Clinical and radiographic outcomes using third-generation bioactive glass as a bone graft substitute for multi-level anterior cervical discectomy and fusion—a retrospective case series study
Introduction
Anterior discectomy and interbody fusion (ACDF) is routinely engaged to decompress the central spinal canal and neuroforamina by direct removal of disc fragments, radial osteophytes, and uncovertebral joint osteophytes. Indirect decompression of the canal and neuroforamina can also be achieved simultaneously during restoration of disc space height as well as normal segmental lordosis. ACDF is well established as reliably effective in achieving improved patient outcomes and satisfaction for singe level and multi-level cervical spondylosis (1,2).
Following anterior decompression the aims of cervical interbody fusion are to provide stabilization, maintain segmental lordosis, and restored anatomic disc space height. There are various grafting techniques that have been described to accomplish these goals (3-5). One of the grafting materials used for promoting fusion is autogenous iliac crest bone graft (ICBG). It is well supported that autogenous grafts demonstrate improved rates of fusion when compared to allografts (6-8); however, separate incision bone graft harvesting techniques are associated with donor site pain (9). Due to the donor site morbidity of iliac crest harvest, bone substitute alternatives have become increasingly attractive. One such alternative, bioactive glass, has shown the ability to produce an environment conducive for bone formation and has been utilized in various specialties, including ENT and Dentistry, over the last twenty years as a synthetic bone graft substitute (10).
Biomaterials have evolved over the last fifty years, from generation to generation through bioengineering. The materials produced demonstrated biological inertness with body fluids (first-generation), the production of a strong tissue-implant bond (second-generation) and genetic activation of specific cell pathway (third-generation) (11). Bioactive glasses have converged into the third-generation with the ability to activate genes and stimulate bone formation (11).
Spinal fusion requires a complex cascade of biologic events and osseous union is not always successful. The successful progression to fusion and healing is influenced by multiple factors. The type of graft and interbody spacer impacts clinical and radiographic outcomes (12,13). Porous PEEK implants used with autograft have been shown to have a high rate of fusion and improved clinical outcomes (14,15). The purpose of this retrospective case series study was to evaluate the safety and effectiveness of a third-generation bioactive glass synthetic bone graft replacement (11,16,17) as a method of facilitating spinal fusion at multiple levels in patients with symptomatic cervical degenerative disc disease. We present the following study in accordance with the STROBE reporting checklist (available at http://dx.doi.org/10.21037/jss-20-645).
Methods
Patient demographics
Thirty-nine consecutive patients underwent multi-level instrumented ACDF between May 2016 and December 2017 by a single fellowship-trained spine surgeon. Inclusion criteria included adults (>18 years old) with symptomatic degenerative cervical disc disease as manifest from the C3 and T1 spinal levels. All patients manifested associated radiculopathy, myelopathy, or both. Subjects experienced neck and radicular upper extremity pain for a minimum interval of 6 weeks preoperatively and that was recalcitrant to standard nonoperative treatment modalities (to include structured physical therapy, activity modification, and anti-inflammatory medications, injection-based modalities, and other similar approaches). All patients demonstrated radiographic evidence of cervical disc disease that was established and visualized on plain static and dynamic radiographs (Figure 1), as well as magnetic resonance imaging (MRI).
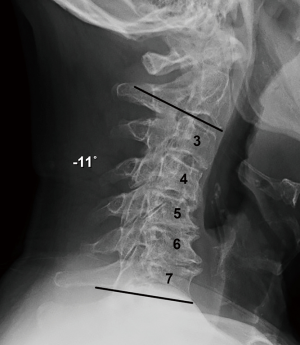
Patients were excluded from the study if they had cervical spinal conditions other than multi-level symptomatic degenerative disc disease. Other exclusion criteria include a history of discitis, or a medical condition that required medication, such as steroids or nonsteroidal anti-inflammatory medications that could interfere with fusion.
Clinical and radiographic follow-up
Data was acquired and compiled preoperatively, intraoperatively, and at standard postoperative intervals of 3, 6, 12, and 24 months. Safety and effectiveness were evaluated in the course of the above. Operative procedure details and adverse events were recorded. Standardized outcome measures including Neck Disability Index (NDI) (18,19) and axial cervical pain and radicular arm pain visual analog numerical scores (VAS) were used to benchmark preoperative and postoperative condition in this context. Neurological status was determined by measuring three objective clinical findings: motor function, sensory function, and deep tendon reflexes. Success for each of these three objective findings was based on postoperative maintenance or improvement in condition compared with preoperative status. Overall neurological status success was determined by maintenance or improvement in all three clinical findings.
Routine radiographs were used to assess spinal alignment, arthrodesis, bone ingrowth, subsidence, and any visible shift or position changes of the implant. Neutral anteroposterior, lateral, and dynamic flexion-extension lateral radiographs were obtained at each study point. C2-C7 lordosis angle (Figure 2), fusion segment lordosis, proximal and distal adjacent lordosis angle, and T1 slope was measured on neutral lateral radiographs. Intradiscal distraction and subsidence were measured by assessing the vertical distance between the midpoints of the adjacent vertebral endplates. Fusion success was defined radiographically by meeting four criteria: (I) evidence of bridging bone (based on radiographic evidence of a continuous bony connection from the superior vertebral body to the inferior vertebral body; (II) evidence of radiolucency (no greater than 25% of the superior or inferior implant-vertebral interface); (III) no motion greater than 5 degrees on dynamic flexion/extension radiographs and (IV) no change in interspinous process distance >1 mm on dynamic flexion/extension radiographs (20) (Figure 3A,B). All radiographs were reviewed by an independent observer.
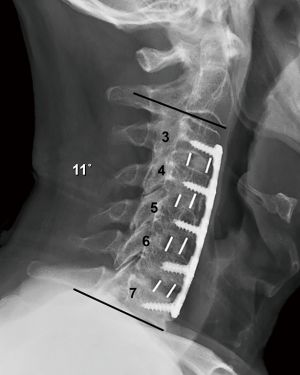
Surgical technique
A usual anterior muscle splitting surgical dissection of the left neck was performed in each case. The majority of the disc resection was performed with curettes in order to minimize heat-induced compromise of the adjacent endplate vascularity. High-speed burr was used for removal of uncovertebral joint spurs and radial osteophytes; however high-speed burr use was limited and osseous contact times were purposefully controlled with the goal of meticulously maintaining endplate integrity while minimizing any potential thermal compromise of adjacent osseous vascular integrity.
A new third-generation of bioactive glass bone graft putty was utilized in this study (BioSphere® Putty, Synergy Biomedical, Wayne, PA, USA). This putty utilizes 45S5 bioactive glass particles with a unique, spherical shape mixed with a phospholipid carrier. A range of 1.5–2.0 cc of synthetic graft putty was used at each treated cervical level. Porous PEEK interbody spacers of mixed size were used at every level (Cohere, NuVasive San Diego, CA, USA). The interbody spacers central graft windows were uniformly packed with the bone graft substitute along with the porous surface interstices of the interbody implant so as to optimize material fill and complete endplate contact with the bioactive glass.
Statistical methods
Preoperative and postoperative clinical outcomes and radiographic measures were compared using paired t-tests. The threshold for statistical significance was set at P<0.05.
Ethical statement
This manuscript reports on the outcomes of a retrospective IRB approved case series study. Ethical approval was obtained from the Hughston Foundation Institutional Review Board and the participants gave informed consent. The study conformed to the provisions of the Declaration of Helsinki (as revised in 2013).
Results
Demographics
Thirty-nine patients (21 females and 18 males) were treated with bioactive glass as a bone graft substitute The average age was 60.1 years and the average body mass index was 30.6 (+/− 1.2 kg/m2). Eight patients (20%) used tobacco within 6 months. All patients were followed for a minimum of 6 months for mean of 16.0 months (range, 6 to 36 months); no patients were lost to follow-up in this series.
Surgery
Seventeen patients (43%) underwent a two-level fusion; 12 (31%) underwent a three-level fusion; 9 (23%) underwent a four-level fusion; and 1 (3%) underwent a five-level fusion.
Clinical outcomes
NDI improved significantly from the preoperative scores (18.92) and these improvements were maintained out to 1 year (7.4) (P<0.001) (Table 1). VAS neck pain scores also demonstrated statistically significant improvement from the preoperative scores averaging 3.97 to an average score of 0.74 at 1 year (P<0.001). Similarly, VAS arm pain scores improved significantly from the preoperative scores (5.47) to (0.13) (P<0.001) at 1 year. Neurologic status was maintained or improved at all postoperative visits.

Full table
Radiographic outcomes
All patients demonstrated osseous integration of the interbody spacers to the vertebral endplates and trabeculated new bone formation across the fused interspace. No radiographic lucencies developed at the interface of the porous PEEK implant to the vertebral endplate. There was no motion on dynamic flexion/extension radiographs, migration of the implants, broken screws, or plates.
There was a significant improvement in the fusion segment lordosis when comparing preoperative to the first and final postoperative radiographs (Pre-Post P<0.001, Pre-Final P<0.001) (Table 2). Both proximal and distal adjacent segment lordosis improved from preoperative to final postoperative radiographs but were not statistically significant (Pre-Final P>0.05).
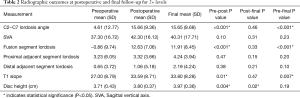
Full table
C2−C7 lordosis angle, as well as T1 slope, significantly improved from the preoperative to first postoperative and final radiographs (Pre-Post P<0.001, Pre-Final P<0.001). Disc height remained unchanged from the first postoperative visit to the final follow-up (Post-Final P<0.02). Sagittal vertical axis (SVA) demonstrated improvement from preoperative to final postoperative radiographs, although failed to demonstrate statistical significance (Pre-Final P>0.05).
Adverse events
There were no unanticipated adverse device effects reported in the course of this study. Dysphagia, local swelling, incision site edema, incision site erythema, and incision site infection are events that are commonly associated with an ACDF. There were no serious adverse events such as life-threatening events, deaths, and events that required hospitalization or an emergency room visit.
Discussion
Synthetic alternatives to autologous and allogeneic bone grafts have been developed to avoid the complication of graft harvesting, to increase rates of fusion, and to improve clinical outcomes. Bioactive glasses are a unique class of bioceramics that were discovered in 1969 and provided an alternative to inert implant materials.
Bioactive glasses are a group of synthetic silica-based bioactive materials with bone bonding properties (11,16). Bioactive glasses are typically composed of 4 different oxide materials: SiO2, CaO, Na2O, and P2O5. They have several unique properties compared with other synthetic bioresorbable bioactive ceramics, such as calcium phosphates, hydroxyapatite (HA), and tricalcium phosphate (TCP). Importantly, bioactive glasses bond with bone more rapidly than other bioceramics and are dependent on a hydroxy carbonate apatite (HCA) layer produced on the surface of the bioactive glass particle after contact with body fluids (11,21).
Bioactive glass induces a high local turnover of bone formation and resorption. With the transition from the first and second to the third-generation bioactive glasses, a focus on using spherical particles in the third-generation was created. As compared to the monolithic shapes of older generation materials, the spherical particles create a uniform geometry, thus increasing the surface area and allowing for greater bioactive glass-body fluid interaction producing a strong bond to host tissue and bone formation (11). The optimization of the particle shape and size improves the bone healing properties of bioactive glass. When used in spherical, particulate form bioactive glass can stimulate osteogenesis. Studies have shown that the third-generation material is highly osteoconductive and promotes the growth of new bone on its surface (22-25).
The chemical composition strongly determines the glass structure, the biocompatibility, the degradation rate, and the ease of processing (scaffolds fabrication, and sintering) (17). There is a characteristic and active balance between new bone formation and bioactive glass resorption that is required to achieve a successful arthrodesis.
In this study, the high rates of fusion (and subsequent maintenance of restored disc space height and sagittal lordosis) were associated with the use of the unique porous surface of the interbody fusion cages and a novel third-generation bioactive glass bone graft replacement. In a challenging healing environment (26-32), all patients (including smokers and obesity) were implanted with a novel, spherical form of bioactive glass alone as an autograft replacement. Bioactive glass is a unique material with bioactive and osteostimulatory properties that allow it to take an active role in bone healing (22). In the current study, bioactive glass in a unique, spherical form is a safe and effective and proven bone graft material for interbody fusion in complex multi-level cervical spine surgery. Limitations of this study include the sample size and the length of follow-up. A larger sample of patients and follow-up out to 24 months is needed to confirm the significance and relevance of our study.
Conclusions
Third-generation bioactive glass in spherical, particulate form has been shown to be a promising and effective method of facilitating anterior intervertebral spinal fusion and of decreasing pain and improving clinical outcomes after multi-level anterior cervical fusion surgery.
Acknowledgments
I would like to thank J. Kenneth Burkus, MD, and the team at the Hughston Foundation for their assistance and support in preparing this report.
Funding: None.
Footnote
Reporting Checklist: The authors have completed the STROBE reporting checklist. Available at http://dx.doi.org/10.21037/jss-20-645
Data Sharing Statement: Available at http://dx.doi.org/10.21037/jss-20-645
Conflicts of Interest: The authors have completed the ICMJE uniform disclosure form (available at http://dx.doi.org/10.21037/jss-20-645). LEW reports personal fees from SeaSpine, personal fees from NuVasive, outside the submitted work. GG has no conflicts of interest to declare.
Ethical Statement: The authors are accountable for all aspects of the work in ensuring that questions related to the accuracy or integrity of any part of the work are appropriately investigated and resolved. This manuscript reports on the outcomes of a retrospective IRB approved case series study. Ethical approval was obtained from the Hughston Foundation Institutional Review Board and the participants gave informed consent. The study conformed to the provisions of the Declaration of Helsinki (as revised in 2013).
Open Access Statement: This is an Open Access article distributed in accordance with the Creative Commons Attribution-NonCommercial-NoDerivs 4.0 International License (CC BY-NC-ND 4.0), which permits the non-commercial replication and distribution of the article with the strict proviso that no changes or edits are made and the original work is properly cited (including links to both the formal publication through the relevant DOI and the license). See: https://creativecommons.org/licenses/by-nc-nd/4.0/.
References
- Buttermann GR. Anterior cervical discectomy and fusion outcomes over 10 Years: A prospective study. Spine 2018;43:207-14. [Crossref] [PubMed]
- Gore DR, Sepic SB. Anterior cervical fusion for degenerated or protruded discs. A review of on-hundred forty-six patients. Spine 1984;9:667-71. [Crossref] [PubMed]
- Brodke DS, Zdeblick TA. Modified Smith-Robinson procedure for anterior cervical discectomy and fusion. Spine 1992;17:S427-30. [Crossref] [PubMed]
- Emery SE, Bolesta MJ, Banks MA, et al. Robinson anterior cervical fusion comparison of the standard and modified techniques. Spine 1994;19:660-3. [Crossref] [PubMed]
- Krag MH, Robertson PA, Johnson CC, et al. Anterior cervical fusion using a modified tricortical bone graft: A radiographic analysis of outcome. J Spinal Disord 1997;10:420-30. [Crossref] [PubMed]
- Miller LE, Block JE. Safety and effectiveness of bone allografts in anterior cervical discectomy and fusion surgery. Spine 2011;36:2045-50. [Crossref] [PubMed]
- Shapiro S. Banked fibula and the locking anterior cervical plate in anterior cervical fusions following cervical discectomy. J Neurosurg 1996;84:161-5. [Crossref] [PubMed]
- Zdeblick TA, Ducker TB. The use of freeze-dried allograft bone for anterior cervical fusions. Spine 1991;16:726-9. [Crossref] [PubMed]
- Gruskay JA, Basques BA, Bohl DD, et al. Short-term adverse events, length of stay, and readmission after Iliac bone graft for spinal surgery. Spine 2014;39:1718-24. [Crossref] [PubMed]
- Baino F, Hamzehlou S, Kargozar S. Bioactive Glasses: Where Are We and Where Are We Going? J Funct Biomater 2018;9:25. [Crossref] [PubMed]
- Hench LL. The story of Bioglass. J Mater Sci Mater Med 2006;17:967-78. [Crossref] [PubMed]
- Burkus JK, Dryer RF, Arnold PM, et al. Clinical and radiographic outcomes in patients undergoing single-level anterior cervical arthrodesis: A prospective trial comparing allograft to a reduced dose of rhBMP-2. Clin Spine Surg 2017;30:E1321-32. [Crossref] [PubMed]
- Liu JM, Xiong X, Peng AF, et al. A comparison of local bone graft with PEEK cage versus iliac bone graft used in anterior cervical discectomy and fusion. Clin Neurol Neurosurg 2017;155:30-5. [Crossref] [PubMed]
- Burkus JK. Early outcomes of anterior cervical discectomy and fusion using a porous PEEK interbody fusion device. J Spine Neurosurg 2018;7:2. [Crossref]
- Burkus JK, Rehak CR. Anterior cervical discectomy and fusion using porous PEEK implants at levels adjacent to a previous fusion. J Spine Neurosurg 2019;8:3.
- Hench LL, Jones JR. Bioactive glasses: Frontiers and challenges. Front Bioeng Biotechnol 2015;3:194. [Crossref] [PubMed]
- Krishnan V, Lakshmi T. Bioglass: A novel biocompatible innovation. J Adv Pharm Technol Res 2013;4:78-83. [Crossref] [PubMed]
- Vernon H, Mior S. The Neck Disability Index: A study of reliability and validity. J Manipulative Physiol Ther 1991;14:409-15. [PubMed]
- Westaway MD, Stratford PW, Binkley JM. The patient-specific functional scale: Validation of its use in persons with neck dysfunction. J Orthop Sports Phys Ther 1998;27:331-8. [Crossref] [PubMed]
- Riew KD, Yang JJ, Chang DG, et al. What is the most accurate radiographic criterion to determine anterior cervical fusion? Spine J 2019;19:469-75. [Crossref] [PubMed]
- Oonishi H, Kushitani S, Yasukawa E, et al. Particulate bioglass compared with hydroxyapatite as a bone graft substitute. Clin Orthop Relat Res 1997;316-25. [Crossref] [PubMed]
- Westerlund LE, Borden M. Clinical experience with the use of spherical bioactive glass putty for cervical and lumbar interbody fusion. J Spine Surg 2020;6:49-61. [Crossref] [PubMed]
- Ilharreborde B, Morel E, Fitoussi F, et al. Bioactive glass as a bone substitute for spinal fusion in adolescent idiopathic scoliosis: a comparative study with iliac crest autograft. J Pediatr Orthop 2008;28:347-51. [Crossref] [PubMed]
- Furusawa T, Mizunuma K. Osteoconductive properties and efficacy of resorbable bioactive glass as a bone-grafting material. Implant Dent 1997;6:93-101. [Crossref] [PubMed]
- Rantakokko J, Frantzén JP, Heinänen J, et al. Posterolateral spondylodesis using bioactive glass S53P4 and autogenous bone in instrumented unstable lumbar spine burst fractures. A prospective 10-year follow-up study. Scand J Surg 2012;101:66-71. [Crossref] [PubMed]
- Berman D, Oren JH, Bendo J, et al. The effect of smoking on spinal fusion. Int J Spine Surg 2017;11:29. [Crossref] [PubMed]
- Brown CW, Orme TJ, Richardson HD. The rate of pseudarthrosis (surgical nonunion) in patients who are smokers and patients who are nonsmokers: a comparison study. Spine (Phila Pa 1976) 1986;11:942-3. [Crossref] [PubMed]
- Hilibrand AS, Fye MA, Emery SE, et al. Impact of smoking on the outcome of anterior cervical arthrodesis with interbody or strut-grafting. J Bone Joint Surg Am 2001;83:668-73. [Crossref] [PubMed]
- Laratta JL, Reddy HP, Bratcher KR, et al. Outcomes and revision rates following multilevel anterior cervical discectomy and fusion. J Spine Surg 2018;4:496-500. [Crossref] [PubMed]
- Nandyala SV, Marquez-Lara A, Fineberg SJ, et al. Comparison of revision surgeries for one- to two-level cervical TDR and ACDF from 2002 to 2011. Spine J 2014;14:2841-6. [Crossref] [PubMed]
- Narain AS, Hijji FY, Haws BE, et al. Impact of body mass index on surgical outcomes, narcotics consumption, and hospital cost following anterior cervical discectomy and fusion. J Neurosurg Spine 2018;28:160-6. [Crossref] [PubMed]
- Veeravagu A, Cole T, Jiang B, et al. Revision rates and complication incidence in single- and multilevel anterior cervical discectomy and fusion procedures: an administrative database study. Spine J 2014;14:1125-31. [Crossref] [PubMed]