PEEK and Ti (23,24,41) while concurrently upregulating osteogenic activity. Its mechanical, chemical, and osteoconductive qualities have been extensively studied (42-46). While Si3N4 may appear to be novel, it has one of the longest histories of any spinal fusion biomaterial. It was first used in a human clinical trial for lumbar fusion beginning in 1986, with follow-ups reported at 15 and 30+ years (47,48). Although the early design and material composition of these implants were suboptimal, modern data equally attest to the material’s biocompatibility, safety, and efficacy (48). Pre-clinical and clinical studies have also demonstrated the effectiveness of Si3N4 as spinal spacers, particularly in the cervical spine (27,49-53).
The present study reports on the 2-year clinical and radiographic outcomes of a prospective randomized controlled non-inferiority clinical trial that compared Si3N4 and PEEK intervertebral cages implanted in patients with symptomatic degenerative lumbar disc disorders (37). The purpose of this investigation was to determine if devices made from Si3N4 have a similar clinical performance to PEEK. The research hypothesis was that Si3N4 implants would not be inferior to PEEK cages. This article was prepared per the CONSORT reporting checklist (54,55). (available at http://dx.doi.org/10.21037/jss-20-588).
Methods
Study design
The study was designed in concordance with the declaration of Helsinki (as revised in 2013) as a non-inferiority multicenter 100 patient (50 per cohort) prospective randomized controlled clinical trial where both the observer and patient were blinded. The study protocol was previously published (37) and a summary is available at www.clinicaltrials.gov (Identifier NCT01557829). It was approved by the Medical Research Ethics Committee United, Nieuwegein, the Netherlands (Verenigde Commissies Mensgebonden Onderzoek, https://www.ccmo.nl/). Patients (18–75 years) presenting with chronic LBP and disc degeneration of Pfirmann grade III or higher and/or isthmic or degenerative spondylolisthesis grade I or II were included. Patients were excluded for prior failed fusion at the same level, more than two symptomatic levels that required fusion, degenerative scoliosis, spondylolisthesis greater than grade II, osteoporosis, active or prior infection at the surgical site, neoplasm, psychiatric, or mental disorders, age >80 years, and insufficient Dutch language skills. Patients were randomly allocated to one of the two groups at the time of surgery using a centralized 24-h online computerized randomization system (Sealed Envelope, LTD, London, UK). Patients and clinical observers were blinded for the assigned treatment during the 24-month follow-up. Clinical and radiographic assessments were performed at 3, 6, 12, and 24 months.
Surgical procedure
Single- or double-level transforaminal lumbar interbody fusion with pedicle screw fixation was performed with either an oblique PEEK or Si3N4 cage (Phantom™ PLIF and Valeo® OL, respectively, CTL-Amedica, Dallas, TX, USA). Representative photographs of the two cages are shown in Figure 1A,B. The Si3N4 cage had a lordosis of 0° whereas the PEEK implant had 6° of lordosis. In brief, after adequate exposure and placement of pedicle screws, a facetectomy was performed followed by an appropriate decompression of the symptomatic site. The disc space was cleared of disc material and the endplates were prepared. Cages were packed with locally harvested autograft from the lamina and facet joints. A single oblique cage was then placed in the disc space. Final fixation of the pedicle screws and rods was performed under compression. Patients were mobilized on the first day after surgery without orthotics.
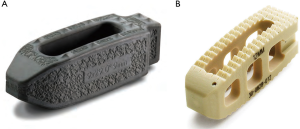
Outcome measures
Clinical assessment
The primary outcome measure was the average improvement in the validated Dutch version of the Roland-Morris Disability Questionnaire (RMDQ, 0–24-point scale) between the two treatment groups, with a higher score indicating more severe disability (56). Secondary outcome measures included scores from the generic quality of life questionnaires SF-36 (57), Oswestry Disability Index (ODI, 0–50-point scale) (58), leg and back pain Visual Analog Scales (VAS, 0 to 100 mm) (59), and the Likert score (7-point scale) for perceived recovery by the patient and surgeon (60); Likert scores indicating complete recovery and almost complete recovery were considered good outcome measures (61). A neurological examination was also conducted at 3-, 6-, 12-, and 24-month follow-ups.
Radiological assessment
Each patient’s fusion status was evaluated according to the criteria mentioned by Burkus et al. (62,63), which included: (I) the presence of bridging bone on a CT scan (Siemens Sensation 16, Malvern, PA, USA, 3.0-mm slice) at 12- and 24-month follow-ups; (II) disc height and angular changes in segmental alignment on plain lateral radiographs at 24-month follow-up; and (III) an assessment of device-host interface on a CT scan at 12-month follow-up (63). Standing anterior-posterior (AP) and lateral radiographs were collected at 3-, 6-, 12-, and 24-month follow-ups. Lateral X-ray radiographic images at 24-month follow-up are shown in Figure 2A,B for both implant types. Note that both images show solid fusion with bone bridging between the endplates. Average disc heights were determined as the mean of the anterior and posterior measurements. Subsidence was defined as a loss of >1 mm in average disc height. Also, at 12-month follow-up, a CT scan (Siemens Sensation 16, Malvern, PA, USA, 3.0-mm slice) was collected to monitor bony bridging. At 24 months, additional flexion/extension lateral radiographs were obtained to monitor angular motion. Fusion was defined as an angular motion of <2° and a translational motion of <0.5 mm. Each level was analyzed separately for patients with two-level fusion. All radiological analyses were performed by an independent organization (Medical Metrics, Houston, TX, USA).
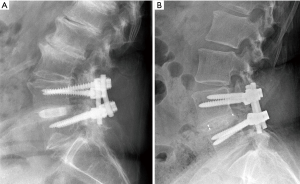
Statistical analyses
Primary efficacy analysis
The primary statistical outcome was to demonstrate that cages made of Si3N4 were non-inferior to PEEK as measured by the average improvement in RMDQ scores at 12-month follow-up. The minimum clinically important difference (MCID) for the RMDQ was a priori set at 2.6 points on a scale of 24 based on an equal number of 50 patients in each cohort (37,64). The analytical method was based on a mixed-effects model for repeated measurements (MMRM). This model included the treatment (i.e., type of cage) and operative center as independent variables, and the baseline RMDQ (fixed effect) and patients (random effect) as covariates. An unstructured covariance matrix was assumed to model the “within-patient variance” and an estimation was performed by restricted maximum likelihood (65). The upper bound of a one-sided 97.5% confidence interval for the difference in the mean change from baseline to 12-month follow-up RMDQ scores for the two cages (i.e., Si3N4 and PEEK) was used to assess non-inferiority. Non-inferiority was to be demonstrated only if the upper boundary of the confidence interval did not exceed 2.6 of the RMDQ score—the smaller the RMDQ score, the better. Sensitivity analysis was used to assess the impact of dropouts. This analysis was conducted using the Last Observation Carried Forward (LOCF) imputation. The analytical and estimation method for the sensitivity analyses used the same MMRM with the same terms as the primary efficacy analysis. Additionally, a post hoc analysis was conducted because patient follow-up losses and RMDQ standard deviations were larger than protocol assumptions. These variances compromised the power of the original study from 90% to 50%. Consequently, as explained in the “Results” and “Discussion” sections, the upper boundary of the confidence interval was increased to 4.0 from 2.6.
Secondary efficacy analyses
The secondary efficacy outcomes (i.e., ODI, leg and back VAS, Physical and Mental Function SF-36, and radiological measurements) were analyzed using the same MMRM adjusting for baseline values. Dichotomous outcomes based on the Likert scales for patient and surgeon perception were compared between treatment groups using Chi-Squared tests for proportions.
Statistical analyses were performed using either RStudio Version 3.1-131 (Boston, MA, USA), SAS 9.4 Proc Mixed (SAS Institute, Cary, NC, USA), or MedCalc Version 18.6 (Ostend, Belgium). Post hoc analyses were assisted with the use of PS Power and Sample Size Calculations software (Vanderbilt University, Nashville, TN, USA, Version 3.0, 2009, http://biostat.mc.vanderbilt.edu/wiki/Main/PowerSampleSize).
Results
Patient accountability and baseline characteristics
After receiving informed consent, 101 patients were originally included in the study between February 2012 and January 2015 (49 and 52 for Centers 1 and 2, respectively). Eight patients were subsequently excluded due to protocol violations (i.e., no pre-operative randomization, proof of osteoporosis after inclusion, or age >80 at the time of surgery) or by the cancellation of surgery by the patient after inclusion. Of the remaining 92 patients (46 each per center), 48 were randomized for PEEK and 44 for Si3N4. Most patients had symptoms of LBP combined with radicular leg pain (69 out of 92 patients). As shown in Table 1, baseline characteristics were evenly distributed between the two treatment arms without statistically significant differences. Eight patients in the Si3N4 group received two-level fusion compared with five patients in the PEEK cohort. There were no crossovers. At 24 months, 13 additional patients were not evaluable (14.1%) due to either unrelated trauma, revision surgery, epidural steroid injections, or refusal of treatment. A patient accountability flow-chart is provided in Figure 3.
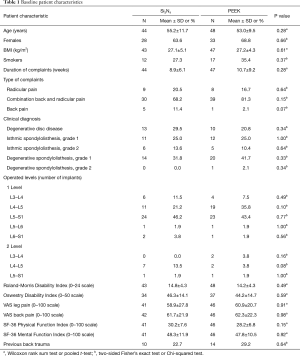
Full table
Perioperative results
Perioperative data are provided in Table 2. There were no differences in the length of hospital stay between the two groups. Average operative time and blood loss for the Si3N4 group were significantly greater than the PEEK cohort because insertion of the Si3N4 cages represented a new implantation procedure for the participating surgeons and hospitals. There was also a slightly higher rate of perioperative complications for the Si3N4 group, although the differences were not statistically significant.
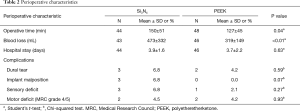
Full table
Primary clinical outcome
The primary clinical outcome for the two cohorts was their average improvement in RMDQ scores. As shown in Figure 4, both treatment arms showed reductions in disability during the 24-month study. Mean improvements for either group were highly statistically significant at every time point (P<0.001). Although RMDQ scores between the groups at each follow-up were not significantly different (P>0.12–0.19), the PEEK group consistently had lower scores; but a trendline analysis indicated that there was no difference in the rate of improvement from pre-operative to 24 months between the two groups, neither were there any statistical differences based on patient diagnosis. At each time point, the MCID between the PEEK and Si3N4 groups was less than the non-inferiority margin of 2.6 points. Using the a priori criteria for non-inferiority (i.e., n=50 patients in each cohort, RMDQ standard deviations of ≤4.0, and a one-sided upper confidence interval of 2.5%), the null hypothesis that Si3N4 is non-inferior to PEEK could not be established. This outcome is graphically shown in Figure 5. The confidence interval (indicated by the error lines) exceeds the non-inferiority margin of 2.6 at each follow-up period. However, this conclusion is perhaps erroneous because the power of the study was compromised due to patient losses and RMDQ standard deviations which exceeded protocol assumptions. A post hoc analysis, which accounted for patient fallout and actual RMDQ standard deviations, is provided in Table 3. At the primary endpoint of 12-month follow-up, the power to detect a discernable difference using the original 2.6 non-inferiority margin was only ~50%. Consequently, a revised non-inferiority margin of 4.0 was determined based on an additional review of the literature (64,66-74) (see “Discussion” section). Indeed, based on this revised margin, it was concluded that Si3N4 was non-inferior to PEEK in the context of this study.
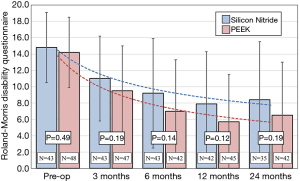
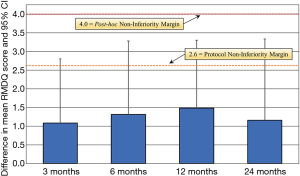
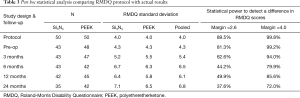
Full table
Secondary clinical outcomes
Secondary clinical outcomes are given in Table 4. There were no significant differences in ODI, leg and back VAS pain scores, and SF-36 Physical or Mental Function indices at each of the follow-up periods. Also, follow-up VAS scores were not statistically different for the two implant materials when correlated with patient diagnosis. Likert scores for surgeon and patient-perceived outcomes are provided in Table 5. Although both physicians and subjects reported generally better recovery rates for the PEEK group at each follow-up time point, these differences did not reach statistical significance.
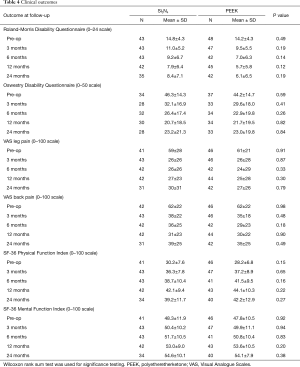
Full table
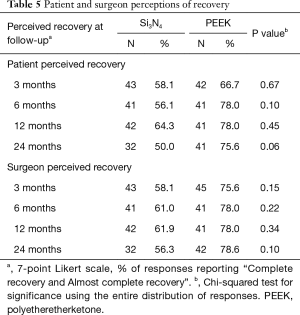
Full table
Radiological outcomes
X-ray radiography at 12- and 24-month follow-ups and CT scans at 12 months were used to assess for segmental motion and fusion. The radiographic data are provided in Table 6. There were no differences in relative intervertebral disc heights or movements for 12- or 24-month follow-ups between the two implant groups. Neither subsidence nor migration were notable issues. The flexion-extension X-ray images obtained at 24-month follow-up also showed no significant fusion differences between the two cohorts using two generally accepted assessment criteria—the original protocol (37) and FDA guidance (75). The CT images at 12-month follow-up indicated that 42% of the PEEK and 57% of the Si3N4 implants exhibited bone bridging between the endplates (P=0.13). Sagittal and coronal views for a Si3N4 implant are shown in Figure 6A,B, respectively. Because the PEEK implants were radiotransparent, the interface between the endplates and the implants could not be adequately ascertained. An assessment of the device-bone interface (i.e., radiolucency or osseous integration) was therefore deemed to be unreliable and not incorporated into the radiological analyses.
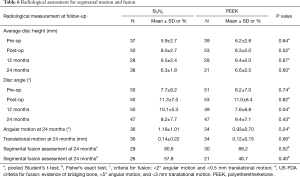
Full table
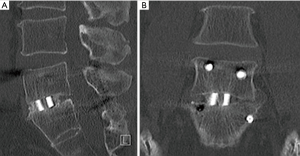
Complications and revisions
There were 14 revisions during the 24-month follow-up (15.2%). Details are provided in Table 7. In the PEEK group, four patients (8.3%) were revised at between 5 and 14 months following their index surgeries due to decompression and adjacent level surgeries. This compares to ten patients in the Si3N4 cohort (22.7%) that were also revised up to 20 months post-operatively for factors related to implant positioning, a neurological disorder, pseudarthrosis, adjacent level procedures, screw malfunctions, and a cage non-union. Many of the repeat surgeries in both groups were performed due to adjacent level disease. Although reoperations were higher for the Si3N4 cohort, the difference was not statistically significant using Fisher’s exact test (P=0.08).
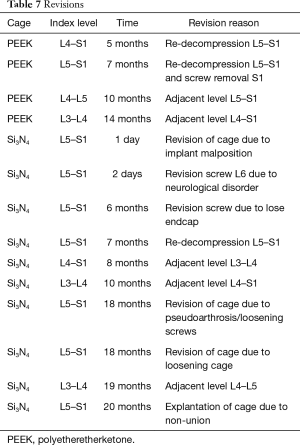
Full table
Discussion
This trial was designed to compare the clinical and radiological outcomes for two spinal cage materials, Si3N4 and PEEK, in patients undergoing fusion surgery due to intractable lumbar back or leg pain (37). The overall results indicated that patients treated with either cage material had similar outcomes for disability, pain, and fusion. In particular, the RMDQ improvements of this trial were similar to results from other spinal fusion studies (66,67,73), thereby reflecting good 1- and 2-year clinical outcomes for both the Si3N4 and PEEK groups. The other post-operative improvement scores from the study were also consistent with reported literature values for other studies using PEEK cages, ranging from 24 to 36 for VAS back pain, and 26 to 42 for VAS leg pain (76,77). In the present trial, both treatment groups showed an average of more than 30 points of improvement for both VAS back and leg pain after 12-month follow-up. Average ODI changes found in the literature range from 9 to 20 (21,76,78) while the ODI improvements in this study after 12 months were more than 12.5 points for both treatment groups. The fact that the average improvement scores for VAS and ODI in this trial are comparable with data found in the literature (specifically for lumbar interbody fusion using PEEK cages) provides confidence in the validity of the RMDQ measurements as well. Lastly, the fusion results observed in the current study were also found to be similar to values reported in a recent systematic review (79).
Primary outcome
In this study, it was hypothesized that Si3N4 would be non-inferior to PEEK as measured by a differential improvement in RMDQ scores at 12-month follow-up of no more than 2.6 points (i.e., the non-inferiority margin). There was insufficient evidence to conclude that Si3N4 was non-inferior based on the original protocol assumptions. However, the actual study significantly deviated from the protocol, particularly for the number of patients in each cohort and the RMDQ standard deviations. The 2.6-point non-inferiority margin would have been adequate for patient populations of 50 in each group and RMDQ standard deviations of ≤4, but the power to reject the research hypothesis was reduced from a planned 90% to approximately 50% at 12-month follow-up due to variances in these suppositions (cf., Table 3). The protocol non-inferiority margin was originally set to the MCID based on studies by Robertson and Plank (66), Scheufler et al. (67), Patrick et al. (68), Roland and Fairbank (69), and Ostelo et al. (64). In delving into other relevant literature, it was concluded that the 2.6-point non-inferiority margin may have been inappropriate. For instance, in a two-paper series, Stratford et al. (70) and Riddle et al. (71) examined 226 patients subjected to treatments for LBP using the RMDQ index. They found that the minimum detectable difference between pre- and post-treatments varied based on the patient’s initial RMDQ score. They concluded that clinically important changes in the RMDQ were 2 (for an initial score of 0 to 8), 4 (for an initial score of 5 to 12), 5 (for an initial score of 9 to 16), 8 (for an initial score of 13 to 20), and 8 (for an initial score of 17 to 24). In no instance did they recommend a tight margin for initial moderate to severe scores (i.e., RMDQ >12). In a separate publication, Stratford et al. utilized the RMDQ to study 60 outpatients with lower back pain (74). They found that the minimum level of detectable change was 4 to 5 points at a 90% confidence level, which is significantly greater than the originally specified non-inferiority margin of the current study. An additional lumbar interbody fusion study by Ohba et al. reported RMDQ standard deviations which were substantially larger than 4 (i.e., range of 5.4 to 5.9 at 12-month follow-up) for a two-cohort study with similar patient populations (73). Finally, Brouwer et al. used RMDQ as the primary outcome measure in a non-inferiority comparison of percutaneous laser disc decompression and conventional microdiscectomy (72). Their study had similar RMDQ scores and standard deviations as the present study, and they selected a value of 4 as the non-inferiority margin for determining a MCID. These additional references coupled with a post hoc power analysis suggest the current study was not adequately powered for a non-inferiority margin as small as 2.6, and further group differences in means somewhat larger than 2.6 are also likely associated with a group difference in means that is not clinically meaningful. Consequently, as shown in Figure 5 and Table 3, a revised non-inferiority margin of 4.0 appears clinically justifiable; and under this assumption, the non-inferiority of the Si3N4 versus PEEK cages was affirmed.
Perioperative outcomes
A significant difference was found in operative time and blood loss in favor of the PEEK cohort (i.e., 127 versus 150 min, and 319 versus 473 mL, respectively). The greater amount of blood loss was directly linked to the longer operative time for the Si3N4 group. However, this result is skewed due to an outlier for one patient in the Si3N4 group whose blood loss was 1,700 mL. The difference in operative time can also be partially explained by a higher number of 2-level procedures in the Si3N4 cohort compared to PEEK (i.e., 8 versus 5). Additionally, the operative time for the Si3N4 cages was increased due to the surgeons rotating the Si3N4 cages within the disc space during implantation—an unfamiliar technique that was not performed with the PEEK devices. During insertion, a Si3N4 cage fractured in each of two patients which extended the duration of their surgeries. However, after replacing these implants, no additional fractures occurred. Given these issues, the data indicate an average of 10 min less operative time between the first and second halves of the Si3N4 patients. This was not the case for the PEEK cages, as they had already been in use for several years in both participating hospitals before the start of the trial. Other perioperative complications were evenly distributed over the length of the study. There was no statistically significant difference in complication rates between the two participating centers.
Radiological outcomes
There is considerable controversy in the scientific literature as to when a lumbar segment is fused (62,80,81). Some practitioners argue that radiographic motion analyses are at best inconclusive. They favor operative assessments. Others believe that radiography can be effectively used, and various criteria of angular and translational motions have been proposed. Still, others suggest that a combination of radiographic motion coupled with the presence of anterior bridging bone (i.e., the “sentinel sign”) on the radiograph along with no radiolucencies at the superior or inferior surfaces of the implant is the best method. In this study, the PEEK implants were radiotransparent and the interface between the endplates and implants could not be adequately ascertained. An assessment of either radiolucency or osseous integration for the PEEK devices was deemed unreliable and therefore not incorporated into the analyses. However, several other criteria were used. Bony bridging (i.e., between the superior and inferior endplates) was measured using CT scans at 12 months post-operative. Disc height measurements were also added for an analysis of potential subsidence. No significant differences were noted in the amount of bony bridging or subsidence between the two implant groups. Segmental motion, from flexion/extension X-ray radiographs, was also used to analyze for fusion. Both the original protocol criteria (i.e., <2° of angular and <0.5 mm of translational motion) (37) and the US FDA criteria (i.e., evidence of bridging bone, <5° angular and <3 mm translation motion) (75) were employed to assess for fusion. The protocol criteria indicated that ~81% of Si3N4 and ~88% for PEEK segments were fused, which is consistent with a systematic review for transforaminal lumbar interbody fusion (79) (i.e., ~76% to 100%); whereas the FDA criteria showed ~58% of the Si3N4 and 47% of PEEK segments were fused (cf., Table 6). However, a technically successful fusion does not necessarily equate to the same clinical outcome because vertebral stability may occur before it is radiographically evident (82). There is only weak evidence to suggest that bony fusion correlates with good clinical outcomes (63,83). A sub-analysis of the data showed that boney bridging is not an indicator of RMDQ improvement. Therefore, in designing the study, our primary objective was to test for non-inferiority as measured by RMDQ scores.
Revisions
Although not statistically significant, there were more revisions within the Si3N4 group than the PEEK cohort (i.e., 10 versus 4). Excluding the five revisions which were strictly associated with adjacent level disease, the rate due solely to perioperative complications (9.8%) was similar to other reported transforaminal lumbar studies (84-86). Also, as described earlier, a learning curve may have impacted the higher than anticipated revision rate for the Si3N4 cages whereas the PEEK devices have been used by the participating surgeons for many years.
Limitations
The design of this trial had several limitations. The use of a single oblique cage was chosen to allow for more accurate fusion measurements using X-ray and CT imaging. However, a single cage is mechanically unstable compared to two parallel cages (87). This could have biased the results and it also helps to explain the overall revision rate of 15.2% and revisions due solely to perioperative complications (9.8%). Also, from a retrospective point of view, the clinical outcomes for the two cohorts may have been more balanced if the blinded randomization had considered the pre-operative RMDQ and pain scores. Although both cohorts experienced significant improvements in their disability and pain scores during the 24 months, the trendline data suggests that those pre-operative patients with the highest disability and pain scores maintained their relative position at each follow-up time point (cf., Figure 4). It appears that in randomizing the population, more of these types of patients were apportioned to the Si3N4 group than the PEEK cohort.
Conclusions
Similar clinical outcomes and overall recovery rates were reported after transforaminal lumbar interbody fusion using either PEEK or Si3N4 cages in patients with degenerative lumbar disc disorders. There was no significant difference in clinical measures during the 24 months of active follow-up. The primary RMDQ non-inferiority hypothesis for the Si3N4 cages could not be demonstrated utilizing the original protocol assumptions (i.e., n=50 patients in each cohort and an RMDQ standard deviation of ≤4) due to significant patient fallout (n=43 for Si3N4 and 45 for PEEK) and higher than expected RMDQ standard deviations (SD =6.4 for Si3N4 and SD =5.8 for PEEK) at 12-month follow-up. These variances reduced the power of the study from a planned value of 90% to only 50%. After an additional review of the literature and a post hoc analysis, the non-inferiority margin was revised from 2.6 to 4.0; and under this assumption, the non-inferiority of the Si3N4 cages was affirmed. In conclusion, both the Si3N4 and PEEK cages were determined to provide comparable clinical outcomes for lumbar spinal fusion.
Acknowledgments
Appreciation is expressed to the surgeons and staffs of the Clinical Orthopedic Research Centermidden Nederland (CORC-mN), the Department of Orthopedics, Diakonessenhuis Utrecht/Zeist, the Department of Orthopedics, University Medical Center Utrecht, Utrecht University, the Department of Orthopedics, Acibadem International Medical Center, Amsterdam, the Department of Neurosurgery, Medical Center Haaglanden, The Hague, and the Julius Centre for Health Sciences and Primary Care, University Medical Center Utrecht, Utrecht University, The Netherlands for the performance of the study.
Funding: This study was funded by Amedica Corporation (now SINTX Technologies, Inc.), Salt Lake City, UT, USA. The research departments of the participating centers were compensated for their time and materials in completing this study.
Footnote
Reporting Checklist: The authors have completed the CONSORT reporting checklist. Available at http://dx.doi.org/10.21037/jss-20-588
Data Sharing Statement: Available at http://dx.doi.org/10.21037/jss-20-588
Conflicts of Interest: All authors have completed the ICMJE uniform disclosure form (available at http://dx.doi.org/10.21037/jss-20-588). BSB serves as an unpaid editorial board member of Journal of Spine Surgery from March 2020 to February 2022. Dr. BJM and Dr. BSB are officers and employees of SINTX Technologies, Inc. Dr. GM is an independent consultant who was contracted by SINTX Technologies to provide biomedical statistical analyses for this study.
Ethical Statement: The authors are accountable for all aspects of the work in ensuring that questions related to the accuracy or integrity of any part of the work are appropriately investigated and resolved. The study was conducted in accordance with the Declaration of Helsinki (as revised in 2013). The study was approved by Medical Research Ethics Committee United, Nieuwegein, the Netherlands (Verenigde Commissies Mensgebonden Onderzoek) (https://www.ccmo.nl/) and informed consent was taken from all the patients.
Open Access Statement: This is an Open Access article distributed in accordance with the Creative Commons Attribution-NonCommercial-NoDerivs 4.0 International License (CC BY-NC-ND 4.0), which permits the non-commercial replication and distribution of the article with the strict proviso that no changes or edits are made and the original work is properly cited (including links to both the formal publication through the relevant DOI and the license). See: https://creativecommons.org/licenses/by-nc-nd/4.0/.
References
- Fritzell P, Hägg O, Wessberg P, et al. 2001 Volvo Award Winner in Clinical Studies: Lumbar fusion versus nonsurgical treatment for chronic low back pain: a multicenter randomized controlled trial from the Swedish Lumbar Spine Study Group. Spine (Phila Pa 1976) 2001;26:2521-32; discussion 2532-4. [Crossref] [PubMed]
- Gore M, Sadosky A, Stacey BR, et al. The Burden of Chronic Low Back Pain. Spine (Phila Pa 1976) 2012;37:E668-77. [Crossref] [PubMed]
- Montgomery W, Sato M, Nagasaka Y, et al. The economic and humanistic costs of chronic lower back pain in Japan. Clinicoecon Outcomes Res 2017;9:361-71. [Crossref] [PubMed]
- Geurts JW, Willems PC, Kallewaard JW, et al. The Impact of Chronic Discogenic Low Back Pain: Costs and Patients' Burden. Pain Res Manag 2018;2018:4696180. [Crossref] [PubMed]
- Mannion AF, Brox JI, Fairbank JC. Consensus at Last! Long-Term Results of all Randomized Controlled Trials Show that Fusion is No Better than Non-Operative Care in Improving Pain and Disability in Chronic Low Back Pain. Spine J 2016;16:588-90. [Crossref] [PubMed]
- Wang JC, Mummaneni PV, Haid RW. Current Treatment Strategies for the Painful Lumbar Motion Segment: Posterolateral Fusion versus Interbody Fusion. Spine (Phila Pa 1976) 2005;30:S33-43. [Crossref] [PubMed]
- Willems P, de Bie R, Oner C, et al. Clinical Decision Making in Spinal Fusion for Chronic Low Back Pain. Results of a Nationwide Survey Among Spine Surgeons. BMJ Open 2011;1:e000391. [Crossref] [PubMed]
- Zhou ZJ, Zhao FD, Fang XQ, et al. Meta-Analysis of Instrumented Posterior Interbody Fusion versus Instrumented Posterolateral Fusion in the Lumbar Spine. J Neurosurg Spine 2011;15:295-310. [Crossref] [PubMed]
- Yavin D, Casha S, Wiebe S, et al. Lumbar Fusion for Degenerative Disease: A Systematic Review and Meta-Analysis. Neurosurgery 2017;80:701-15. [Crossref] [PubMed]
- Lee YC, Zotti MGT, Osti OL. Operative Management of Lumbar Degenerative Disc Disease. Asian Spine J 2016;10:801-19. [Crossref] [PubMed]
- Brantigan JW. Pseudarthrosis Rate after Allograft Posterior Lumbar Interbody Fusion with Pedicle Screw and Plate Fixation. Spine (Phila Pa 1976) 1994;19:1271-9. [Crossref] [PubMed]
- McAfee PC. Interbody fusion cages in reconstructive operations on the spine. J Bone Joint Surg Am 1999;81:859-80. [Crossref] [PubMed]
- Rao PJ, Pelletier MH, Walsh WR, et al. Spine Interbody Implants: Material Selection and Modification, Functionalization and Bioactivation of Surfaces to Improve Osseointegration. Orthop Surg 2014;6:81-9. [Crossref] [PubMed]
- Phan K, Mobbs RJ. Evolution of Design of Interbody Cages for Anterior Lumbar Interbody Fusion. Orthop Surg 2016;8:270-7. [Crossref] [PubMed]
- Katchko K, Schneider AD, Hsu WK. Lumbar Interbody Fusion Implant Materials. Contemporary Spine Surgery 2017;18:1-8. [Crossref]
- Kurtz SM. editor. Development and Clinical Performance of PEEK Intervertebral Cages. In: PEEK Biomaterials Handbook. 2nd edition. Elsevier Inc., 2019:263-80.
- Kuslich SD, Ulstrom CL, Griffith SL, et al. The Bagby and Kuslich method of lumbar interbody fusion. History, techniques, and 2-year follow-up results of a United States prospective, multicenter trial. Spine (Phila Pa 1976) 1998;23:1267-78; discussion 1279. [Crossref] [PubMed]
- Feller L, Jadwat Y, Khammissa RA, et al. Cellular responses evoked by different surface characteristics of intraosseous titanium implants. Biomed Res Int 2015;2015:171945. [Crossref] [PubMed]
- McEntire BJ, Bal BS, Rahaman MN, et al. Ceramics and Ceramic Coatings in Orthopaedics. J Eur Ceram Soc 2015;35:4327-69. [Crossref]
- Rousseau MA, Lazennec JY, Saillant G. Circumferential Arthrodesis using PEEK Cages at the Lumbar Spine. J Spinal Disord Tech 2007;20:278-81. [Crossref] [PubMed]
- Cutler AR, Siddiqui S, Mohan AL, et al. Comparison of Polyetheretherketone Cages with Femoral Cortical Bone Allograft as a Single-Piece Interbody Spacer in Transforaminal Lumbar Interbody Fusion. J Neurosurg Spine 2006;5:534-9. [Crossref] [PubMed]
- Noiset O, Schneider YJ, Marchand-Brynaert J. Fibronectin Adsorption or/and Covalent Grafting on Chemically Modified PEEK Film Surfaces. J Biomater Sci Polym Ed 1999;10:657-77. [Crossref] [PubMed]
- Gorth DJ, Puckett S, Ercan B, et al. Decreased Bacteria Activity on Si3N4 Surfaces Compared with PEEK or Titanium. Int J Nanomedicine 2012;7:4829-40. [PubMed]
- Webster TJ, Patel AA, Rahaman MN, et al. Anti-Infective and Osteointegration Properties of Silicon Nitride, Poly (Ether Ether Ketone), and Titanium Implants. Acta Biomater 2012;8:4447-54. [Crossref] [PubMed]
- Khonsari RH, Berthier P, Rouillon T, et al. Severe Infectious Complications after PEEK-Derived Implant Placement: Report of Three Cases. J Oral Maxillofac Surg Med Pathol 2014;26:477-82. [Crossref]
- Phan K, Hogan JA, Assem Y, et al. PEEK-Halo effect in interbody fusion. J Clin Neurosci 2016;24:138-40. [Crossref] [PubMed]
- Kersten RFMR, Wu G, Pouran B, et al. Comparison of polyetheretherketone versus silicon nitride intervertebral spinal spacers in a caprine model. J Biomed Mater Res B Appl Biomater 2019;107:688-99. [Crossref] [PubMed]
- Seaman S, Kerezoudis P, Bydon M, et al. Titanium vs. Polyetheretherketone (PEEK) Interbody Fusion: Meta-Analysis and Review of the Literature. J Clin Neurosci 2017;44:23-9. [Crossref] [PubMed]
- Kersten RF, van Gaalen SM, de Gast A, et al. Polyetheretherketone (PEEK) cages in cervical applications: a systematic review. Spine J 2015;15:1446-60. [Crossref] [PubMed]
- Marchi L, Abdala N, Oliveira L, et al. Radiographic and Clinical Evaluation of Cage Subsidence after Stand-Alone Lateral Interbody Fusion. J Neurosurg Spine 2013;19:110-8. [Crossref] [PubMed]
- Suh PB, Puttlitz C, Lewis C, et al. The Effect of Cervical Interbody Cage Morphology, Material Composition, and Substrate Density on Cage Subsidence. J Am Acad Orthop Surg 2017;25:160-8. [Crossref] [PubMed]
- Chong E, Mobbs RJ, Pelletier MH, et al. Titanium/Polyetheretherketone Cages for Cervical Arthrodesis with Degenerative and Traumatic Pathologies: Early Clinical Outcomes and Fusion Rates. Orthop Surg 2016;8:19-26. [Crossref] [PubMed]
- Torstrick FB, Lin ASP, Potter D, et al. Porous PEEK Improves the Bone-Implant Interface Compared to Plasma-Sprayed Titanium Coating on PEEK. Biomaterials 2018;185:106-16. [Crossref] [PubMed]
- Barkarmo S, Andersson M, Currie F, et al. Enhanced Bone Healing Around Nanohydroxyapatite-Coated Polyetheretherketone Implants: An Experimental Study in Rabbit Bone. J Biomater Appl 2014;29:737-47. [Crossref] [PubMed]
- Johansson P, Barkarmo S, Hawthan M, et al. Biomechanical, Histological, and Computed X-ray Tomographic Analyses of Hydroxyapatite-Coated PEEK Implants in an Extended Healing Model in Rabbit. J Biomed Mater Res A 2018;106:1440-7. [Crossref] [PubMed]
- Kersten RFMR, van Gaalen SM, Willems PC, et al. Lumbar Spinal Fusion: Indications, Surgical Techniques and Post-Operative Management. A Survey among Spine Surgeons in the Netherlands. MOJ Orthop Rheumatol 2016;4:00155. [Crossref]
- Kersten RF, van Gaalen SM, Arts MP, et al. The SNAP Trial: A Double Blind Multi-Center Randomized Controlled Trial of a Silicon Nitride Versus a PEEK Cage in Transforaminal Lumbar Interbody Fusion in Patients with Symptomatic Degenerative Lumbar Disc Disorders: Study Protocol. BMC Musculoskelet Disord 2014;15:57. [Crossref] [PubMed]
- McEntire BJ, Lakshminarayanan R, Thirugnanasambandam P, et al. Processing and Characterization of Silicon Nitride Bioceramics. Bioceram Dev Appl 2016;6:1000093. [Crossref]
- McEntire BJ, Enomoto Y, Zhu W, et al. Surface Toughness of Silicon Nitride Bioceramics: II, Comparison with Commercial Oxide Materials. J Mech Behav Biomed Mater 2016;54:346-59. [Crossref] [PubMed]
- Anderson M, Bernero J, Brodke D. Medical Imaging Characteristics of Silicon Nitride Ceramic A New Material for Spinal Arthroplasty Implants. In: 8th Annual Spine Arthroplasty Society Global Symposium on Motion Preservation Technology, Miami, FL; 2008:547.
- Bock RM, Jones EN, Ray DA, et al. Bacteriostatic Behavior of Surface-Modulated Silicon Nitride in Comparison to Polyetheretherketone and Titanium. J Biomed Mater Res A 2017;105:1521-34. [Crossref] [PubMed]
- Howlett CR, McCartney E, Ching W. The Effect of Silicon Nitride Ceramic on Rabbit Skeletal Cells and Tissue. Clin Orthop Relat Res 1989.293-304. [PubMed]
- Neumann A, Reske T, Held M, et al. Comparative Investigation of the Biocompatibility of Various Silicon Nitride Ceramic Qualities in vitro. J Mater Sci Mater Med 2004;15:1135-40. [Crossref] [PubMed]
- Mazzocchi M, Gardini D, Traverso PL, et al. On the Possibility of Silicon Nitride as a Ceramic for Structural Orthopaedic Implants. Part II: Chemical Stability and Wear Resistance in Body Environment. J Mater Sci Mater Med 2008;19:2889-901. [Crossref] [PubMed]
- Pezzotti G, Enomoto Y, Zhu W, et al. Surface Toughness of Silicon Nitride Bioceramics: I, Raman Spectroscopy-Assisted Micromechanics. J Mech Behav Biomed Mater 2016;54:328-45. [Crossref] [PubMed]
- Pezzotti G, McEntire BJ, Bock R, et al. Silicon Nitride: A Synthetic Mineral for Vertebrate Biology. Sci Rep 2016;6:31717. [Crossref] [PubMed]
- Sorrell CC, Hardcastle PH, Druitt RK, et al. Results of 15-Year Clinical Study of Reaction Bonded Silicon Nitride Intervertebral Spacers. Proceedings of the 7th World Biomaterials Congress, 2004;1872.
- Mobbs RJ, Rao PJ, Phan K, et al. Anterior Lumbar Interbody Fusion Using Reaction Bonded Silicon Nitride Implants: Long Term Case Series of the First Synthetic ALIF Spacer Implanted in Humans. World Neurosurg 2018;120:256-64. [Crossref] [PubMed]
- Arts MP, Wolfs JFC, Corbin TP. Porous Silicon Nitride Spacers versus PEEK Cages for Anterior Cervical Discectomy and Fusion: Clinical and Radiological Results of a Single-Blinded Randomized Controlled Trial. Eur Spine J 2017;26:2372-9. [Crossref] [PubMed]
- Ball HT, McEntire BJ, Bal BS. Accelerated Cervical Fusion of Silicon Nitride versus PEEK Spacers: A Comparative Clinical Study. J Spine 2017;6:1000396. [Crossref]
- Smith MW, Romano DR, McEntire BJ, et al. A Single Center Retrospective Clinical Evaluation of Anterior Cervical Discectomy and Fusion Comparing Allograft Spacers to Silicon Nitride Cages. J Spine Surg 2018;4:349-60. [Crossref] [PubMed]
- Youssef JA, Myhre SL, Bal BS. Radiographic Follow-up of Transforaminal Lumbar Fusion with Silicon Nitride Spacers: A Case Report of Two Patients. J Musculoskelet Disord Treat 2016;2:009.
- Rambo WM. Treatment of Lumbar Discitis using Silicon Nitride Spinal Spacers: A Case Series and Literature Review. Int J Surg Case Rep 2018;43:61-8. [Crossref] [PubMed]
- Schulz KF, Altman DG, Moher D. CONSORT 2010 Statement: Updated Guidelines for Reporting Parallel Group Randomised Trials. Int J Surg 2011;9:672-7. [Crossref] [PubMed]
- Piaggio G, Elbourne DR, Pocock SJ, et al. Reporting of Noninferiority and Equivalence Randomized Trials: Extension of the CONSORT 2010 Statement. JAMA 2012;308:2594-604. [Crossref] [PubMed]
- Brouwer S, Kuijer W, Dijkstra PU, et al. Reliability and Stability of the Roland Morris Disability Questionnaire: Intra Class Correlation and Limits of Agreement. Disabil Rehabil 2004;26:162-5. [Crossref] [PubMed]
- Ware JE, Sherbourne CD. The MOS 36-item short-form health survey (SF-36). I. Conceptual framework and item selection. Med Care 1992;30:473-83. [Crossref] [PubMed]
- van Hooff ML, Spruit M, Fairbank JCT, et al. The Oswestry Disability Index (Version 2.1a). Spine (Phila Pa 1976) 2015;40:E83-90. [Crossref] [PubMed]
- Collins SL, Moore RA, McQuay HJ. The Visual Analogue Pain Intensity Scale: What is Moderate Pain in millimetres? Pain 1997;72:95-7. [Crossref] [PubMed]
- Likert R. A Technique for the Measurement of Attitudes. Archives of Psychology 1932;22:55.
- Bombardier C. Outcome Assessments in the Evaluation of Treatment of Spinal Disorders: Summary and General Recommendations. Spine (Phila Pa 1976) 2000;25:3100-3. [Crossref] [PubMed]
- Burkus JK, Foley KT, Haid RW, et al. Surgical Interbody Research Group - Radiographic Assessment of Interbody Fusion Devices: Fusion Criteria for Anterior Lumbar Interbody Surgery. Neurosurg Focus 2001;10:E11. [Crossref] [PubMed]
- Park Y, Ha JW, Lee YT, et al. The Effect of a Radiographic Solid Fusion on Clinical Outcomes after Minimally Invasive Transforaminal Lumbar Interbody Fusion. Spine J 2011;11:205-12. [Crossref] [PubMed]
- Ostelo RWJG, De Vet HCW, Knol DL, et al. 24-Item Roland-Morris Disability Questionnaire was Preferred Out of Six Functional Status Questionnaires for Post-Lumbar Disc Surgery. J Clin Epidemiol 2004;57:268-76. [Crossref] [PubMed]
- Bartlett MS. Properties of Sufficiency and Statistical Tests. Proc R Soc London Math Phys Sci 1937;160:268-82.
- Robertson PA, Plank LD. Prospective Cohort Analysis of Disability Reduction with Lumbar Spinal Fusion Surgery in Community Practice. J Spinal Disord Tech 2008;21:235-40. [Crossref] [PubMed]
- Scheufler KM, Dohmen H, Vougioukas VI. Percutaneous Transforaminal Lumbar Interbody Fusion for the Treatment of Degenerative Lumbar Instability. Neurosurgery 2007;60:203-12; discussion 212-3. [PubMed]
- Patrick DL, Deyo RA, Atlas SJ, et al. Assessing health-related quality of life in patients with sciatica. Spine (Phila Pa 1976) 1995;20:1899-908; discussion 1909.
- Roland M, Fairbank J. The Roland Morris Disability Questionnaire and the Oswestry Disability Questionnaire. Spine (Phila Pa 1976) 2000;25:3115-24. [Crossref] [PubMed]
- Stratford PW, Binkley JM, Riddle DL, et al. Sensitivity to Change of the Roland-Morris Back Pain Questionnaire: Part 1. Phys Ther 1998;78:1186-96. [Crossref] [PubMed]
- Riddle DL, Stratford PW, Binkley JM. Sensitivity to Change of the Roland-Morris Back Pain Questionnaire: Part 2. Phys Ther 1998;78:1197-207. [Crossref] [PubMed]
- Brouwer PA, Brand R, van den Akker-van Marle ME, et al. Percutaneous Laser Disc Decompression Versus Conventional Microdiscectomy in Sciatica: A Randomized Controlled Trial. Spine J 2015;15:857-65. [Crossref] [PubMed]
- Ohba T, Ebata S, Haro H. Comparison of serum markers for muscle damage, surgical blood loss, postoperative recovery, and surgical site pain after extreme lateral interbody fusion with percutaneous pedicle screws or traditional open posterior lumbar interbody fusion. BMC Musculoskelet Disord 2017;18:415. [Crossref] [PubMed]
- Stratford PW, Binkley J, Solomon P, et al. Defining the Minimum Level of Detectable Change for the Roland Morris Questionnaire. Phys Ther 1996;76:359-65. [Crossref] [PubMed]
- US FDA. Guidance Document for the Preparation of IDEs for Spinal Systems. Device Evaluation Office United States, 2000:1-32.
- Jiya TU, Smit T, Van Royen BJ, et al. Posterior Lumbar Interbody Fusion using Non Resorbable Poly-ether-ether- ketone Versus Resorbable Poly-L-lactide-co-D, L-Lactide Fusion Devices. Clinical Outcome at a Minimum of 2-Year Follow-up. Eur Spine J 2011;20:618-22. [Crossref] [PubMed]
- Nemoto O, Asazuma T, Yato Y, et al. Comparison of Fusion Rates following Transforaminal Lumbar Interbody Fusion using Polyetheretherketone Cages or Titanium Cages with Transpedicular Instrumentation. Eur Spine J 2014;23:2150-5. [Crossref] [PubMed]
- Yu CH, Wang CT, Chen PQ. Instrumented Posterior Lumbar Interbody Fusion in Adult Spondylolisthesis. Clin Orthop Relat Res 2008;466:3034-43. [Crossref] [PubMed]
- Bevevino AJ, Kang DG, Lehman RA, et al. Systematic Review and Meta-Analysis of Minimally Invasive Transforaminal Lumbar Interbody Fusion Rates Performed Without Posterolateral Fusion. J Clin Neurosci 2014;21:1686-90. [Crossref] [PubMed]
- Fogel GR, Toohey JS, Neidre A, et al. Fusion assessment of posterior lumbar interbody fusion using radiolucent cages: X-ray films and helical computed tomography scans compared with surgical exploration of fusion. Spine J 2008;8:570-7. [Crossref] [PubMed]
- Goldstein C, Drew B. When is a spine fused? Injury 2011;42:306-13. [Crossref] [PubMed]
- Kroeze RJ, Van Der Veen AJ, Van Royen BJ, et al. Relation Between Radiological Assessment and Biomechanical Stability of Lumbar Interbody Fusion in a Large Animal Model. Eur Spine J 2013;22:2731-9. [Crossref] [PubMed]
- Djurasovic M, Glassman SD, Dimar JR, et al. Does Fusion Status Correlate with Patient Outcomes in Lumbar Spinal Fusion? Spine (Phila Pa 1976) 2011;36:404-9. [Crossref] [PubMed]
- Keorochana G, Setrkraising K, Woratanarat P, et al. Clinical Outcomes after Minimally Invasive Transforaminal Lumbar Interbody Fusion and Lateral Lumbar Interbody Fusion for Treatment of Degenerative Lumbar Disease: A Systematic Review and Meta-Analysis. Neurosurg Rev 2018;41:755-70. [Crossref] [PubMed]
- Vazan M, Gempt J, Meyer B, et al. Minimally Invasive Transforaminal Lumbar Interbody Fusion Versus Open Transforaminal Lumbar Interbody Fusion: A Technical Description and Review of the Literature. Acta Neurochir (Wien) 2017;159:1137-46. [Crossref] [PubMed]
- Joseph JR, Smith BW, La Marca F, et al. Comparison of Complication Rates of Minimally Invasive Transforaminal Lumbar Interbody Fusion and Lateral Lumbar Interbody Fusion: A Systematic Review of the Literature. Neurosurg Focus 2015;39:E4. [Crossref] [PubMed]
- Landham PR, Don AS, Robertson PA. Do Position and Size Matter? An Analysis of Cage and Placement Variables for Optimum Lordosis in PLIF Reconstruction. Eur Spine J 2017;26:2843-50. [Crossref] [PubMed]