Lateral lumbar interbody fusion using a cellular allogeneic bone matrix in the treatment of symptomatic degenerative lumbar disc disease and lumbar spinal instability
Introduction
Degenerative disc disease can evolve into neurocompressive disorders, such as spinal stenosis, and lumbar instability patterns, such as spondylolisthesis and scoliosis. These conditions can be associated with disabling pain and neurological deficits. Most patients respond to nonsurgical treatments, but for those with intractable chronic low back pain, lumbar fusion surgery is a viable treatment option. The goals of fusion surgery include the restoration of segmental anatomic disc space height and sagittal contour and the elimination of painful motion by creating an osseous bridge across lumbar motion segments (1-4).
Numerous interbody devices, varying in size, shape and material, are available for lumbar interbody fusion that reflect adaptations for different surgical approaches and biomechanical needs (5-7). However, even in conjunction with advanced biomaterials and interbody cage designs, the fusion process in the spine is complex and does not always heal successfully (8,9). Creating new bone formation across a spinal motion segment occurs in a challenging healing environment (10). A successful interbody fusion induces new bone formation that bridges the disc space that does not normally support viable bone. Autogenous bone or an autograft substitute in combination with an interbody fusion device is commonly used (11-15).
A unique cellular allograft has been developed (16) which demonstrates both osteoconductive and inductive capabilities. This specialized allograft provides a natural, osteoconductive bone scaffold composed of demineralized cortical and mineralized cortical and cancellous bone. The allograft tissues are engineered to a microparticulate bone-scaffold size ranging from 100 to 300 µm, which is optimized for bone regeneration (17). In addition, a nondimethyl sulfoxide (DMSO), innovative cellular preservation technique is used to support cell viability (18). This cellular allograft material also has been shown osteoinductive capability by its ability to induce simultaneous activity of osteoclasts and osteoblasts, demonstrating rapid healing of bone defects (13).
Our objective was to evaluate the clinical and radiographic outcomes and the potential adverse events related to cellular allogeneic bone matrix as an alternative to autogenous iliac crest bone graft (ICBG) or other costly biomaterials or growth factors in patients undergoing lateral lumbar interbody fusion (LLIF) with posterior supplemental fixation. The LLIF technique was chosen for study because it is a minimally invasive technique for interbody fusion using a retroperitoneal approach to the anterior spinal column that circumvents some of the challenges and morbidity risk of anterior or posterior lumbar interbody fusion techniques
We present the following article in accordance with the STROBE reporting checklist (available at https://dx.doi.org/10.21037/jss-21-28).
Methods
Sixty-seven consecutive patients who underwent lumbar interbody fusion through a minimally invasive lateral approach were eligible for inclusion in this retrospective, nonrandomized clinical and radiographic outcome study. All patients were treated at a single institution by a single fellowship-trained attending physician. We report the outcomes of this retrospective IRB-approved case series study. Ethical approval was obtained from the Sterling IRB Institutional Review Board (8618) and the participants gave informed consent. The study conformed to the provisions of the Declaration of Helsinki (as revised in 2013). Patients were examined preoperatively and postoperatively at 3 and 12 months. The patients’ demographic data, smoking status, and existing medical conditions were recorded.
Inclusion criteria
Patients undergoing single- and multilevel fusions were included in this study. All patients, who were treated between January 2016 and December 2017, were between the ages of 26 and 85 years old and had symptomatic degenerative disc disease at the T12 to L5 levels. Inclusion was generally based on presentation of symptomatic chronic low back pain and identification of level specific degenerative changes on radiographical imaging. All had symptoms of low back pain for at least 6 months before their surgery, which were recalcitrant to nonoperative treatment modalities, including physical therapy, bed rest, and anti-inflammatory medications. Patients were included if they had objective signs of neural compression, resulting from generalized disc degeneration or other specific radiological findings such as stenosis. All patients had plain radiographic findings of single-level or multi-level multilevel disc disease and had undergone at least one additional confirmatory neuroradiographic study, such as magnetic resonance imaging (MRI), computed tomography (CT)-enhanced myelography, or discography. All patients were considered candidates for a single-level or multilevel LLIF procedure. Patients were excluded from the study if they had spinal conditions other than symptomatic degenerative disc disease, spondylolisthesis, or scoliosis.
Exclusion criteria was as follows: symptomatic disc disease at a level other than T12 to L5; obesity (>40% ideal body weight); pregnancy; metabolic bone diseases, such as Paget disease or osteomalacia; or any medical condition that required medication, such as steroids or nonsteroidal anti-inflammatory medications that could interfere with fusion. Patients were excluded with the diagnosis of fracture, infection, or neoplasm.
Allogeneic matrix
The allogeneic matrix is composed of small particles of cortical and cancellous bone, which is mineralized and demineralized (100 to 300 µm) with viable osteoprogenitor cells. Cell viability is assessed on an automated cell counter (MOXI flow, Orflo Technologies, Ketchum, ID). Cell viability is assessed via an exclusion assay using propidium iodide (stain for dead cells) and the Coulter Principle. Acceptance criterion is 75% viability with a total cell count above 150,000 cells/cc. On average, cell viability is 89.4%±5.7% and the average cell count is 3.03×105±6.18×104. The quality control assessment occurs a minimum of 20 days after cryopreservation. Cell viability was not assessed intraoperatively.
Operative technique
All patients underwent a minimally invasive extreme lateral retroperitoneal approach to the lumbar spine (LLIF); no out-patient surgeries were performed. All LLIF fusions were supplemented with postural segmental spinal fixation. An anterior discectomy was carried out by an en bloc lateral discectomy through a single annular opening. An incision was made in the anulus fibrosus, and the nucleus pulposus and the cartilaginous end plates were removed under direct visualization. The vertebral end plates were preserved. The disc space was distracted to an anatomic height equal to the adjacent spinal motion segments. A lateral titanium interbody fusion device (Globus Medical, Inc., Audubon, PA, USA) was filled with the cellular allogeneic bone matrix (VIA® Graft, VIVEX Biologics, Inc., Atlanta, GA, USA) and was inserted into each disc space. No other bone- or cell-based products were used. This bone matrix was used exclusively as the biologic adjuvant to support the interbody fusion in our patients.
Clinical outcome assessment
Self-administered clinical outcome measures, including Oswestry Disability Index (ODI) (19), the 36-Item Short Form Survey (SF-36) (20), and visual analog scale (VAS) scores of back and leg pain (21), were completed preoperatively and at 3 and 12 months after surgery. The ODI questionnaire was used to measure the effects of back pain associated with activities of daily living. To assess back and leg pain intensity, VAS scores were determined using a 100-point scale. A 10-point frequency score and 10-point intensity score for pain were summed. The result was multiplied to create a 100-point scale.
Radiographic assessment
Adjacent levels were deemed fused if there was de novo bone formation crossing the disc space and no evident subsidence or motion at the disc space. The presence of continuous trabecular bone formation between the vertebral bodies was assessed using plain radiographs and CT scans. At each surgical level, thin-slice (1 mm overlapping) CT scans with coronal and sagittal plane reconstructions were used to assess bridging bone and allograft incorporation. Intradiscal motion and implant migration was assessed on the dynamic radiographs. Fusion was defined as bridging bone connecting the adjacent vertebral bodies either through the implants or around the implants, <5° of angular motion, ≤3 mm of translation, and an absence of radiolucent lines around more than 50% of either implant. Fusion was assessed at 3 and 12 months and was considered successful only if all four criteria were achieved. All radiographic studies were assessed by an independent physician.
Adverse events
All patients were monitored for the presence of adverse events during their procedure and during routine or unanticipated office visits. All complications were documented, including subsequent surgical interventions.
Statistical analysis
Statistical significance of the observed changes in ODI and VAS back scores were assessed using paired 2-sided t-tests. Significance was ascribed to P values <0.05.
Results
Sixty-seven patients with symptomatic degenerative disc disease between the T12 and L5 levels who underwent LLIFs were evaluated for this study. No patients were lost to follow-up; all patients in the study had a minimum follow-up of 12 months. Thirty-four males (51%) and33 females (49%) underwent surgery at an average age of 66.8 years (Table 1). An average of 2.25 levels was treated per patient for a total of 151 levels.
Table 1
Characteristics | No. of patients |
---|---|
Age [range] | 66.8 [45–85] |
Mean height (range), inches | 67.4 (61.0–77.0) |
Mean weight (range), lbs | 203.7 (125.0–315.0) |
Sex (%) | |
Male | 34 (51.0) |
Female | 33 (49.0) |
Tobacco use (%) | 13 (19.4) |
Total Waddell signs [% negative] | 67 [100] |
BMI (range) | 30.3 (21.0–39.7) |
Diabetes (%) | 11 (16.4) |
BMI, body mass index.
Radiographic outcomes
Fusion outcomes were assessed with both dynamic plain radiographs and by CT scans (Figure 1). In one patient, fusion was not achieved by surgery at 1 level; 66/67 (98.5%) patients demonstrated at least a partial fusion at all levels treated. Fusion was achieved at 142 levels (142/151; 94%) at 3 months after surgery. No deterioration in fusion status occurred between 3 and 12 months; all of these levels remained fused Eight levels (8/151; 5.3%) showed partial fusions at 3 months and remained unfused at last follow-up at 12 months (Figure 2). One level was a failed fusion at 3 and 12 months.
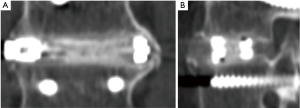

There was no significant change in the rates of fusion when single-level fusions (15/16; 93.8%) were compared with multi-level fusions (127/135; 94.1%) (P>0.999; Fisher’s exact test) (Figure 3). Similarly, there was no significant change in fusion rates when comparing 1- and 2-level fusion (69/71; 97.2%) with 3- or more level fusions (74/80; 92.5%), (P=0.283 Fisher’s exact test).
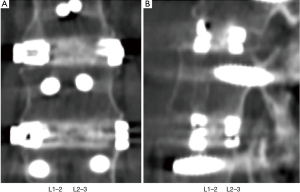
Clinical outcomes
Patients rated their back pain duration and intensity on a scale from 0–100, with a score of 0 representing “no pain” and a score of 100 representing “pain as bad as it could be.” In those patients who showed radiographic evidence of fusion, the mean back and leg pain scores showed improvement from preoperative scores at both 3 and 12 months (P<0.001) (Figure 4). Functional outcomes showed similar clinical success and were documented by significant improvement in both SF-36 and ODI scores in the group of patients with fusions at 3 months and at 12 months after surgery (P<0.001). For those patients who had only a partial interbody fusion, a significant VAS pain reduction response was seen at both 3 and 12 months (P<0.001); however, SF-36 and ODI scores did not attain a significant improvement in this incomplete fusion group.
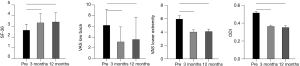
Adverse events
No adverse events occurred during surgery or during the postoperative period. No patient underwent a secondary surgical procedure.
Discussion
During the spinal fusion procedure, biocompatible materials that can be osteoconductive or osteoinductive are placed in varying anatomic positions spanning an intervertebral motion segment. The healing process is a race balancing resorption of the graft material, cellular apoptosis, autologous cell migration revascularization, and the formation of new bone growing through the graft connecting the vertebral bodies. It is important to optimize the cell volumes placed in each interbody implant to consistently induce bone formation (8,21). Autologous bone from the iliac crest has been considered the gold standard for most types of bone graft procedures because of its osteogenic properties coupled with its cell content (12). ICBGs are effective, but they are associated with a number of problems, such as postsurgical pain and morbidity, as well as infection risk at the donor site (22,23).
Cellular allogeneic bone matrices have been widely used in spinal surgery (12,13,15). These specialized allografts provide a unique, moldable, 3-dimensional structure capable of conforming to the full geometry of the recipient site and providing both an inductive and conductive scaffold for bone growth. Importantly, these allografts contain osteogenic cell precursors and cytokines that have been shown to be as effective as ICBG in promoting bone healing (9,11,16).
Techniques and materials for the preservation of allogeneic cells have been developed to allow for long-term storage and recovery of viable bone cells. The cellular bone matrices used in this study involved an innovative cellular preservation technique that supports cell viability. The cell components were collected from the vertebral body region of each donor. The age criteria were limited to donors between the ages of 15 and 55. In addition, flow cytometry analysis and quality control processes verified a viable cell population for osteogenic supplementation.
In this retrospective study, a cellular allogeneic bone matrix used as a singular biological adjuvant in a broad population of patients undergoing minimally invasive spine fusion with an LLIF technique supported mechanical fusion and symptomatic relief. Statistically significant improvement in clinical and functional outcomes in our patients occurred in the SF-36, VAS, and ODI scores at 12 months. No adverse events were identified in our study from the use of viable allografts.
Outcomes at 12 months demonstrated the minimally invasive LLIF surgical approach to be clinically and radiographically successful in treating patients with single-level or multi-level lumbar degenerative disc disease and spinal deformities. The cellular allogeneic bone matrix used exclusively in this study demonstrated a potentially viable implant alternative to autograft for achieving successful radiographic and clinical outcomes.
This study has several limitations. It is a retrospective examination of a series of consecutive patients treated with LLIF by a single surgeon at a single clinical site. The study was not randomized nor controlled by comparing operative patients with patients treated nonoperatively or with a control arm (fusion without cellular allograft). This case series is a descriptive observational study. This research design is most useful for describing the potential effectiveness of new interventions and serves as a means of initially reporting on novel diagnostic or therapeutic strategies. The case series is an efficient study design; the decision about the treatment regimen remains with the surgeon and the patient. The lack of a comparison group distinguishes cohort studies from case series. A randomized controlled trial carries the highest level of evidence; however, for technical or ethical reasons, it is not an appropriate design for all clinical research. Case series have methodological limitations with regard to establishing the relation between specific treatments and outcomes. However, they can be instrumental in generating a hypothesis that can be tested in further analytic studies.
The duration of follow-up was limited to 1 year. Despite the short follow-up, the intent of the study was to assess the presence or absence of fusion and how this affected pain and patient function. To this end, a cellular allograft as a sole biologic adjunct to discectomy, restoration of disc height and stabilization resulted in fusion or partial fusion in 98% of levels fused. These results were durable at 12 months. Further follow up is necessary to assess this approach in treating patients with degenerative disc disease who do not respond to nonoperative management.
Conclusions
LLIF showed good clinical outcomes with a low complication rate. Although cellular allografts are no substitute for appropriate patient selection and meticulous surgical technique, this study provides evidence that successful interbody fusion can be achieved using cellular bone allogeneic matrix rivaling that of autograft or growth factor administration. Cellular allogeneic bone matrices provide a biologic strategy for achieving successful clinical and radiological outcomes in LLIF surgery, particularly in multilevel surgeries in which fusion rates are lower.
Regarding clinical relevance, a cellular allogeneic bone matrix is an alternative bone grafting implant for improving the osseointegration and fusion rates of interbody devices in patients undergoing LLIF at multiple levels. Successful fusion documented by CT scans was correlated with functional improvement and pain reduction.
Acknowledgments
The authors thank the Hughston Foundation for assistance with medical illustrations and manuscript editing.
Funding: This study was supported by VIVEX Biologics, Inc., Atlanta, Georegia, USA, which contributed to study design, data monitoring, statistical analysis and reporting of results, and paid for independent data collection, core laboratory, and EDC services.
Footnote
Reporting Checklist: The authors have completed the STROBE reporting checklist. Available at https://dx.doi.org/10.21037/jss-21-28
Data Sharing Statement: Available at https://dx.doi.org/10.21037/jss-21-28
Conflicts of Interest: All authors have completed the ICMJE uniform disclosure form (available at https://dx.doi.org/10.21037/jss-21-28). TCT, MD, is shareholder, royalty earner, consultant, and married to employee of VIVEX Biologics, Inc.; HTT, MD, is the Chief Medical Officer and Medical Director of VIVEX Biologics, Inc.; JKB, MD, is a consultant with VIVEX Biologics, Inc. The authors have no conflicts of interest to declare.
Ethical Statement: The authors are accountable for all aspects of the work in ensuring that questions related to the accuracy or integrity of any part of the work are appropriately investigated and resolved. Ethical approval was obtained from the Sterling IRB Institutional Review Board (8618) and the participants gave informed consent. The study conformed to the provisions of the Declaration of Helsinki (as revised in 2013).
Open Access Statement: This is an Open Access article distributed in accordance with the Creative Commons Attribution-NonCommercial-NoDerivs 4.0 International License (CC BY-NC-ND 4.0), which permits the non-commercial replication and distribution of the article with the strict proviso that no changes or edits are made and the original work is properly cited (including links to both the formal publication through the relevant DOI and the license). See: https://creativecommons.org/licenses/by-nc-nd/4.0/.
References
- Alimi M, Hofstetter CP, Cong GT, et al. Radiological and clinical outcomes following extreme lateral interbody fusion. J Neurosurg Spine 2014;20:623-35. [Crossref] [PubMed]
- Carlson BB, Saville P, Dowdell J, et al. Restoration of lumbar lordosis after minimally invasive transforaminal lumbar interbody fusion: a systematic review. Spine J 2019;19:951-8. [Crossref] [PubMed]
- Li HM, Zhang RJ, Shen CL. Differences in radiographic and clinical outcomes of oblique lateral interbody fusion and lateral lumbar interbody fusion for degenerative lumbar disease: a meta-analysis. BMC Musculoskelet Disord 2019;20:582. [Crossref] [PubMed]
- Malham GM, Ellis NJ, Parker RM, et al. Maintenance of Segmental Lordosis and Disk Height in Stand-alone and Instrumented Extreme Lateral Interbody Fusion (XLIF). Clin Spine Surg 2017;30:E90-8. [Crossref] [PubMed]
- Kettler A, Wilke HJ, Dietl R, et al. Stabilizing effect of posterior lumbar interbody fusion cages before and after cyclic loading. J Neurosurg 2000;92:87-92. [PubMed]
- Lund T, Oxland TR, Jost B, et al. Interbody cage stabilisation in the lumbar spine: biomechanical evaluation of cage design, posterior instrumentation and bone density. J Bone Joint Surg Br 1998;80:351-9. [Crossref] [PubMed]
- Nomoto EK, Fogel GR, Rasouli A, et al. Biomechanical Analysis of Cortical Versus Pedicle Screw Fixation Stability in TLIF, PLIF, and XLIF Applications. Global Spine J 2019;9:162-8. [Crossref] [PubMed]
- Gao Y, Li J, Cui H, et al. Comparison of intervertebral fusion rates of different bone graft materials in extreme lateral interbody fusion. Medicine (Baltimore) 2019;98:e17685 [Crossref] [PubMed]
- LoGuidice A, Houlihan A, Deans R. Multipotent adult progenitor cells on an allograft scaffold facilitate the bone repair process. J Tissue Eng 2016;7:2041731416656148 [Crossref] [PubMed]
- Seo DK, Kim MJ, Roh SW, et al. Morphological analysis of interbody fusion following posterior lumbar interbody fusion with cages using computed tomography. Medicine (Baltimore) 2017;96:e7816 [Crossref] [PubMed]
- Kerr EJ 3rd, Jawahar A, Wooten T, et al. The use of osteo-conductive stem-cells allograft in lumbar interbody fusion procedures: an alternative to recombinant human bone morphogenetic protein. J Surg Orthop Adv 2011;20:193-7. [PubMed]
- Lee DD, Kim JY. A comparison of radiographic and clinical outcomes of anterior lumbar interbody fusion performed with either a cellular bone allograft containing multipotent adult progenitor cells or recombinant human bone morphogenetic protein-2. J Orthop Surg Res 2017;12:126. [Crossref] [PubMed]
- Tally WC, Temple HT, Subhawong TY, et al. Transforaminal Lumbar Interbody Fusion With Viable Allograft: 75 Consecutive Cases at 12-Month Follow-up. Int J Spine Surg 2018;12:76-84. [Crossref] [PubMed]
- Tessitore E, Molliqaj G, Schaller K, et al. Extreme lateral interbody fusion (XLIF): A single-center clinical and radiological follow-up study of 20 patients. J Clin Neurosci 2017;36:76-9. [Crossref] [PubMed]
- Tohmeh AG, Watson B, Tohmeh M, et al. Allograft cellular bone matrix in extreme lateral interbody fusion: preliminary radiographic and clinical outcomes. ScientificWorldJournal 2012;2012:263637 [Crossref] [PubMed]
- Martin WB, Sicard R, Namin SM, et al. Methods of Cryoprotectant Preservation: Allogeneic Cellular Bone Grafts and Potential Effects. Biomed Res Int 2019;2019:5025398 [Crossref] [PubMed]
- Malinin TI, Carpenter EM, Temple HT. Particulate bone allograft incorporation in regeneration of osseous defects; importance of particle sizes. Open Orthop J 2007;1:19-24. [Crossref] [PubMed]
- Martin W B, Sicard R, Namin S M, et al. Methods of cryoprotectant preservation: Allogeneic cellular bone grafts and potential effects. Biomed Res Int 2019;2019:5025398 [Crossref] [PubMed]
- Fairbank JC, Couper J, Davies JB, et al. The Oswestry low back pain disability questionnaire. Physiotherapy 1980;66:271-3. [PubMed]
- McHorney CA, Ware JE Jr, Lu JF, et al. The MOS 36-item Short-Form Health Survey (SF-36): III. Tests of data quality, scaling assumptions, and reliability across diverse patient groups. Med Care 1994;32:40-66. [Crossref] [PubMed]
- Keorochana G, Setrkraising K, Woratanarat P, et al. Clinical outcomes after minimally invasive transforaminal lumbar interbody fusion and lateral lumbar interbody fusion for treatment of degenerative lumbar disease: a systematic review and meta-analysis. Neurosurg Rev 2018;41:755-70. [Crossref] [PubMed]
- Kim DH, Rhim R, Li L, et al. Prospective study of iliac crest bone graft harvest site pain and morbidity. Spine J 2009;9:886-92. [Crossref] [PubMed]
- Schwartz CE, Martha JF, Kowalski P, et al. Prospective evaluation of chronic pain associated with posterior autologous iliac crest bone graft harvest and its effect on postoperative outcome. Health Qual Life Outcomes 2009;7:49. [Crossref] [PubMed]