Kyphoplasty versus percutaneous posterior instrumentation for osteoporotic vertebral fractures with posterior wall injury: a propensity score matched cohort study
Introduction
Vertebral compression fractures represent the most common type of fracture resulting from osteoporosis, affecting 30–50% of people over the age of 50 (1). Patients with osteoporotic vertebral fractures (OVFs) face significant impairment to quality of life and increased overall mortality risk for up to 5 years after initial fracture diagnosis (2-4). Balloon kyphoplasty (BKP) is considered a standard surgical procedure for the treatment of painful OVFs (5-7). Randomized controlled trials (RCTs) and prospective open-label studies have demonstrated the safety and superiority of BKP compared with non-surgical management in improving pain, function, quality of life, and patient satisfaction (8-11). Immediate reduction in pain, promoting early mobilization, and improvement of vertebral body deformity and angular kyphosis are the goals of surgery. However, a more severe subtype of OVF shows cortical defects of the posterior vertebral wall (12), potentially resulting in a segmental instability. In these cases, a reasonable apprehension exists among surgeons regarding the risk of posterior cement leakage into the spinal canal during augmentation and subsequent neurologic deficits (13-15). Although percutaneous fixation with pedicle screws (PS) might be performed under such circumstances, concerns exist regarding implant-related complications (i.e., screw pull-out, subsidence) because of poor bone quality (16). Furthermore, posterior instrumentation, which must include several vertebral levels for adequate fixation (17), requires longer constructs in osteoporotic patients that presumably results in longer surgical times and a higher probability of perioperative complications given their advanced age and morbidity. Standalone BKP still can be an option for the treatment of OVFs even in the presence of posterior wall injury given that there are relative but no absolute contraindications to the procedure (18). However, no controlled clinical trials have been conducted for the surgical management of OVFs with posterior wall injury, and treatment recommendations for OVFs are often based on expert consensus by different medical societies (19,20).
With the growing healthcare problem of osteoporosis globally and the lack of comparative data on perioperative morbidity of different surgical techniques, this retrospective study evaluated our center’s procedural data, surgical results, and perioperative complications in a cohort of patients with OVFs with posterior wall injury who underwent treatment with BKP with or without percutaneous posterior instrumentation. Compared with percutaneous posterior instrumentation, we hypothesized that standalone BKP could be safely performed to achieve both sufficient correction of radiographic parameters and good clinical outcomes. We present the following article in accordance with the STROBE reporting checklist (21) (available at http://dx.doi.org/10.21037/jss-20-625).
Methods
This retrospective study included 50 patients with 61 OVFs of the thoracic or lumbar vertebrae with evidence of posterior wall cortical injury who underwent surgical treatment between January 2010 and December 2018 at a single tertiary care center. Surgical treatment included BKP or percutaneous posterior instrumentation with PS or both. The study was conducted in accordance with the Declaration of Helsinki (as revised in 2013). The study was approved by the Ethical Committee of Northwestern and Central Switzerland (ID number EKNZ BASEC 2018-00185), and individual consent for this retrospective analysis was waived.
Inclusion criteria
Inclusion criteria were the following:
- Age ≥50 years;
- OVFs occurred spontaneously or as a result of low-energy trauma;
- One to three OVFs of the thoracic and/or lumbar vertebrae with cortical disruption and morphological alterations of the posterior wall in at least one vertebra identified on preoperative sagittal computed tomography (CT) imaging;
- Fracture age <8 weeks;
- Evidence of vertebral body edema on magnetic resonance imaging (MRI) with fat suppression sequences suggestive of acute or subacute fractures in two- or three-level fractures.
Exclusion criteria
Exclusion criteria were the following:
- Posterior tension band injuries according to AOSpine thoracolumbar injury classification system (22);
- High-energy trauma identified as the mechanism of injury;
- Simple bulging of the posterior wall with no obvious cortical disruption on sagittal CT imaging;
- Symptomatic spinal canal stenosis requiring decompression;
- Metastasizing malignancy, myeloproliferative disorder or multiple myeloma;
- Ankylosing spondylitis or diffuse idiopathic skeletal hyperostosis;
- Parkinson’s disease;
- Degenerative scoliosis with a Cobb angle >20°;
- Prior arthrodesis or cement augmentation of adjacent segments.
Surgical technique
The kit used for percutaneous instrumentation was Viper® 2 (DePuy Synthes Spine, Oberdorf, Switzerland) until 2015, and the CD Horizon® Longitude® II (Medtronic Spinal and Biologics, Memphis, TN, USA) from 2016 to 2018. The kit used for BKP was Confidence Spinal Cement System® (DePuy Synthes Spine, Oberdorf, Switzerland), and Kyphon® Express and Express II (Medtronic Spinal and Biologics, Memphis, TN, USA). Bone cement used for screw augmentation and BKP was Confidence® (DePuy Synthes Spine, Oberdorf, Switzerland), and Kyphon® Xpede (Medtronic Spinal and Biologics, Memphis, TN, USA). Both cements were composed of polymethylmethacrylate (PMMA), mixed during surgery, and set to reach an appropriate viscosity for injection. In a stepwise approach from both sides under fluoroscopy, the cement was injected slowly through the cannulated screws or into the void created by the balloons using the Confidence Spinal Cement System® (DePuy Synthes Spine), the Kyphon® bone-filling devices, or the Kyphon® Cement Delivery System (Medtronic Spinal and Biologics). In the PS group, BKP was performed only after screw placement, insertion and reduction of the rods and final closure of the system to prevent disruption of the bone-cement-interface at the index level.
Group formation
We identified two study groups based on the surgical treatment received. First, the PS group included 25 patients with 32 OVFs with posterior wall injury who underwent percutaneous placement of cannulated and fenestrated PS and rod constructs with additional cement augmentation techniques (Figure 1). Very few patients who underwent percutaneous instrumentation without BKP, but cement augmentation of PS, were also included in this group for the ease of analysis. Second, the BKP group was selected based on comparable propensity scores (23) from our previously reported study of 214 patients who underwent BKP for OVFs at our institution (24). Of these, 46 patients met the inclusion criteria of posterior wall cortical injury detected on CT imaging and were further screened with propensity scoring. With propensity scores matched to each PS patient (1:1) as closely as possible, the BKP group consisted of 25 patients with 29 OVFs with posterior wall injury (Figure 2). Propensity scores were calculated and matched based on the treatment and the covariates of: age, sex, number of fractured vertebrae, fracture etiology (spontaneous or low-energy trauma), fracture age, involvement of the thoracolumbar junction (T11–L2), sagittal Cobb angle (angle between the superior endplate from the vertebral body one level above the injured vertebral body and the inferior endplate of the vertebral body one level below) (25), vertebral wedge angle (angle between superior and inferior endplate of the injured vertebral body), posterior cortical wall height loss >1/5, and additional presence of pincer-type injury (focal cortical injury of the upper and lower endplate without fracture dislocation).
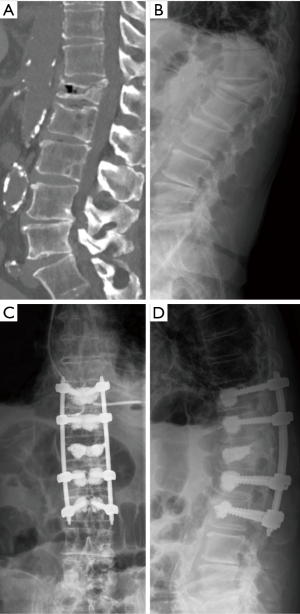

Surgical and clinical decisions were made by attending spine surgeons on a case-by-case series or by team consensus. Procedures were performed under general anesthesia with the patient positioned prone, and with the use of two image intensifiers (anteroposterior and lateral views). Standing radiographs after surgery were obtained as soon as the patients were mobilized, normally on the first postoperative day, and at every follow-up visit. Patient and radiological data were retrieved from the local patient data management software and Picture Archiving and Communication System (PACS). Radiographic reviews were done by a single board-certified neurosurgeon and attending spine surgeon using PACS viewer Merlin 5.3 (Phoenix-PACS, Freiburg, Germany). After completion of data collection, patient data was encrypted before statistical analysis.
Primary endpoint
The primary endpoint, the occurrence of a postoperative complication, was defined using the 4-point grading system proposed by Landriel Ibañez et al. (26). This scale defines the severity of a complication as any deviation from the normal postoperative course that develops within 30 days of surgery. Grades, from mild to severe, were based on the therapy then administered to treat a postoperative adverse event:
- Grade I (mild): non-life-threating complication not requiring drug treatment (grade Ia), or requiring drug treatment (grade Ib);
- Grade II (moderate): complication requiring invasive treatment (surgical, endoscopic, or endovascular) without general anesthesia (grade IIa), or under general anesthesia (grade IIb);
- Grade III (severe): life-threating complication requiring management in intensive care unit (ICU) for a single organ failure (grade IIIa), or multiple organ failure (grade IIIb);
- Grade IV (death).
In patients suffering more than one complication within the first 30 days postoperatively, the complication with the highest grade was considered for analysis.
Secondary endpoints
Secondary endpoints were improvements of sagittal radiographic parameters, including Cobb angle for sagittal deformity, vertebral wedge angle, and anterior vertebral height. In multilevel OVFs, radiographic measurements were confined only to the vertebrae with posterior wall injury. Fractures occurring exclusively at the L3–L5 vertebrae were excluded from analysis because of the lordotic orientation of the lower lumbar spine. Radiographic parameters were evaluated by radiographic analysis to compare baseline preoperative images with the first postoperative standing lateral radiographs. Additionally, presence, direction and extent of cement leakage were evaluated on postoperative anteroposterior and lateral radiographs. Potential loss of correction was evaluated by comparing standing lateral radiographs at 3-month follow-up with early postoperative images.
Surgical time and length of stay (LOS) were also assessed. Patient-reported outcomes included initial back pain and at 3-month follow-up rated on a numeric rating scale (NRS) from 0 (no pain) to 10 (worst pain imaginable), and opioid use at discharge, and 3-month follow-up. Minimally clinically significant improvement in back pain was considered an NRS change of 3.5 points (27). Occurrence of any subsequent OVFs was noted in up to 3-month follow-up.
Statistical analysis
Pearson’s χ2 test and Fisher’s Exact test were used for the comparison of categorical data. The two-sample t test and Mann-Whitney U test were used for the comparison of means, with the calculation of 95% confidence intervals (CIs), as applicable. Descriptive statistics were used to calculate means, medians, standard deviations, and ranges. Subgroup analysis was performed, if appropriate. Statistical analysis was performed with SPSS Statistics version 23.0 (IBM Corp., Armonk NY, USA). Results at a probability value P<0.05 were considered statistically significant.
Results
Demographic and surgical characteristics
Demographics and baseline characteristics of the study population are reported in Table 1. Both groups were comparable for the 10 preoperative covariates rated in propensity score matching, except for the smaller sagittal Cobb angle in the BKP versus PS groups [mean ± standard deviation (SD)] of 15.8°±8.7° vs. 22.9°±9°, respectively (95% CI: 1.78–12.386; P=0.010). Of covariates excluded from propensity score matching, differences between BKP and PS groups, respectively, were significant for body mass index (BMI) (24.3±4.5 vs. 27.5±5 kg/m2; 95% CI: 0.4934–5.8826; P=0.021); first manifestation of osteoporosis (80% vs. 48%; P=0.018); and American Society of Anesthesiologists (ASA) Class II (64% vs. 32%; P=0.024). Surgical characteristics are outlined in Table 2. In the PS group, 200 PS were inserted in 105 vertebrae, with instrumentation two levels above and two levels below the index level in 12 (63.2%) of 19 single-level fractures (Figure 1). In this group, cement augmentation with PMMA was used for the screws in 21 (84%) of 25 patients, and all but 3 patients had additional BKP of the fracture level, preferably as a single-staged procedure. Follow-up averaged 8.4 months (range, 1–56.4 months).
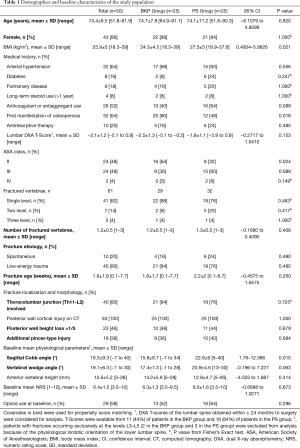
Full table
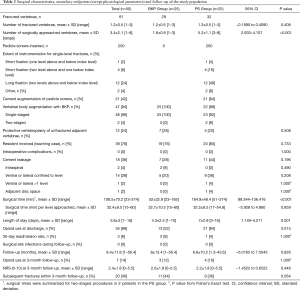
Full table
30-day morbidity
Overall, 17 (34%) of all 50 patients developed a complication within 30 days after surgery, 6 (24%) in the BKP group and 11 (44%) in the PS group (P=0.136) (Table 3). Of complications recorded, 14 (82.4%) were mild, 2 (11.8%) were moderate, and 1 (5.9%) was severe; differences between groups were not significant, but a trend towards more mild complications requiring drug treatment (Ibañez Ib) was observed in the PS group (P=0.059). The 30-day mortality was 0%, and no surgical site infections were observed during follow-up. Mild complications commonly included water-electrolyte imbalance, urinary tract infection, delirium, and pulmonary embolism; all were treated with medications (Ibañez Ib). Of note, a 76-year-old woman in the PS group with cement augmentation of PS (1/21; 4.8%) developed symptomatic pulmonary cement embolism that was confirmed by contrast-enhanced chest CT; she was readmitted 4 days after discharge (7 days after surgery) because of progressive dyspnea. After treatment with Rivaroxaban and medications for additional co-morbidities, dyspnea resolved and the patient recovered without further interventions (Ibañez Ib). One 81-year-old woman in the PS group developed transient proximal muscle weakness in her right leg that resolved within a few days after treatment with dexamethasone; this was not attributed to screw misplacement or cement leakage (Ibañez Ib), but was possibly related to a subsequent fracture of the lower endplate of the lower instrumented vertebra L4. No other neurologic complications developed in the study population. Except for placement of a suprapubic catheter under local anesthesia (Ibañez IIa) in one patient in each group, there were no endoscopic or surgical interventions performed within the first 30 days postoperatively.
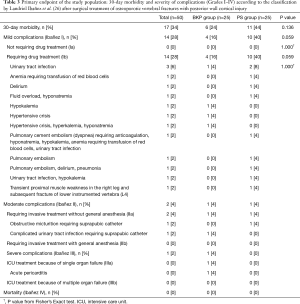
Full table
Secondary endpoints
Secondary endpoints are outlined in Table 2, with radiographic parameters before and after surgery shown in Figures 3-5. No significant differences in cement leakage were observed between groups; specifically, 7 (28%) in the BKP group and 11 (44%) in the PS group (P=0.196). Most cement leaks occurred ventrally and/or laterally confined to the vertebral level of origin. Asymptomatic cement leakage into the spinal canal contained by the posterior longitudinal ligament occurred in two patients only in the BKP group (P=0.490).
All radiographic parameters significantly improved compared with baseline measures. Mean preoperative sagittal Cobb angle improved from 15.8°±8.7° (median 16°) at baseline to 11.1°±9.4° (median 8°) after surgery (95% CI: 1.761–7.63; P=0.003) in the BKP group and from 22.9°±9° (median 23°) at baseline to 8.6°±8.4° (median 10°) after surgery (95% CI: 11.809–16.828; P<0.001) in the PS group (Figure 3). Mean preoperative vertebral wedge angle improved from 17.4°±7.3° (median 18°) at baseline to 9.1°±6.7° (median 8°) after surgery (95% CI: 5.352–11.083; P<0.001) in the BKP group and from 20.9°±4.6° (median 21°) to 5.5°±5.6° (median 3°) after surgery (95% CI: 12.381–18.437; P<0.001) in the PS group (Figure 4). Mean anterior vertebral height improved from 14.2±5.9 mm (median 12 mm) at baseline to 21.5±5.7 mm (median 22 mm) after surgery (95% CI: −9.026 to −5.582; P<0.001) in the BKP group and from 12.9±4.7 mm (median 13 mm) to 24.3±4.2 mm (median 25 mm) after surgery (95% CI: −13.227 to −9.5; P<0.001) in the PS group (Figure 5).
At 3-month follow-up, mean sagittal Cobb angle increased to 14.9°±7.4° in the BKP group (average loss of correction 3.8°) and remained stable at 8.2°±9.2° in the PS group. Mean vertebral wedge angle increased to 11.9°±6.4° in the BKP group (average loss of correction 2.8°) and to 7.1°±4.3° in the PS group (average loss of correction 1.6°). Mean anterior vertebral height decreased to 19.3±5.1 mm in the BKP group (average loss of correction 2.2 mm) and to 22±5 mm in the PS group (average loss of correction 2.3 mm). Although no statistical significant differences were seen in radiographic parameters early after surgery between groups (all P>0.05), mean sagittal Cobb angle and mean vertebral wedge angle were significantly greater at 3-month follow-up in the BKP than the PS group, with 14.9°±7.4° vs. 8.2°±9.2° (95% CI: −12.227 to −1.212; P=0.018) and 11.9°±6.4° vs. 7.1°±4.3° (95% CI: −8.463 to −1.326; P=0.009), respectively.
Surgical times were significantly shorter in the BKP than PS groups at 52±32.9 vs. 164.9±48.4 min, respectively (95% CI: 89.344–136.416; P<0.001). Significantly fewer vertebrae per patient were surgically approached in the BKP (1.6±0.9) than PS (5.2±1.1) groups (95% CI: 2.933–4.107; P<0.001). Comparing BKP and PS groups, respectively, mean LOS was significantly shorter at 4.3±2.5 vs. 7±2.9 days (95% CI: 1.149–4.211; P=0.001), and opioid use at discharge was significantly less at 52% vs. 84% (P=0.015).
At 3-month follow-up, both groups had achieved the minimally clinical important change in NRS score for back pain: improvement averaged 3.7±1.4 in the BKP group versus 4.2±1.5 in the PS group (95% CI: −0.3125 to 1.3925; P=0.209). No differences were observed in final NRS scores between groups (2.6±1.9 vs. 2.2±1.8; 95% CI: −1.4522 to 0.6522; P=0.448). Opioid use at 3-month follow-up was equally low in both groups, with no significant difference (12% BKP vs. 16% PS groups; P=1.000).
Subsequent fractures occurring within 3-month follow-up affected 20 (40%) of all patients, 11 (44%) in the BKP group and 9 (36%) in the PS group (P=0.564). Fractures were diagnosed an average of 45.7±9.1 in BKP and 47±18.6 days in PS groups (95% CI: −12.060 to 14.605; P=0.843) after the index procedure. Four patients in the BKP group showed fracture progression of the cement augmented level, which was managed conservatively in all cases. Of two patients in the PS group with a subsequent fracture of the lower instrumented vertebra, both had received instrumentation two levels above and one level below the index level: one was managed conservatively, and another underwent revision surgery with caudal extension of the instrumentation 83 days after the index procedure. Adjacent level fractures, which accounted for 70% of subsequent fractures, equally affected 7 (28%) patients per group (P=1.000).
Discussion
Our retrospective study of 50 patients with 61 OVFs with posterior wall injury provides evidence for the hypothesis that BKP alone was comparable to BKP with percutaneous instrumentation in achieving correction of radiographic parameters and yielding good clinical outcomes. Specifically, both BKP and PS groups showed significant improvement in radiographic parameters versus baseline values and similar 30-day morbidity rates. However, the BKP group achieved significantly shorter mean surgical times, shorter LOS, and received less opioid medication at discharge. Potentially life-threatening complications or directly procedure-related complications were minimal, with one patient affected in each group. Significantly more BKP patients were classified as ASA Class II, whereas more (not significant) PS patients were classified as ASA Class III. At 3-month follow-up, loss of correction was more likely to occur in the BKP group, but this did not affect clinical outcome. Findings of this propensity score matched study can add to the discussion when weighing the choice of treatment in aging populations affected by osteoporosis and increasing numbers of OVFs with a higher risk for instabilities.
Complications, cement leakage, and subsequent fractures
In a retrospective, non-matched study of 99 patients that compared PS with instrumentation to BKP for OVFs with posterior wall injury, Tassemeier et al. (28) showed that the surgical approach and not the ASA Class was a relevant factor for complications and revision surgery. Similar to our results, the authors found patients with PS averaged longer surgical time (120 min), longer LOS (21 days), and better correction of vertebral wedge angle (7.5°). In the EVOLVE-Trial of 350 patients, Beall et al. (11) reported 30-day morbidity after BKP was 24.9%, which aligned with the 24% rate in our BKP group.
Symptomatic pulmonary cement embolism was the only directly procedure-related complication in 1 (2%) patient in our study population. Cement leakage, a common complication of BKP, has a reported incidence ranging from 2.9% to 27% (8,11,29,30). Compared with vertebroplasty, BKP significantly decreases the risk of cement leakage (RR 0.62; 95% CI: 0.47–0.80) (31) because of the lower pressures used to inject the cement into the vertebral body after creating a void with inflatable balloons (5,6). Furthermore, correction of kyphotic deformity and restoration of vertebral height was significantly greater after BKP (31). Cement viscosity, injected cement volume, cortical disruption, and history of pulmonary diseases are factors reported to affect the complications resulting from cement leakage (32).
The risk of cement-related pulmonary embolism after vertebral augmentation techniques for OVFs ranges from 3.5% to 23% (33). In a meta-analysis, Zhan et al. (29) noted that cortical disruption posed a more than 5-fold higher risk of cement leakage after vertebroplasty or BKP (OR, 5.56; 95% CI: 1.84–16.81; P<0.01) than no cortical disruption. The question arises, “Should BKP be performed in the presence of posterior wall injury?” In a retrospective series of 97 patients with incomplete osteoporotic burst fractures, Krüger et al. (34) reported cement leakage in 47.4% of patients, including leakage into the spinal canal in 7.2% of patients; nonetheless, all cases were clinically unremarkable. In a prospective series of 82 patients with 98 OVFs with posterior wall injury treated with BKP, Abdelgawaad et al. (18) found no cement leakage into the spinal canal in any of their patients. The authors attributed this to their technique modification: after first creating a large void in the anterior two-thirds of the vertebrae using small-sized balloons, they incrementally injected high-viscosity cement in volumes equal to or lower than that of the void created. In cases of posterior wall fractures, Liu et al. (35) reported the least cement leakage (3.7%) when using high-viscosity cement and an unilateral approach for BKP, whereas Walter et al. (36) noted higher rates of cement leakage into the spinal canal (9.8%) with 2/13 patients undergoing revision surgery. In our study, the 2 (8%) intraspinal cement leaks in the BKP group oriented longitudinally along the posterior vertebral wall, seen on lateral radiography, indicated an intact posterior longitudinal ligament. To avoid unnecessary radiation exposure, no additional CT imaging was performed because these 2 patients were neurologically intact and had an unremarkable postoperative course. Given the available literature, the rate of symptomatic leaks into the spinal canal seems to be low, and modifications in surgical technique may further reduce that risk.
Instrumentation of the osteoporotic spine is a challenging task because of the tendency to instrumentation failure secondary to pull-out and subsidence, leading to revision surgeries (16). In recent years, although cement augmentation of PS has received growing attention and is increasingly performed (37), there is a lack of Level I evidence in the literature and no standardized technique for cement augmentation of PS. The use of cannulated PS for cement augmentation has been investigated in in-vitro pull-out studies (38,39). In fatigue testing in an osteoporotic cadaveric study, Weiser et al. (17) reported increased stability of long segment fixation (T11–T12 to L2–L3) compared to short fixation (T12 to L2). However, augmentation with bone cement failed to demonstrate a significant increase in the mean cycles to failure and fatigue force between short- and long-segment fixation. Cement augmentation of PS in long fixation together with vertebral augmentation of the fracture level potentially puts the patient at higher risk of symptomatic cement leakage because of the higher total volumes of cement injected. Janssen et al. (40) reported asymptomatic cement leakage in 110 (66.7%) of 165 patients after cement augmentation of PS, while Martín-Fernández et al. (41) reported cement leakage in 650 (62.3%) of 1,043 vertebrae instrumented with cement augmented PS. In the former study, the overall symptomatic complication rate was 5.5% (9/165), including symptomatic pulmonary cement embolism in 3% (5/165), and a 30-day mortality rate as high as 1.8% (3/165). Clinically relevant cement leakage after cement augmentation of PS has been reported in 0.6–5.5% (40-43), which was similar to the 4.8% found in our PS group. Given the high rate of cement leakage reported in the literature, including potentially life-threatening complications and even death, indication to augment PS with bone cement should be cautious and patients should be properly informed about such potential risks.
In a retrospective series of 214 BKPs performed at our institution for OVFs, the 25.7% rate of subsequent fractures in the first year after BKP included 3/4 of those fractures occurring within 12.9 weeks after the index procedure (24). Beall et al. reported 36.7% incidence of subsequent fractures within 3 months after BKP in 350 patients (11). In the present study, the rate of subsequent fractures at 3 months was 44% in the BKP group. Only one patient in our PS group showed a subsequent fracture of the lower instrumented vertebra L4 in the first 30 days after surgery. However, the 30-day morbidity does not adequately reflect subsequent fractures. The lower rate of subsequent fractures in the PS group was probably related to better restoration of radiographic parameters; this did not affect the rate of adjacent level fractures, which were equal between the two study groups.
In the results of an international RCT that included 141 patients, Noriega et al. (44) demonstrated the non-inferiority of a titanium implantable vertebral augmentation device (TIVAD) compared with BKP for painful OVFs. Compared with BKP, TIVAD achieved better fracture reduction of midline vertebral height, better pain control at 1 and 6 months, and lower incidence of adjacent fractures (all significant). Given the technical advancement in this vertebral augmentation technique, the availability of a new classification-system (12), and classification-based scoring methods for OVFs (20), surgical decision making may be refined and candidates for standalone vertebral augmentation recognized more easily.
Among several limitations, first is the selection bias of this retrospective, single-center study with specified inclusion criteria. Nonetheless, using matching with propensity scores, two comparable groups could be formed in terms of age, sex, osteoporosis severity, and fracture characteristics, although kyphotic deformity at baseline, as measured by sagittal Cobb angle, was significantly greater in the PS group. Second, the indication to perform percutaneous instrumentation did not follow a therapeutic algorithm or classification-based scoring method and was potentially influenced by higher degrees of kyphotic deformity at baseline in the PS group. Rather, based on individual preference, it also resulted in a form of surgical bias and heterogeneity for both groups. Our results may also be interpreted that for more severe cases of kyphotic deformity, percutaneous instrumentation was feasible and achieved better preservation of deformity correction at 3-month follow-up. Third, classifying posterior wall injury on CT was simplified to mere cortical disruption and morphological changes like height loss >1/5. Whether or not severity and extent of posterior wall injury (e.g., degree, extent of comminution, displacement or angulation) influence the risk for posterior cement leakage cannot be answered and could be further investigated. Fourth, the low sample size with one patient accounting for 2% of overall results and 4% of group results; while not statistically significant, mild complications were more than double the rate in the PS group compared to the BKP group. Considering the primary endpoint, our study has a power (1−β) of 0.386 with an α error probability of 0.136. To achieve an α error probability of 0.05 with the same power, it would have been necessary to include a total of 82 patients (41 patients in each group), which was not possible given the inclusion criteria applied. Fifth, the rather short follow-up, unable to determine long-term outcomes of the study population. Given these limitations, our findings must be interpreted with appropriate caution.
Conclusions
BKP with and without percutaneous posterior instrumentation with PS are both effective techniques in the surgical treatment of OVFs with posterior wall injury. Both techniques had similar 30-day morbidity and achieved significant improvements in early postoperative radiographic parameters versus their baseline values. When compared with percutaneously instrumented OVFs, standalone BKP was a less invasive surgical procedure associated with significantly shorter surgical times, shorter LOS, and less opioid requirement at discharge, that achieved equally good clinical outcomes at 3-month follow-up.
Acknowledgments
The authors would like to thank Mary Kemper for medical editing.
Funding: This work was supported by an institutional research grant from Medtronic to MM and EN.
Footnote
Reporting Checklist: The authors have completed the STROBE reporting checklist. Available at http://dx.doi.org/10.21037/jss-20-625
Data Sharing Statement: Available at http://dx.doi.org/10.21037/jss-20-625
Conflicts of Interest: All authors have completed the ICMJE uniform disclosure form (available at http://dx.doi.org/10.21037/jss-20-625). MM and EN report an institutional research grant from Medtronic during the conduct of the study. The other author has nothing to disclose.
Ethical Statement: The authors are accountable for all aspects of the work in ensuring that questions related to the accuracy or integrity of any part of the work are appropriately investigated and resolved. The study was conducted in accordance with the Declaration of Helsinki (as revised in 2013). The study was approved by the Ethical Committee of Northwestern and Central Switzerland (ID number EKNZ BASEC 2018-00185), and individual consent for this retrospective analysis was waived.
Open Access Statement: This is an Open Access article distributed in accordance with the Creative Commons Attribution-NonCommercial-NoDerivs 4.0 International License (CC BY-NC-ND 4.0), which permits the non-commercial replication and distribution of the article with the strict proviso that no changes or edits are made and the original work is properly cited (including links to both the formal publication through the relevant DOI and the license). See: https://creativecommons.org/licenses/by-nc-nd/4.0/.
References
- Ballane G, Cauley JA, Luckey MM, et al. Worldwide prevalence and incidence of osteoporotic vertebral fractures. Osteoporos Int 2017;28:1531-42. [Crossref] [PubMed]
- Bliuc D, Nguyen ND, Milch VE. Mortality Risk Associated With Low-Trauma Fracture in Men and Women. JAMA 2009;301:513-21. [Crossref] [PubMed]
- Center JR, Nguyen TV, Schneider D, et al. Mortality after all major types of osteoporotic fracture in men and women: an observational study. Lancet 1999;353:878-82. [Crossref] [PubMed]
- Lau E, Ong K, Kurtz S, et al. Mortality Following the Diagnosis of a Vertebral Compression Fracture in the Medicare Population. J Bone Joint Surg Am 2008;90:1479-86. [Crossref] [PubMed]
- Garfin SR, Yuan HA, Reiley MA. New technologies in spine: kyphoplasty and vertebroplasty for the treatment of painful osteoporotic compression fractures. Spine (Phila Pa 1976) 2001;26:1511-5. [Crossref] [PubMed]
- Lieberman IH, Dudeney S, Reinhardt MK, et al. Initial outcome and efficacy of “kyphoplasty” in the treatment of painful osteoporotic vertebral compression fractures. Spine (Phila Pa 1976) 2001;26:1631-8. [Crossref] [PubMed]
- Watts NB, Harris ST, Genant HK. Treatment of painful osteoporotic vertebral fractures with percutaneous vertebroplasty or kyphoplasty. Osteoporos Int 2001;12:429-37. [Crossref] [PubMed]
- Wardlaw D, Cummings SR, Van Meirhaeghe J, et al. Efficacy and safety of balloon kyphoplasty compared with non-surgical care for vertebral compression fracture (FREE): a randomised controlled trial. Lancet 2009;373:1016-24. [Crossref] [PubMed]
- Boonen S, Van Meirhaeghe J, Bastian L, et al. Balloon kyphoplasty for the treatment of acute vertebral compression fractures: 2-year results from a randomized trial. J Bone Miner Res 2011;26:1627-37. [Crossref] [PubMed]
- Van Meirhaeghe J, Bastian L, Boonen S, et al. A randomized trial of balloon kyphoplasty and nonsurgical management for treating acute vertebral compression fractures: vertebral body kyphosis correction and surgical parameters. Spine (Phila Pa 1976) 2013;38:971-83. [Crossref] [PubMed]
- Beall DP, Chambers MR, Thomas S, et al. Prospective and Multicenter Evaluation of Outcomes for Quality of Life and Activities of Daily Living for Balloon Kyphoplasty in the Treatment of Vertebral Compression Fractures: The EVOLVE Trial. Neurosurgery 2019;84:169-78. [Crossref] [PubMed]
- Schnake KJ, Blattert TR, Hahn P, et al. Classification of Osteoporotic Thoracolumbar Spine Fractures: Recommendations of the Spine Section of the German Society for Orthopaedics and Trauma (DGOU). Global Spine J 2018;8:46S-49S. [Crossref] [PubMed]
- Harrington KD. Major neurological complications following percutaneous vertebroplasty with polymethylmethacrylate : a case report. J Bone Joint Surg Am. 2001;83:1070-3. [Crossref] [PubMed]
- McArthur N, Kasperk C, Baier M, et al. 1150 kyphoplasties over 7 years: indications, techniques, and intraoperative complications. Orthopedics 2009;32:90. [Crossref] [PubMed]
- Nieuwenhuijse MJ, Van Erkel AR, Dijkstra PDS. Cement leakage in percutaneous vertebroplasty for osteoporotic vertebral compression fractures: identification of risk factors. Spine J 2011;11:839-48. [Crossref] [PubMed]
- Ponnusamy KE, Iyer S, Gupta G, et al. Instrumentation of the osteoporotic spine: biomechanical and clinical considerations. Spine J 2011;11:54-63. [Crossref] [PubMed]
- Weiser L, Dreimann M, Huber G, et al. Cement augmentation versus extended dorsal instrumentation in the treatment of osteoporotic vertebral fractures: a biomechanical comparison. Bone Joint J 2016;98-B:1099-105. [Crossref] [PubMed]
- Abdelgawaad AS, Ezzati A, Govindasamy R, et al. Kyphoplasty for osteoporotic vertebral fractures with posterior wall injury. Spine J 2018;18:1143-8. [Crossref] [PubMed]
- Parreira PCS, Maher CG, Megale RZ, et al. An overview of clinical guidelines for the management of vertebral compression fracture: a systematic review. Spine J 2017;17:1932-8. [Crossref] [PubMed]
- Blattert TR, Schnake KJ, Gonschorek O, et al. Nonsurgical and Surgical Management of Osteoporotic Vertebral Body Fractures: Recommendations of the Spine Section of the German Society for Orthopaedics and Trauma (DGOU). Global Spine J 2018;8:50S-55S. [Crossref] [PubMed]
- von Elm E, Altman DG, Egger M, et al. The Strengthening the Reporting of Observational Studies in Epidemiology (STROBE) statement: guidelines for reporting observational studies. Ann Intern Med 2007;147:573-7. [Crossref] [PubMed]
- Vaccaro AR, Oner C, Kepler CK, et al. AOSpine thoracolumbar spine injury classification system: fracture description, neurological status, and key modifiers. Spine (Phila Pa 1976) 2013;38:2028-37. [Crossref] [PubMed]
- Kuss O, Blettner M, Borgermann J. Propensity Score: an Alternative Method of Analyzing Treatment Effects. Dtsch Arztebl Int 2016;113:597-603. [Crossref] [PubMed]
- Moser M, Schmassmann P, Noger M, et al. Usefulness of Fat Suppression Magnetic Resonance Imaging of Osteoporotic Vertebral Fractures in Preventing Subsequent Fractures After Kyphoplasty. World Neurosurg 2019;125:e764-73. [Crossref] [PubMed]
- Kuklo TR, Polly DW, Owens BD, et al. Measurement of thoracic and lumbar fracture kyphosis: evaluation of intraobserver, interobserver, and technique variability. Spine (Phila Pa 1976) 2001;26:61-5; discussion 66. [Crossref] [PubMed]
- Landriel Ibañez FA, Hem S, Ajler P, et al. A new classification of complications in neurosurgery. World Neurosurg 2011;75:709-15. [Crossref] [PubMed]
- Ostelo RWJG, de Vet HCW. Clinically important outcomes in low back pain. Best Pract Res Clin Rheumatol 2005;19:593-607. [Crossref] [PubMed]
- Tassemeier T, Haversath M, Schutzbach M, et al. Who benefits more in osteoporotic fractures: Pedicle screw instrumentation or kyphoplasty for American Society of Anesthesiologists II/III patients? J Craniovertebr Junction Spine 2018;9:232-7. [Crossref] [PubMed]
- Zhan Y, Jiang J, Liao H, et al. Risk Factors for Cement Leakage After Vertebroplasty or Kyphoplasty: A Meta-Analysis of Published Evidence. World Neurosurg 2017;101:633-42. [Crossref] [PubMed]
- Liu H, Zhang J, Liang X, et al. Distribution Pattern Making Sense: Patients Achieve Rapider Pain Relief with Confluent Rather Than Separated Bilateral Cement in Percutaneous Kyphoplasty for Osteoporotic Vertebral Compression Fractures. World Neurosurg 2019;126:e1190-6. [Crossref] [PubMed]
- Wang B, Zhao CP, Song LX, et al. Balloon kyphoplasty versus percutaneous vertebroplasty for osteoporotic vertebral compression fracture: a meta-analysis and systematic review. J Orthop Surg Res 2018;13:264. [Crossref] [PubMed]
- Ren H, Shen Y, Zhang YZ, et al. Correlative factor analysis on the complications resulting from cement leakage after percutaneous kyphoplasty in the treatment of osteoporotic vertebral compression fracture. J Spinal Disord Tech 2010;23:e9-15. [Crossref] [PubMed]
- Krueger A, Bliemel C, Zettl R, et al. Management of pulmonary cement embolism after percutaneous vertebroplasty and kyphoplasty: a systematic review of the literature. Eur Spine J 2009;18:1257-65. [Crossref] [PubMed]
- Krüger A, Zettl R, Ziring E, et al. Kyphoplasty for the treatment of incomplete osteoporotic burst fractures. Eur Spine J 2010;19:893-900. [Crossref] [PubMed]
- Liu T, Li Z, Su Q, et al. Cement leakage in osteoporotic vertebral compression fractures with cortical defect using high-viscosity bone cement during unilateral percutaneous kyphoplasty surgery. Medicine (Baltimore) 2017;96:e7216. [Crossref] [PubMed]
- Walter J, Haciyakupoglu E, Waschke A, et al. Cement leakage as a possible complication of balloon kyphoplasty--is there a difference between osteoporotic compression fractures (AO type A1) and incomplete burst fractures (AO type A3.1)? Acta Neurochir (Wien) 2012;154:313-9. [Crossref] [PubMed]
- Goldstein CL, Brodke DS, Choma TJ. Surgical Management of Spinal Conditions in the Elderly Osteoporotic Spine. Neurosurgery 2015;77 Suppl 4:S98-107. [Crossref] [PubMed]
- Burval DJ, McLain RF, Milks R, et al. Primary pedicle screw augmentation in osteoporotic lumbar vertebrae: biomechanical analysis of pedicle fixation strength. Spine (Phila Pa 1976) 2007;32:1077-83. [Crossref] [PubMed]
- Becker S, Chavanne A, Spitaler R, et al. Assessment of different screw augmentation techniques and screw designs in osteoporotic spines. Eur Spine J 2008;17:1462-9. [Crossref] [PubMed]
- Janssen I, Ryang YM, Gempt J, et al. Risk of cement leakage and pulmonary embolism by bone cement-augmented pedicle screw fixation of the thoracolumbar spine. Spine J 2017;17:837-44. [Crossref] [PubMed]
- Martín-Fernández M, Lopez-Herradon A, Pinera AR, et al. Potential risks of using cement-augmented screws for spinal fusion in patients with low bone quality. Spine J 2017;17:1192-9. [Crossref] [PubMed]
- Chang MC, Kao HC, Ying SH, et al. Polymethylmethacrylate augmentation of cannulated pedicle screws for fixation in osteoporotic spines and comparison of its clinical results and biomechanical characteristics with the needle injection method. J Spinal Disord Tech 2013;26:305-15. [Crossref] [PubMed]
- Hu MH, Wu HTH, Chang MC, et al. Polymethylmethacrylate augmentation of the pedicle screw: the cement distribution in the vertebral body. Eur Spine J 2011;20:1281-8. [Crossref] [PubMed]
- Noriega D, Marcia S, Theumann N, et al. A prospective, international, randomized, noninferiority study comparing an implantable titanium vertebral augmentation device versus balloon kyphoplasty in the reduction of vertebral compression fractures (SAKOS study). Spine J 2019;19:1782-95. [Crossref] [PubMed]