MAZOR-X robotic-navigated percutaneous C2 screw placement for hangman’s fracture: a case report
Introduction
Hangman’s fractures are defined by fractures through the lamina, articular facets, pedicles and/or pars of C2 (1). The Levine-Edwards classification of hangman’s fractures (2) identifies Type I and adequately reduced type II as stable fractures amenable to non-operative treatment with a rigid collar or halo immobilization. Cases that fail non-operative treatment, or select type II, type IIa and type III fractures usually require surgical fusion. Surgical management options for hangman’s fractures include C2-3 anterior cervical discectomy and fusion, or C1-2 or C1-3 posterior cervical fusion with or without extension to the occiput (3-5). Each of these surgical options involves some loss of flexion/extension and rotational motion with its associated morbidity. Direct C2 transpedicular fixation has been used to overcome these shortcomings (6), however, variable anatomy, risk of neurovascular injury and motion associated with an unstable fracture limit this approach. Buchholz et al. demonstrated a minimally-invasive percutaneous direct screw fixation using O-arm neuronaviation as a novel motion-preserving and physiologic operation with shorter operative times, faster recovery, decreased blood loss and decreased muscle disruption (1).
Robotic-assisted spine surgery using the MAZOR-X system (Mazor Robotics Ltd., Caesarea, Israel) was FDA approved in 2004 and has the potential to decrease radiation exposure and operative time while increasing accuracy (7,8). The robotic platform includes an operative planning module which uses a high-resolution pre-operative CT scan. Surgical screws can be specified for each level, and 3-dimentional renderings can be used to visualize and specify screw dimensions, insertion points and trajectories. Critical structures can thus be avoided, and ideal screw sizes and trajectories chosen to improve safety and accuracy. Two recent retrospective studies compared MAZOR-X to O-arm neuronavigation for spine surgery and respectively found improved precision and accuracy (9), and decreased fluoroscopy time, time-per-screw placement and patient length of stay (10). Robotic assistance is gaining wider applicability, however, its use in the cervical spine is still limited. Here we report the first case to our knowledge of MAZOR-X navigated percutaneous placement of C2 screws for a hangman’s fracture.
We present the following case in accordance with the CARE reporting checklist (available at https://dx.doi.org/10.21037/jss-20-676).
Case presentation
A 27-year-old female with no pertinent past medical history presented after an ATV accident with a Levine-Edwards type II hangman’s fracture. All procedures performed in this study were in accordance with the ethical standards of the institutional research committee and with the Helsinki Declaration (as revised in 2013). Written informed consent was obtained from the patient. She was treated non-operatively for 3 months in a rigid Aspen Vista cervical collar, however she continued to have significant neck pain despite conservative management. She developed upper extremity paresthesias which were described as tingling down the right triceps, dorsal forearm and 4th and 5th digits. A CT cervical spine at 3 months showed non-union and increased distance between bony fragments at the fracture site (Figure 1A-1C). She also had motion of the pars fracture on cervical flexion and extension X-rays. Having tried and failed non-operative management she was indicated for surgery.
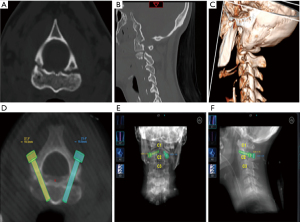
Pre-operatively high-resolution 3D CT scans were obtained, and screw trajectories were planned using the MAZOR-X software (Figure 1D-1F). Bilateral 5 mm lag screws were used (Synthes). The patient was placed in a Mayfield head frame in the prone position on an open Jackson surgical table. Intraoperative fluoroscopic images were used to register the anatomy to preoperative CT. Once accuracy and plan were confirmed, the MAZOR-X robotic arm was sent into position.
Using the robotic arm as a guide, transverse 1cm incisions were made bilaterally 3 cm lateral from midline at approximately the C3 level. We then docked the drill guide onto the pars surface and confirmed position with intraoperative fluoroscopy. Once docked we drilled across the fracture site using a Stryker cordless high-speed drill (Figure 2A). We again visualized this with intraoperative fluoroscopy to verify no movement of the fracture site. A K-wire was then placed down the trajectory across the fracture site (Figure 2B). A tap was inserted over the K-wire and we tapped across the fracture site. As a final step our lag screw was placed over the K-wire using fluoroscopy to confirm good position of instrumentation across the fracture site (Figure 2C).

The operative setup allowed for robotic arm placement as well as X-ray confirmation (Figure 2D). We performed an intraoperative O arm spin to confirm good position of instrumentation and confirmed bilateral screw placement and reduction of fracture. Incisions were closed with Dermabond. Post-operative X-rays confirmed proper screw placement and fracture reduction (Figure 2E). The patient recovered well post-operatively with resolution of her right-sided paresthesias. She was discharged home on post-operative day 1. The patient was seen at a 6 month follow up from surgery. She reported no neck pain or paresthesias and has normal range of motion. She has returned to work and is pleased with her care.
Discussion
Owing to variable spine anatomy particularly after trauma, direct placement of C2 pars or pedicle screws is technically challenging (11-13). C2 pars and pedicle screw fixation have been described for Hangman’s fractures (11,13). Open transpedicular screw fixation yields excellent anatomical and functional results with motion preservation in patients with Levine-Edwards Type II fractures (14), and appears to be safer and more cost effective than C2-3 anterior plating.
Percutaneous transpedicular screw fixation has also been described using preoperative imaging for planning and intraoperative fluoroscopy for verification of anatomic locations and screw position (15). Postoperative CT imaging showed 17 of 20 screws (85%) were placed satisfactorily and 3 of 20 screws (15%) showed a perforation of the pedicle wall (<2 mm), but all screws otherwise showed proper alignment with solid fusion and patients reported being asymptomatic from cortical breach (15).
Buchholz et al. reported the first series of direct, percutaneous, minimally invasive, CT-based-neuro-navigation-assisted repair of Hangman’s fractures (1). The percutaneous technique was as safe and effective as the open procedures previously reported, without the pedicle wall perforation rate of 15% reported in the percutaneous approach taken by Wu et al. (15). In this report we showed for the first time robotic-assisted percutaneous minimally invasive repair of a Hangman’s fracture.
Current robotic platforms include Renaissance (Medtronic), SpineAssist (Medtronic), ROSA Spine (Zimmer Biomet), Excelcius GPS (Globus) and MAZOR-X (Medtronic). Detailed technical notes on the MAZOR-X method have been recently published (16). Briefly, the robotic software suite calculates the most efficient and safest screw trajectories. After planning and registration, the robotic effector arm is sent sequentially to pre-determined screw entry points and provides a targeted docking arm through which cannulas and instruments are placed along pre-planned trajectories for accurate screw placement (10,16).
Despite its higher upfront costs and technical requirements, robotic-assisted spine surgery is gaining traction due to its increased accuracy and shorter operative times. In the cervical spine, accurate screw placement is imperative due to smaller bony structures and increased risk of vascular and visceral injuries. A recent prospective randomized controlled study of robotic-assisted versus traditional fluoroscopic-guided screw placement in the cervical spine found higher accuracy, decreased blood loss and shorter hospital stay despite similar operative times (17), demonstrating a clear clinical advantage to using this strategy.
In our case, robotic assistance provided a minimally invasive surgical option to treat the fracture and preserve motion in a young active patient. Placement of C2 percutaneous pars screws, a technically challenging surgery, was aided by robotic assistance. This surgical description is not meant as a recommendation of treatment for Hangman fractures but rather presents a possible option in the appropriate patient. Further work is needed to refine indications and establish protocols for robotic assistance in the cervical spine.
Conclusions
We describe the first case of MAZOR-X robotic-assisted percutaneous screw placement for stabilization of a Levine-Edwards Type II C2 hangman’s fracture with good radiographic fracture reduction. Robotic-assisted surgery for upper cervical injuries has potential for significant clinical impact in spine surgery.
Acknowledgments
Funding: None.
Footnote
Reporting Checklist: The authors have completed the CARE reporting checklist. Available at https://dx.doi.org/10.21037/jss-20-676
Conflicts of Interest: Both authors have completed the ICMJE uniform disclosure form (available at https://dx.doi.org/10.21037/jss-20-676). The authors have no conflicts of interest to declare.
Ethical Statement: The authors are accountable for all aspects of the work in ensuring that questions related to the accuracy or integrity of any part of the work are appropriately investigated and resolved. All authors confirm they have no conflicts of interest. All procedures performed in this study were in accordance with the ethical standards of the institutional research committee and with the Helsinki Declaration (as revised in 2013). Written informed consent was obtained from the patient.
Open Access Statement: This is an Open Access article distributed in accordance with the Creative Commons Attribution-NonCommercial-NoDerivs 4.0 International License (CC BY-NC-ND 4.0), which permits the non-commercial replication and distribution of the article with the strict proviso that no changes or edits are made and the original work is properly cited (including links to both the formal publication through the relevant DOI and the license). See: https://creativecommons.org/licenses/by-nc-nd/4.0/.
References
- Buchholz AL, Morgan SL, Robinson LC, et al. Minimally invasive percutaneous screw fixation of traumatic spondylolisthesis of the axis. J Neurosurg Spine 2015;22:459-65. [Crossref] [PubMed]
- Levine AM, Edwards CC. The management of traumatic spondylolisthesis of the axis. J Bone Joint Surg Am 1985;67:217-26. [Crossref] [PubMed]
- Chittiboina P, Wylen E, Ogden A, et al. Traumatic spondylolisthesis of the axis: a biomechanical comparison of clinically relevant anterior and posterior fusion techniques. J Neurosurg Spine 2009;11:379-87. [Crossref] [PubMed]
- Boullosa JL, Colli BO, Carlotti CG Jr, et al. Surgical management of axis' traumatic spondylolisthesis (Hangman's fracture). Arq Neuropsiquiatr 2004;62:821-6. [Crossref] [PubMed]
- Wang S, Wang Q, Yang H, et al. A novel technique for unstable Hangman's fracture: lag screw-rod (LSR) technique. Eur Spine J 2017;26:1284-90. [Crossref] [PubMed]
- Rajasekaran S, Vidyadhara S, Shetty AP. Intra-operative Iso-C3D navigation for pedicle screw instrumentation of hangman's fracture: a case report. J Orthop Surg (Hong Kong) 2007;15:73-7. [Crossref] [PubMed]
- D'Souza M, Gendreau J, Feng A, et al. Robotic-Assisted Spine Surgery: History, Efficacy, Cost, And Future Trends. Robot Surg 2019;6:9-23. [Crossref] [PubMed]
- Meyers JE, Khan A, Pollina J. Robotic Guidance for the Insertion of Posterior Pedicle Screws: 2-Dimensional Operative Video. Oper Neurosurg (Hagerstown) 2019;16:766-7. [Crossref] [PubMed]
- Mao G, Gigliotti MJ, Myers D, et al. Single-Surgeon Direct Comparison of O-arm Neuronavigation versus Mazor X Robotic-Guided Posterior Spinal Instrumentation. World Neurosurg 2020;137:e278-85. [Crossref] [PubMed]
- Khan A, Meyers JE, Yavorek S, et al. Comparing Next-Generation Robotic Technology with 3-Dimensional Computed Tomography Navigation Technology for the Insertion of Posterior Pedicle Screws. World Neurosurg 2019;123:e474-81. [Crossref] [PubMed]
- Bristol R, Henn JS, Dickman CA. Pars screw fixation of a hangman's fracture: technical case report. Neurosurgery 2005;56:E204 [PubMed]
- Borne GM, Bedou GL, Pinaudeau M. Treatment of pedicular fractures of the axis. A clinical study and screw fixation technique. J Neurosurg 1984;60:88-93. [Crossref] [PubMed]
- Ludwig SC, Kramer DL, Balderston RA, et al. Placement of pedicle screws in the human cadaveric cervical spine: comparative accuracy of three techniques. Spine (Phila Pa 1976) 2000;25:1655-67. [Crossref] [PubMed]
- ElMiligui Y, Koptan W, Emran I. Transpedicular screw fixation for type II Hangman's fracture: a motion preserving procedure. Eur Spine J 2010;19:1299-305. [Crossref] [PubMed]
- Wu YS, Lin Y, Zhang XL, et al. Management of hangman's fracture with percutaneous transpedicular screw fixation. Eur Spine J 2013;22:79-86. [Crossref] [PubMed]
- O'Connor TE, O'Hehir MM, Khan A, et al. Mazor X Stealth Robotic Technology: A Technical Note. World Neurosurg 2021;145:435-42. [Crossref] [PubMed]
- Fan M, Liu Y, He D, et al. Improved Accuracy of Cervical Spinal Surgery With Robot-Assisted Screw Insertion: A Prospective, Randomized, Controlled Study. Spine (Phila Pa 1976) 2020;45:285-91. [Crossref] [PubMed]