The use of minimally invasive interspinous process devices for the treatment of lumbar canal stenosis: a narrative literature review
Introduction
Lumbar canal stenosis (LCS) is a degenerative condition that leads to spinal canal narrowing (1). LCS can be debilitating and is often associated with intermittent neurogenic claudication (NC). Degenerative spondylolisthesis can present with or without instability (1-3).
With an aging population, LCS has become increasingly common. While most patients can be managed conservatively, symptomatic patients who are unable to tolerate the pain may require appropriate surgical intervention after failed trial of non-operative management. Open decompression seems to be most beneficial when NC is the predominant symptom (2) and may include excision of the spinous process, lamina and ligamentum flavum, while spinal arthrodesis may be necessary either prior to decompression or as a result of decompression for concomitant segment instability.
Interspinous process devices (IPD) are minimally invasive interspinous process implants that can provide symptom relief in LCS (4). Despite increasing use, the efficacy and safety of ISS over open decompression techniques are still debated. Furthermore, the wide variety of manufacturers promoting devices may increase difficulty for surgeons and interventionalists to choose the most appropriate implant. IPD includes two groups, namely interspinous distraction devices (IDD) and interspinous stabilizers (ISS). IDD are implanted to separate adjacent spinous processes to provide indirect decompression of neurological structures during spinal extension, preventing progressive collapse of foraminal height. Due to insertion technique and lack of fixation, IDD do not confer stabilization qualities and hence may loosen and generate pain. In addition to spinous process distraction, ISS involve fixing adjacent spinous processes with a bracing component, which provides an additional degree of dynamic stability to adjacent levels. Examples of IPD are shown in Table 1.
Table 1
Interspinous Distraction Device (IDD) | Interspinous Stabilizers (ISS) | |||
---|---|---|---|---|
Brand name | Manufacturer | Brand name | Manufacturer | |
Aperius ( |
Medtronic, Switzerland | Coflex ( |
Paradigm Spine, USA | |
In-Space ( |
Synthes, Germany | DIAM ( |
Medtronic Sofamor Danek, USA | |
Superion ( |
Vertiflex, USA | Wallis ( |
Zimmer, USA | |
X-Stop ( |
St Francis Medical technologies, USA |
DIAM, device for intervertebral assisted motion.
The aim of this study is to provide a comprehensive review of IPD and to investigate if 1. minimally invasive IDD can be an effective substitute for direct neural decompression and 2. ISS are appropriate substitutes for fusion after open decompression.
We present the following article in accordance with the Narrative Review reporting checklist (available at https://dx.doi.org/10.21037/jss-21-57).
Methods
Articles published up to 22nd January 2020 were obtained from PubMed search using the following keywords and Boolean operators: (Interspinous process devices OR interspinous distraction devices OR interspinous stabilizers). Relevant articles published in the English language were selected and critically reviewed. Both comparative and non-comparative studies with retrospective and prospective study designs were included.
Principles of treatment
The principle behind surgically treating LCS is decompression of central canal and foramen by preventing lordosis, and providing distraction of posterior elements (4). NC secondary to LCS is shown to be posture-dependent, where symptoms such as lower limb paraesthesia, pain and hypoesthesia are exacerbated in extension and relieved in flexion, known as the “shopping cart sign” (12). An MRI study demonstrated that extension or rotation of the lumbar spine reduces sagittal diameters and cross-sectional areas of the spinal canal and increased the thickness of ligamentum flavum (13). This was consistent with a cadaveric study that reported a 15% reduction in cross sectional area of the spinal canal when in extension (14). On the contrary, flexion had the opposite effects (13,14).
Treating NC with IDD achieves indirect decompression without jeopardizing spinal stability at the instrumented level. IDD not only restricts extension, but also preserves some degree of flexion at the instrumented level. By doing so, ligamentum flavum is stretched and its thickness reduced. Eventually, diameter of spinal canal is enlarged whilst allowing flexion to relieve the compression of nerve roots (4,15). It is noted that motion preservation at instrumented levels alleviate the stress concentrated at adjacent levels, thus reducing risk of adjacent level spondylosis (4,15,16) (Figure 1).
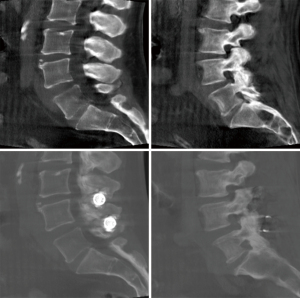
ISS not only maintain decompression of neural structures following open decompression, but also achieve some form of dynamic stabilization. Posterior element stabilization would be indicated if observed grade 1 spondylolisthesis is observed on pre-operative XR or if the spine was found to be unstable intraoperatively (17).
Indications
Current IPD indications vary slightly with implant type and are based on manufacturer recommendations. Common indications include treating skeletally mature patients suffering from symptomatic LCS at one or two levels as defined by history, physical examination and diagnostic radiological evidence of moderate disease using either magnetic resonance imaging (MRI) and/or computed tomography (CT) (5-11). Findings on CT or MRI include thickened ligamentum flavum, narrowed lateral recess, central canal or foraminal narrowing (5-11).
Other indications include (5-11):
- Soft disc protrusions with discogenic low back pain;
- Degenerative grade 1 spondylolisthesis with hyperlordotic curve;
- Degenerative disc disease with retrolisthesis;
- Interspinous pain arising from Baastrup syndrome;
- Degenerative scoliosis (Cobb angle ≤25o);
- Massive herniated disc or recurrent herniated disc;
- Failed 3 to 6 months of conservative management.
These indications were also supported by a survey-based study of orthopaedic surgeons performed by Siewe (18) in Germany to review the perspectives and limitations of IPD. A total 329 responses were received, with 164 (49.8%) stating that IPD is a treatment option for LCS, with 135/329 (41.0%) of respondents using them. It is reported that poor clinical experience (60%) and lack of evidence were main reasons for not using IPD. Interestingly, 87/329 (26.4%) of respondents prefer using IPD in combination with open decompression, while only 13/329 (4.0%) would use it as a stand-alone procedure (18).
Contraindications
Absolute contraindications to IPD use include:
- Cauda equina syndrome;
- Spinal fracture (vertebral body, spinous process, pars interarticularis, laminae);
Other relative contraindications include (5-11):
- More than two levels of moderate stenosis;
- Severe stenosis at any given level;
- Spondylolisthesis more severe than grade 1;
- Advanced deformity at the proposed level of implantation;
- Advanced osteoporosis;
- History of previous open decompression at targeted levels;
- Active systemic or localized infection localized at the proposed implantation site;
- Scoliosis (Cobb angle >25o);
- Morbid obesity (BMI >40 kg/m2);
- Known allergy to specific metal alloys;
- Back or leg pain of unclear etiology;
- Pregnancy;
- Bone immaturity.
Operative technique
Implantation technique varies across available manufacturers. It is recommended to follow manufacturer specific recommendations. In general, IPD can be inserted under local, regional or general anaesthesia, depending on number of implant, patient co-morbidities and preferences. Patients are generally positioned in a prone or lateral decubitus position (5-11).
Minimally invasive approaches without direct decompression, including both complete percutaneous and less invasive techniques, typically require some mild intravenous analgesia along with sedation. Complete percutaneous techniques using implants preserve the ligamentum flavum completely, while mini open techniques involve selective removal of hypertrophic ligamentum flavum. A low dose pre-procedural fluoroscopic image is taken to confirm the correct targeted level. Local anaesthetic is injected into the subcutaneous layer, deep paraspinal muscles and periarticular regions at the targeted level. A small paraspinal stab skin incision is made before introducing a 6-mm K-wire into the interspinous space of the targeted level. Appropriate positioning is confirmed by further low dose CT scans. Incremental 2 mm soft tissue dilation is performed, with the largest size of 12 mm kept in situ. Using fluoroscopic guidance, various sized probes are advanced through the dilator to release interspinous ligament and measure the ideal size of IDD. After appropriate measurements are taken, the sized IDD is mounted and deployed into the interspinous space. The wire, dilator and holder are then removed. Haemostasis is achieved and layered closure of skin follows. Another post procedure CT scan is performed to confirm optimal positioning of IDD and assess for immediate complications (19) (Figure 2).
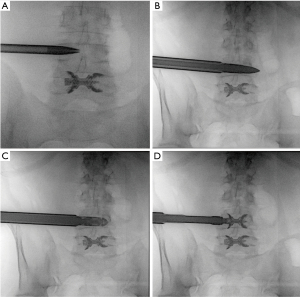
Implantation of ISS, such as Coflex, generally involves general anaesthesia and a midline incision of approximately 4 centimetres over the level of spinal stenosis. Periosteal dissection of paraspinal muscles is performed to access and remove interspinous ligaments and their bony attachments. Adequate decompression is achieved via bilateral partial laminotomies or laminectomies, as well as removing ligamentum flavum. Foraminotomies can also be concomitantly performed to ensure nerve roots are freely mobile. Then, the optimal size of ISS is measured in slight flexion using the trial inserter. The implant is then inserted between spinous processes and tightened wings with clamps (20,21).
Patient selection
Risk factors for complications
Gazeri (22) conducted a retrospective study on 1,108 patients receiving eight different IPD for degenerative disease and suggest that over-distraction of spinous processes and poor bone density were risk factors for complications, including spinous process fractures, dura mater tears, implant dislocation, recurrence of symptoms and revision surgery. Kim (23) performed a prospective study of 39 IPD patients with LCS and compared patients with and without spinous process fractures. Kim (23) reported that degenerative spondylolisthesis (100% vs. 33%, P<0.001) was a risk factor for periprosthetic fractures, while reduced bone mineral density and osteoporosis showed no significant associations. There is a current paucity of literature on the extent to which these suggested risk factors cause complications.
Patient selection is thus imperative in identifying patients who would most benefit from IPD and those with increased risks of adverse outcomes. In 2009, Barbagallo (24) introduced an anatomic scoring system that helped stratify the risk of spinous process fractures in IPD patients (Table 2).
Table 2
Score | Inferior SP morphology | Accessible SP length | Interspinous area shape |
---|---|---|---|
1 | Concave | Entire length | Parallel |
2 | Straight | Posterior 2/3 | Posterior V shape |
3 | Convex/dysmorphic | Posterior 1/3 | – |
Interpretation: Scores of 3–4 indicates suitable conditions, Scores of 5–6 indicates risky conditions, Scores of 7–8 indicates relative contraindication to the implantation of IPD. SP, spinous process.
Patient selection to benefit
Another important consideration during patient selection is LCS severity. In order to gain maximal benefit, IPD are indicated for mild to moderate symptomatic LCS and not severe disease. Schizas (25) proposed a grading classification that defines stenosis based on morphology rather than surface measurements (Table 3). This method had a moderate to substantial inter- and intra-observer agreement, which is a notable improvement from less reliable dural sac cross-sectional areas (DSCSA) measurements.
Table 3
Grading | Description |
---|---|
A | No or minor stenosis; clear sign of cerebrospinal fluid (CSF) visible inside dural space with heterogeneous distribution of rootlets |
B | Moderate stenosis; dural sac is completely occupied by rootlets, but can still be individualized |
C | Severe stenosis; rootlets are unrecognizable, with dural demonstrating homogeneous gray signal with no CSF visible |
D | Extreme stenosis; in additional to no rootlets being recognizable, there is no epidural fat posteriorly |
Interpretation: Grade A and B may be managed optimally with non-operative measurements; Grade C and D more likely to require decompression for symptomatic relief.
Biomechanical analysis
The simplest way to demonstrate biomechanical advantage of IPD is directly measuring the DSCSA. An increase in DSCSA would indicate decompression of impinged nerves and hence relief of clinical symptoms. Akazawa (26) performed a study on 17 patients receiving X-Stop IDD. MRI images were performed preoperatively, at 1 week, 3 months and 2 years post operatively to determine the effect of IPD on DSCSA. Akazawa (26) found a statistically significant 37% (P=0.045) increase in DSCSA at 1 week post operation, although this was not maintained at 3 months (P=0.586) and 2 years (P=0.884). While no IPD had deviated from the interspinous process, Akazawa (26) postulated that non-significant results at 3 months were likely due to spinous process resorption and subsequent implant subsidence. Nandakumar (27) also studied the X-Stop IDD and concluded a significant DSCSA increase from 109 to 177 mm2 (P<0.001) at 2 years in the standing position. Other clinical studies have shown improvements in DSCSA for up to 1 year in patients receiving Aperius IDD (28,29) (Figure 3).
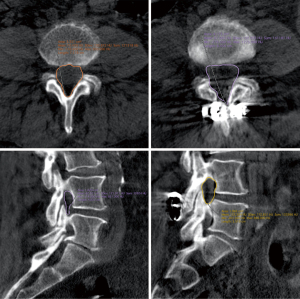
A recently published study by Hjaltadottir (30) replicated a more realistic approach to measuring DSCSA by adopting axial loading MRI imaging in 19 Aperius IDD patients and 13 traditional decompression patients. Hjaltadottir (30) concluded that Aperius IDD had no significant increase in DSCSA at the operated level (mean increase: 3 mm2, P=0.42), but demonstrated significant decrease in adjacent levels (mean decrease: 10 mm2, P=0.04). This was compared to open decompression, which revealed significant improvements of DSCSA at the operated level (mean: 74 mm2, P<0.001), with a non-significant increase at the adjacent level (mean 13 mm2, P=0.47). The results highly suggest that DSCSA improvements were greater in open decompression and hence more likely to provide symptomatic relief.
However, an increased DSCSA may not always be an accurate measure of IPD efficacy to treat LCS. This is because some LCS patients with radiological evidence may be asymptomatic and remain so, without requiring further treatment. The presence of nerve impingement and hence neurophysiological changes may be a more important determinant of IPD efficacy. A prospective study by Schizas (31) studied the neurophysiological effect of interspinous distraction by X-Stop IDD. Schizas (31) found that in single-level disease, an 8-mm distraction yielded similar improvement in motor evoked potentials in all severities of LCS when compared to open decompression. However, distraction >8 mm was less effective when compared to open decompression (P<0.05). Schizas (31) postulated that distraction >8 mm at the instrumented level may cause hyperextension of adjacent segments even in a non-weightbearing situation, thus causing a decrease in DSCSA and subsequently deleterious neurophysiological effects. In multilevel disease, open decompression was significantly more effective than any degree of distraction (P<0.001).
Another way to determine the biomechanical effect of IPD is via the efficacy of preserving and restricting motions that alleviates and exacerbates LCS symptoms (flexion and extension/rotation) respectively. Byun (32) conducted a finite element analysis of Coflex ISS by applying mechanical loads dependent on postural changes. Byun (32) found that Coflex ISS significantly restrained L4/L5 displacement in extension (24.5%) and lateral bending (44.5%), while also reducing average intradiscal pressures by 63% with various movements, when compared to a control group. Flexion was reduced marginally by 1.3%. However, the study observed intensive stresses (120 MPa) exerted by IPD on the base of spinous process during extension, which reasonably explains the common site of spinous process fracture during follow-up. A more recent study by Chen (33) showed supporting evidence for Coflex ISS restraining extension and lateral bending range of motion (ROM), and a 49.7% reduction of flexion ROM at the instrumented level when compared to control models. Coflex ISS were used in both studies, but implanted at different levels (Byun: L4/L5, Chen: L3/L4) (32,33). This suggests that the instrumented level may influence the biomechanical effects of IPD.
However, Chen (33) further assessed the ROM of non-instrumented adjacent segments and found consistent increase in flexion (16–20%), extension (33–35%) and lateral bending (4%) ROM. This increased ROM was attributed to a compensatory mechanism adopted in adjacent segments as a result of increased stiffness at the instrumented level. However, Wan (34) disputed this result by concluding that X-Stop IDD did not alter kinematics of adjacent levels. At the cranial adjacent segment, there was no significant difference before and after X-Stop IDD implantation in terms of extension (1.5ovs. 1.5o, P=0.994), flexion (1.1ovs. 1.3o, P=0.748), left-right lateral flexion (2.5ovs. 2.2o, P=0.951) and left-right twisting (1.8ovs. 1.8o, P=0.998). Similarly, no significant difference was found at the caudal adjacent segment before and after X-Stop IDD implantation in terms of extension (2.4ovs. 2.3o, P=0.994), flexion (2.3ovs. 2.5o, P=0.963), left-right lateral flexion (1.9ovs. 2.3o, P=0.619) and left-right twisting (2.8ovs. 3.7o, P=0.996). More studies are required to determine the presence of compensatory mechanisms of the lumbar spine to maintain ROM and the risk factors associated with development of adjacent segment spondylosis as a result of increased adjacent segment ROM during follow-up.
Interspinous process devices and its clinical outcomes
Reported clinical outcomes include the Oswestry Disability Index (ODI) (35), Rowland Disability Questionnaire (RDQ) (36), Visual Analogue Scale (VAS) for back and leg pain (37) and Zurich Claudication Questionnaire (ZCQ) (38). The ODI, RDQ and ZCQ are validated objective patient reported clinical outcome (PROM) measurement tools, while the VAS is a subjective indication of back and leg pain.
Common complications include treatment failure resulting in persistent or new onset pain, implant loosening with or without dislocation, and spinous process fracture. Reoperations are mainly indicated for these complications. The insertion of IPD converts spinous processes from naturally tension-bearing structures into compression-loading structures, which can induce fractures (39). The risk of fractures is further increased with factors, including osteoporosis, over-distraction, inappropriate device size and poor surgical technique (23). In fact, Kim (40) reported stronger association between IPD and early postoperative spinous process fracture than previously reportedly. These fractures, which are often minimally or undisplaced, present with mild or no localized pain and are easily obscuring by radio-opaque metallic wings of IPD on plain radiographs. Treatment failure and implant dislocation are due to factors such as inappropriate device size causing insufficient distraction or height restoration, suboptimal device positioning or spinous process anatomical variations.
A comprehensive summary of clinical outcomes and complications of observational studies for different IPD brands can be found in Tables 4,5 respectively.
Table 4
Article | Year | Study design | No of patients | Mean age/years | Follow-up/years | ODI | VAS (Back) | VAS (Leg) | ZCQ (symptom severity) | ZCQ (physical function) | ZCQ (patient satisfaction | SF-36 MCS | SF-36 PCS | |||||||||||||||||||||||
---|---|---|---|---|---|---|---|---|---|---|---|---|---|---|---|---|---|---|---|---|---|---|---|---|---|---|---|---|---|---|---|---|---|---|---|---|
Pre-op | Final FU | P value | Pre-op | Final FU | P value | Pre-op | Final FU | P value | Pre-op | Final FU | P value | Pre-op | Final FU | P value | Pre-op | Final FU | P value | Pre-op | Final FU | P value | Pre-op | Final FU | P value | |||||||||||||
Aperius | ||||||||||||||||||||||||||||||||||||
Beyer | 2015 | Prospective | 12 | 64.3 | 2 | 45.7 | 50.2 | >0.05 | 6 | 6.2 | >0.05 | 6 | 7.4 | >0.05 | – | – | – | – | – | – | – | – | – | 37.4 | 42.4 | >0.05 | 31.2 | 39.3 | >0.05 | |||||||
Marcia | 2015 | Prospective | 80 | 70.4 | 3 | 23.3 | 12.5 | <0.01 | 8.1 | 5.2 | <0.01 | – | – | – | – | – | – | – | – | – | – | – | – | – | – | – | – | – | – | |||||||
Masala | 2016 | Retrospective | 24 | 61 | 1 | 26.1 | 16.1 | 0.0075 | 8.3 | 5.1 | 0.0075 | – | – | – | – | – | – | – | – | – | – | – | – | – | – | – | – | – | – | |||||||
Menchetti | 2011 | Retrospective | 70 | 63.5 | 0.5 | – | – | – | 8.2 | 3.6 | – | – | – | – | – | – | – | – | – | – | – | – | – | – | – | – | – | – | – | |||||||
Van Meirhaeghe | 2012 | Prospective | 156 | 64.8 | 1 | – | – | – | 6.2 | N.S | <0.001 | 5.9 | N.S | <0.01 | 3 | 2.2 | <0.001 | 2.5 | 1.9 | <0.001 | – | – | – | – | – | – | – | – | – | |||||||
Meyer | 2018 | Prospective | 68 | 65 | 1 | – | – | – | 4 | 2.2 | 0.045 | 7.9 | 2.2 | 0.01 | 3.3 | 1.9 | <0.001 | 2.6 | 1.5 | <0.001 | – | – | – | – | – | – | – | – | – | |||||||
Surace | 2012 | Prospective | 37 | 64.3 | 1.5 | N.S | N.S | <0.001 | 7 | 2 | <0.001 | – | – | – | N.S | N.S | <0.001 | N.S | N.S | <0.001 | N.S | N.S | <0.001 | – | – | – | – | – | – | |||||||
Coflex | ||||||||||||||||||||||||||||||||||||
Errico | 2009 | Prospective | 127 | 54.8 | 6.3 | – | – | – | 6.4 | 1.8 | 0.002 | 6.5 | 0 | <0.001 | – | – | – | – | – | – | – | – | – | – | – | – | – | – | – | |||||||
Kong | 2007 | Retrospective | 18 | 61.7 | 1 | N.S | N.S | <0.05 | N.S | N.S | <0.05 | N.S | N.S | <0.05 | – | – | – | – | – | – | – | – | – | – | – | – | – | – | – | |||||||
Moojen | 2013 | Prospective | 80 | 66 | 1 | – | – | – | 6 | 2.3 | – | 5.2 | 2.3 | – | 3.1 | – | – | 2.6 | – | – | – | – | – | – | – | – | – | – | – | |||||||
Park | 2009 | Retrospective | 61 | 66.2 | 3.33 | 23 | 11.3 | <0.001 | 4.7 | 2.4 | <0.001 | 6.9 | 2.3 | <0.001 | – | – | – | – | – | – | – | – | – | – | – | – | – | – | – | |||||||
In-Space | ||||||||||||||||||||||||||||||||||||
Kantelhardt | 2010 | Retrospective | 87 | 71.8 | 1 | – | – | – | – | – | – | – | – | – | – | – | – | – | – | – | – | – | – | – | – | – | – | – | – | |||||||
Superion | ||||||||||||||||||||||||||||||||||||
Bini | 2011 | Prospective | 121 | 57.9 | 1 | 60 | 21 | <0.001 | 6.9 | 3.4 | <0.001 | 6.6 | 2.8 | <0.001 | – | – | – | – | – | – | – | – | – | N.S | N.S | <0.001 | N.S | N.S | <0.001 | |||||||
Miller | 2012 | Prospective | 80 | 67 | 0.5 | 38 | – | – | 5.5 | 2.2 | <0.001 | 6.1 | 1.8 | <0.001 | N.S | N.S | <0.001 | N.S | N.S | <0.001 | N.S | N.S | <0.001 | – | – | – | – | – | – | |||||||
Nunley | 2017 | Prospective | 88 | – | 5 | N.S | N.S | <0.001 | N.S | N.S | <0.001 | – | – | – | N.S | N.S | <0.001 | N.S | N.S | <0.001 | N.S | N.S | <0.001 | – | – | – | – | – | – | |||||||
Wallis | ||||||||||||||||||||||||||||||||||||
Daentzer | 2016 | Prospective | 10 | 64.4 | 2 | 40 | 17.3 | 0.017 | 6 | 2.7 | 0.042 | 4.7 | 2.1 | 0.06 | – | – | – | – | – | – | – | – | – | – | – | – | – | – | – | |||||||
X-Stop | ||||||||||||||||||||||||||||||||||||
Brussee | 2008 | Prospective | 65 | 64.4 | 1 | N.S | N.S | <0.001 | N.S | N.S | <0.001 | |||||||||||||||||||||||||
Hartjen | 2016 | Prospective | 42 | 69.8 | 2 | – | – | – | – | – | – | – | – | – | N.S | N.S | <0.001 | N.S | N.S | <0.001 | – | – | – | 49.1 | 52.6 | 0.072 | 28.1 | 37.6 | <0.001 | |||||||
Hsu | 2006 | Prospective | 100 | 70 | 2 | – | – | – | – | – | – | – | – | – | – | – | – | – | – | – | – | – | – | 51.5 | 54.3 | 0.309 | 27.8 | 38.4 | <0.001 | |||||||
Kuchta | 2009 | Retrospective | 175 | 69.4 | 2 | 32.6 | 20.3 | <0.001 | – | – | – | 61 | 39 | <0.001 | – | – | – | – | – | – | – | – | – | – | – | – | – | – | – | |||||||
Miller | 2012 | Prospective | 86 | 67 | 0.5 | 40 | – | – | 5.4 | 3.2 | <0.001 | 6.4 | 2.2 | <0.001 | N.S | N.S | <0.001 | N.S | N.S | <0.001 | N.S | N.S | <0.001 | – | – | – | – | – | – | |||||||
Siddiqui | 2007 | Prospective | 40 | 71.5* | 1 | 48 | 37 | – | – | – | – | – | – | – | 3.37 | 2.83 | - | 2.45 | 2.19 | – | – | 2.12 | – | – | – | – | – | – | – | |||||||
Staats | 2018 | Prospective | 99 | 75.6 | 2 | 53 | 30.3 | <0.001 | 7.7 | 4.1 | <0.001 | – | – | – | 3.5 | 2.5 | <0.001 | 2.9 | 2.1 | <0.001 | – | – | – | – | – | – | – | – | – | |||||||
Zucherman | 2005 | Prospective | 100 | 70 | 2 | – | – | – | – | – | – | – | – | – | – | – | – | – | – | – | – | – | – | – | – | – | – | – | – |
ODI, Oswestry disability scale; VAS, visual analogue scale; ZCQ, Zurich claudication questionnaire; SF-36, 36-Item short form survey; Pre-op, preoperation, Final FU, final follow-up; N.S., not specified.
Table 5
Article | Year | Study design | No of patients | Mean age/years | Follow-up/ |
Complication | ||||||
---|---|---|---|---|---|---|---|---|---|---|---|---|
Infection | Wound issues | Pain | Fracture | Explantation | Reoperation | Dislocation | ||||||
Aperius | ||||||||||||
Beyer | 2015 | Prospective | 12 | 64.3 | 2 | – | – | – | 5 | 5 | 0 | |
Marcia | 2015 | Prospective | 80 | 70.4 | 3 | 0 | 0 | 0 | 0 | 1 | 1 | – |
Masala | 2016 | Retrospective | 24 | 61 | 1 | – | – | – | – | – | – | – |
Menchetti | 2011 | Retrospective | 70 | 63.5 | 0.5 | 0 | 0 | 0 | 0 | 0 | 0 | 0 |
Van Meirhaeghe | 2012 | Prospective | 156 | 64.8 | 1 | 0 | 0 | 7 | 2 | – | – | – |
Meyer | 2018 | Prospective | 68 | 65 | 1 | 0 | 0 | 12 | 2 | – | 14 | 0 |
Surace | 2012 | Prospective | 37 | 64.3 | 1.5 | – | – | 2 | 1 | 2 | – | – |
Coflex | ||||||||||||
Errico | 2009 | Prospective | 127 | 54.8 | 6.3 | 0 | 0 | 0 | 0 | 2 | 2 | – |
Kong | 2007 | Retrospective | 18 | 61.7 | 1 | – | – | – | – | – | – | – |
Moojen | 2013 | Prospective | 80 | 66 | 1 | – | – | 1 | 3 | – | 9 | – |
Park | 2009 | Retrospective | 61 | 66.2 | 3.33 | – | – | 1 | – | – | 1 | – |
In-Space | ||||||||||||
Kantelhardt | 2010 | Retrospective | 87 | 71.8 | 1 | – | – | – | – | – | 10 | – |
Superion | ||||||||||||
Bini | 2011 | Prospective | 121 | 57.9 | 1 | 1 | 5 | 2 | – | 4 | 4 | – |
Miller | 2012 | Prospective | 80 | 67 | 0.5 | 0 | 0 | 2 | 1 | 4 | 6 | 0 |
Nunley | 2017 | Prospective | 88 | – | 5 | 5 | – | – | – | – | – | – |
Wallis | ||||||||||||
Daentzer | 2016 | Prospective | 10 | 64.4 | 2 | 0 | 1 | 0 | – | 1 | 1 | – |
X-Stop | ||||||||||||
Brussee | 2008 | Prospective | 65 | 64.4 | 1 | 0 | 0 | 0 | 0 | 0 | 0 | 0 |
Hartjen | 2016 | Prospective | 42 | 69.8 | 2 | – | – | 3 | 1 | – | – | 1 |
Hsu | 2006 | Prospective | 100 | 70 | 2 | – | – | – | – | – | – | – |
Kuchta | 2009 | Retrospective | 175 | 69.4 | 2 | – | – | – | – | – | – | – |
Miller | 2012 | Prospective | 86 | 67 | 0.5 | 1 | 1 | 1 | 3 | 2 | – | 1 |
Siddiqui | 2007 | Prospective | 40 | 71.5* | 1 | – | – | 7 | – | 2 | 4 | 2 |
Staats | 2018 | Prospective | 99 | 75.6 | 2 | 0 | 0 | 0 | 0 | 0 | 0 | 0 |
Zucherman | 2005 | Prospective | 100 | 70 | 2 | – | 4 | 6 | 1 | 1 | – | 1 |
Interspinous distraction devices
Aperius
Introduced in 2007, Aperius is a first-generation minimally invasive titanium IDD implanted between spinous process of affected spinal level under local anaesthetic. Its mechanism of decompression is via interspinous process distraction, without the need to remove interspinous ligament (11).
Five prospective and two retrospective studies (28,29,41-45) reporting on Aperius were identified. Mean age ranged from 61 to 70.4 years, and follow-up ranging from 0.5 to 3 years. Six of seven studies (28,29,42-45) showed significant clinical outcomes improvements between final follow-up and pre-operation. Only Beyer (41) reported non-significant changes to ODI, VAS (back and leg) and SF-36 scores. Meyer (44) reported highest re-operation rate of 20.6%.
In-Space
In-Space is a minimally invasive second-generation H-shaped IDD that has a central spacer with superior and inferior metal wings, designed to prevent ventral and lateral migration. Its implantation can be done via a posterior or lateral approach with no requirement of stripping paraspinal muscles (14).
One retrospective study (46) was identified involving 87 patients with mean age of 71.8 years and follow-up of 1 year.
Superion
Superion is a second-generation minimally invasive titanium IDD consisting of a single component with deployable superior and inferior projections that engage the spinous processes to secure its position. It is implanted via a cannula inserted between spinous processes (15).
Three prospective studies (47-49) reporting on Superion were identified. Mean age ranged from 57.9 to 67 years, while follow-up ranged from 0.5 to 5 years. There were unanimous significant improvements in clinical outcomes between final follow-up and pre-operation (47-49). Nunley (48) reported highest infection rates at 5.7% after the longest follow-up period of 5 years.
X-Stop
The X-Stop is a first-generation titanium alloy IDD consisting of a spacer and a wing assembly. The spacer is comprised of a tissue expander and oval spacer, while the wing is comprised of an adjustable wing and locking screw (17).
Seven prospective (49-55) and one retrospective study (56) were identified reporting on X-Stop. Mean age ranged from 64.4 to 75.6 years, while follow-up ranged between 0.5 and 2 years. There were unanimous significant improvements in clinical outcomes between final follow-up and pre-operation.
Interspinous stabilizers (ISS)
Coflex
Coflex ISS is a second-generation interlaminar functionally dynamic implant designed to stabilize instrumented levels. It consists of a single titanium alloy U-shaped component that is positioned horizontally with its apex oriented anteriorly. A set of wings extend vertically from superior and inferior arms of the ‘U’, designed to stabilize the implant to adjacent spinous processes. Spacing of superior and inferior wings are staggered to allow consecutive spinal level implantation. Bone-facing surfaces are ridged to reduce migration risks (12).
Two prospective (57,58) and two retrospective studies (20,21) were identified reporting on Coflex. Mean age ranged from 54.8 to 66.2 years, while follow-up ranged from 1 to 6.3 years. There were unanimous significant improvements in clinical outcomes between final follow-up and pre-operation. Moojen (58) reported highest fracture rates at 3.75%.
DIAM
DIAM is a first-generation minimally invasive H-shaped silicone device with woven polyester cables and titanium crimps for additional fixation. DIAM boasts to be a motion preserving device (7).
No study investigating DIAM in the treatment of LCS was found. Patient cohorts were either heterogeneous or omitted LCS as an indication. No further comments could be made.
Wallis
The Wallis is a second-generation device consisting of a polyetheretherketone (PEEK) block that is held in place by a flat Dacron cord or ribbon wrapped about adjacent spinous processes, with side notches to fit the physiological shape of the spine. PEEK has more elasticity to preserve some degree of motion when compared to more rigid titanium first-generation devices (10).
One retrospective study (59) was identified; involving 10 patients with mean age 64.4 years and follow-up of 2 years. Daentzer (59) reported significant improvements in ODI, VAS (back), SF-36 (pain, physical function, social function, emotional health and mental health components) when comparing final follow-up and pre-operation. VAS (leg) did not show significant improvement (P=0.06).
Comparing IPD and other management
Quantitative analysis was performed to consolidate comparative results between IPD and open decompression as well as ISS and interbody fusion (IF), in the form of forest plots. Odds ratio (OR) and 95% confidence interval (CI) were used as primary summary statistics for dichotomous variables, while mean difference (MD) and 95% CI were used for continuous variables. Analysis was performed with inverse variance statistical method and random-effects model to account for variation across studies. I2 statistic estimates degree of variation owing to heterogeneity rather than chance, with I2>50% regarded as substantial heterogeneity. P-value of <0.05 represent statistical significance. Due to the heterogeneity of clinical outcome parameters across studies, quantitative analyses could not be performed.
IPD vs. non-operative management
IPD were noted to be significantly more effective than non-operative therapy in improving quality of life and clinical outcomes for patients suffering from LCS. However, limited studies have reported such comparisons, probably due to an earlier systematic review highlighting the futility of continuing conservative management in symptomatic LCS after failed trial of 3 to 6 months (60). Hence, performing randomized trials to compare IPD and conservative management may infringe on ethical values such as prolonged suffering for patients who have failed conservative management.
Two multi-centered randomized controlled trials (RCT) (52,55) with 2-year follow-up were performed to compare X-Stop IDD and non-operative treatment in symptomatic LCS patients. Both studies reported that patient receiving X-Stop IDD had an overall statistically significant improved SF-36 score from pre-operative values and against non-operative patient. Zucherman (55) reported that X-Stop resulted in significant improvements of ZCQ score, with more patients indicating satisfaction from symptom relief.
Mini open IDD vs. open decompression
Comparing mini open IDD and open decompression revealed that IDD had similar or worse outcomes than open decompression, and was associated with higher complications and reoperations risk. Hence, benefits and risks of mini open IDD have to be weighed out for individual patients, making it unlikely to be a universally effective substitute for neural decompression in symptomatic LCS.
Beyer (41) prospectively compared 13 Aperius IDD and 33 bilateral open microsurgical decompression and revealed no statistically significant improvements in VAS back and leg scores, ODI and Short-form 36 scores for Aperius IDD, unlike the decompression group.
Postacchini (61) prospectively compared 36 Aperius IDD and 35 open decompression patients and reported lower rates of good results in Aperius IDD than open decompression (47% vs. 80%), and was consistent within the moderate (60% vs. 69%) or severe stenosis (31% vs. 80%) subgroups. Aperius IDD had higher rates of poor results (53% vs. 20%).
Sobottke (62) prospectively compared 11 Aperius IDD and 25 open decompression patients and reported no significant difference in ODI, SF-36 and walking tolerance up to 1-year follow-up between both groups, with Aperius IDD having an eventual worsening of VAS leg pain score.
Lønne (63) compared 40 X-Stop IDD and 41 minimally invasive decompression patients in an RCT. No statistically significant difference in ZCQ, ODI, EuroQol 5-dimensional questionnaire and NRS11 scores were reported up to 2-years follow-up.
Strömqvist (64) similarly compared 50 X-Stop IDD and 50 open decompression patients in an RCT and found no statistically significant difference between both groups in terms of ZCQ, SF-36 and VAS scores.
Quantitative analysis showed a higher reoperation rate (OR =3.96, 95% CI: 1.88–8.35, P<0.001) and all complications (OR =1.86, 95% CI: 1.06–3.27, P=0.03) associated with IDD than decompression (Figures 4,5 respectively).
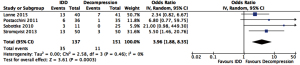
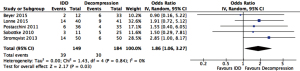
ISS versus open decompression
Zhong (65) retrospectively compared 46 Coflex ISS and 37 open decompression cases indicated for LCS with grade 1 spondylolisthesis and found higher 90-day complications, mean estimated blood loss, longer operative time and length of stay in Coflex ISS. However, at 1.7-year follow-up, overall revision and neurologic complications were similar.
ISS vs. interbody fusion surgery
For up-to-date clinical relevance, evidence comparing between ISS and IF presented were mainly done with Coflex. There were comparable clinical outcomes between both modalities, suggesting safety and efficacy as reasonable alternatives. Furthermore, ISS boast advantages including reduced operative time, blood loss, length of stay and adjacent segment mobility (66).
Kong (20) prospectively compared 18 Coflex ISS and 24 IF patients. No significant difference in ODI, VAS back and leg pain scores were reported between both groups. There was a statistically significant increase in upper adjacent segment ROM (P<0.05) in IF than Coflex ISS. No complications were reported in both groups up to 1-year follow-up.
Park (21) retrospectively compared 30 Coflex ISS and 31 IF patients at 2-year follow-up and found equivocal improvements in ODI, VAS back and leg pain scores and complications in both groups.
Davis (67) compared 215 Coflex ISS and 107 IF symptomatic LCS patients in an RCT and found significantly shorter length of stay, greater improvements in SF-12 physical health outcomes and ZCQ scores with Coflex. There were equivocal improvements in ODI, VAS back and leg pain scores, mental health outcomes and complications. IF was found to increase angulation at superior adjacent levels, accelerating degenerative changes at affected levels.
Quantitative analysis showed a lower mean blood loss (MD =421 mL, 95% CI: 48–795 mL, P=0.03) and shorter mean operative time (MD =93 min, 95% CI: 17–169 min, P=0.02) associated with ISS than IF (Figures 6,7 respectively). Forest plot comparing reoperation rate showed no statistically significant difference (OR=1.32, 95% CI: 0.60–2.93, P=0.49) (Figure 8).



The future
There are still complications associated with current IPD designs despite minimally invasive techniques and shorter operation time. Future developments of ISS involve the exploration of new composite materials to enhance its performance to achieve dynamic decompression, where ISS is rigid enough to provide sufficient distraction and disc height restoration, while still flexible enough to preserve some ROM. The use of higher durability materials may also reduce radiological changes over time, allowing for longer implant longevity.
Another area of development involves posterior spinal element reinforcement using cement augmentation (spinoplasty) (Figures 9,10). Bonaldi investigated the utility of spinoplasty by comparing 19 spinoplasty and 16 non-spinoplasty high risk fragility-fracture patients. He reported significantly reduced symptomatic delayed spinous process fracture with spinoplasty (0% vs. 25%, P=0.035) (68).
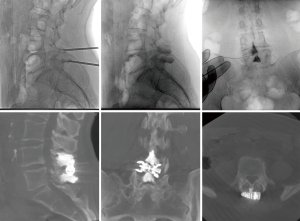
Manfré (69) explored spinoplasty as prophylaxis against bone remodelling or spinous process fracture related failures. Out of 174 patients, no restenosis was reported at 1-year follow-up, while 15 patients received two ISS at the same level with spinoplasty and experienced symptom resolution and remained so at 1-year follow-up (69). Manfre (19) also conducted a 9-year retrospective study involving 688 patients. Manfre (19) found lower symptom recurrence from complications with spinoplasty (<1% vs. 11.3%), suggesting utility in reducing reoperation rates eventually (19).
Another area of development is the approval for use of the minimally invasive, interspinous-interlaminar fusion device (Minuteman, Spinal Simplicity, USA) (70). This supplemental fixation device provides fixation and stabilisation of spinal segments with the aim of bony fusion. The Minuteman is designed to attach to the spinous processes of non-cervical spine with its bilateral locking plates and bone graft material placed within the device. Its minimally invasive approach reduces significant soft tissue dissection and eliminates the need for neural monitoring. Whilst clinical results of this device have not been published in the literature, this is a device that may become an alternative to traditional pedicle screw fixation constructs.
Limitations
There are limitations to this study. There were heterogeneous indications and patient populations in studies comparing between ISS and IF, despite ISS mainly indicated for LCS with stable spondylolisthesis. Hence, mismatch of implant type and patient population may potentially confound and dilutes the real outcomes. Stricter inclusion criteria with appropriate implant options are needed in future studies.
Open decompression techniques also varied widely amongst studies. The paucity of literature in comparing different techniques meant that clinical outcomes may be influenced by the quality of decompression in different technique, thus introducing bias.
Most studies included mainly investigated short- and medium-term outcomes. Possible explanations include the relatively novel nature of IPD and the high early reoperation rate associated with IDD, resulting in IDD to quickly lose favour. Though ISS did not have similar issues, the paucity of long-term data is inadequate to properly characterize outcome and compare with other interventions. Thus, it is imperative to obtain long-term data on IPD efficacy.
Our result when comparing between IDD and open decompression is limited to mini open implantation techniques and not complete percutaneous approaches. Complete percutaneous studies have shown encouraging outcomes in NC patients primarily caused by LSS, but this was not the case in other indications. The paucity of data comparing complete percutaneous IDD and open decompression with or without SF meant that further high quality RCTs are needed in this topic.
Lastly, while most studies discussed have a RCT study design, there are other studies that were comparative non-randomized studies. This meant that selection and recall bias cannot be completely excluded.
Conclusions
IPD provides a minimally invasive approach to treat symptomatic LCS. Mini open IDD had improved outcomes over non-operative treatment in mild-moderate LCS at 2-year follow-up, but had similar patient reported outcomes with a higher risk of re-operations than open decompression. ISS with open decompression may be a suitable alternative to decompression and IF for patients with stable grade 1 spondylolisthesis and central stenosis. To further characterize this procedure, future studies should focus on examining enhanced new generation IPD devices, complete percutaneous approaches, longer-term follow-up and careful patient selection.
Acknowledgments
This work was primarily performed in Monash Medical Centre, Victoria, Australia.
Funding: None.
Footnote
Reporting Checklist: The authors have completed the Narrative Review reporting checklist. Available at https://dx.doi.org/10.21037/jss-21-57
Peer Review File: Available at https://dx.doi.org/10.21037/jss-21-57
Conflicts of Interest: All authors have completed the ICMJE uniform disclosure form (available at https://dx.doi.org/10.21037/jss-21-57). KP serves as the Co-Editor-in-Chief of Journal of Spine Surgery. LM is the Chairperson of Diagnostic and Interventional Spine – European Society of Neuroradiology. LM serves on the European Radiology Scientific Editorial Board, and is the Director of Minimal Invasive Spine Therapy department – Mediterranean Institute for Oncology I.O.M, Catania – Italy. JAH reports grant from the Neiman health Policy Institute, and he is a consultant for Medtronic and persic. The other authors have no conflicts of interest to declare.
Ethical Statement: The authors are accountable for all aspects of the work in ensuring that questions related to the accuracy or integrity of any part of the work are appropriately investigated and resolved.
Open Access Statement: This is an Open Access article distributed in accordance with the Creative Commons Attribution-NonCommercial-NoDerivs 4.0 International License (CC BY-NC-ND 4.0), which permits the non-commercial replication and distribution of the article with the strict proviso that no changes or edits are made and the original work is properly cited (including links to both the formal publication through the relevant DOI and the license). See: https://creativecommons.org/licenses/by-nc-nd/4.0/.
References
- Schroeder GD, Kurd MF, Vaccaro AR. Lumbar Spinal Stenosis: How Is It Classified? J Am Acad Orthop Surg 2016;24:843-52. [Crossref] [PubMed]
- Lurie J, Tomkins-Lane C. Management of lumbar spinal stenosis. BMJ 2016;352:h6234. [Crossref] [PubMed]
- Machado GC, Ferreira PH, Yoo RI, et al. Surgical options for lumbar spinal stenosis. Cochrane Database Syst Rev 2016;11:CD012421 [PubMed]
- Richards JC, Majumdar S, Lindsey DP, et al. The treatment mechanism of an interspinous process implant for lumbar neurogenic intermittent claudication. Spine (Phila Pa 1976) 2005;30:744-9. [Crossref] [PubMed]
- Medtronic. APERIUS PercLID System. A percutaneous therapy for the treatment of degenerative lumbar spinal stenosis. 2008:1-12.
- Paradigm. Coflex Interlaminar stabilisation. 2010:1-35.
- Synthes. In-Space. Percutaneous interspinous distraction. Technique Guide. 2007:1-28.
- Medtronic. DIAM Spinal Stabilization System. Surgical Technique. 2005:1-16.
- Vertiflex. Superion Indirect Decompression System. Surgical Technique Manual. 2018:1-24.
- Zimmer. UniWallis Poserior Dyanmic Stabilization System. Surgical Technique. 2010:1-17.
- St Francis Medical Technology. XSTOP Interspinous Process Decompression System: Summary of Safety and Effectiveness. 2005:1-19.
- Nadeau M, Rosas-Arellano MP, Gurr KR, et al. The reliability of differentiating neurogenic claudication from vascular claudication based on symptomatic presentation. Can J Surg 2013;56:372-7. [Crossref] [PubMed]
- Chung SS, Lee CS, Kim SH, et al. Effect of low back posture on the morphology of the spinal canal. Skeletal Radiol 2000;29:217-23. [Crossref] [PubMed]
- Inufusa A, An HS, Lim TH, et al. Anatomic changes of the spinal canal and intervertebral foramen associated with flexion-extension movement. Spine (Phila Pa 1976) 1996;21:2412-20. [Crossref] [PubMed]
- Siddiqui M, Karadimas E, Nicol M, et al. Influence of X Stop on neural foramina and spinal canal area in spinal stenosis. Spine (Phila Pa 1976) 2006;31:2958-62. [Crossref] [PubMed]
- Wan Z, Wang S, Kozánek M, et al. Biomechanical evaluation of the X-Stop device for surgical treatment of lumbar spinal stenosis. J Spinal Disord Tech 2012;25:374-8. [Crossref] [PubMed]
- Bohm PE, Anderson KK, Friis EA, et al. Grade 1 spondylolisthesis and interspinous device placement: removal in six patients and analysis of current data. Surg Neurol Int 2015;6:54. [Crossref] [PubMed]
- Siewe J, Selbeck M, Koy T, et al. Indications and contraindications: interspinous process decompression devices in lumbar spine surgery. J Neurol Surg A Cent Eur Neurosurg 2015;76:1-7. [PubMed]
- Manfre L, De Vivo AE, Al Qatami H, et al. Successful use of percutaneous interspinous spacers and adjunctive spinoplasty in a 9 year cohort of patients. J Neurointerv Surg 2020;12:673-7. [Crossref] [PubMed]
- Kong DS, Kim ES, Eoh W. One-year outcome evaluation after interspinous implantation for degenerative spinal stenosis with segmental instability. J Korean Med Sci 2007;22:330-5. [Crossref] [PubMed]
- Park SC, Yoon SH, Hong YP, et al. Minimum 2-year follow-up result of degenerative spinal stenosis treated with interspinous u (coflex). J Korean Neurosurg Soc 2009;46:292-9. [Crossref] [PubMed]
- Gazzeri R, Galarza M, Neroni M, et al. Failure rates and complications of interspinous process decompression devices: a European multicenter study. Neurosurg Focus 2015;39:E14 [Crossref] [PubMed]
- Kim DH, Shanti N, Tantorski ME, et al. Association between degenerative spondylolisthesis and spinous process fracture after interspinous process spacer surgery. Spine J 2012;12:466-72. [Crossref] [PubMed]
- Barbagallo GM, Olindo G, Corbino L, et al. Analysis of complications in patients treated with the X-Stop Interspinous Process Decompression System: proposal for a novel anatomic scoring system for patient selection and review of the literature. Neurosurgery 2009;65:111-19; discussion 119-20. [Crossref] [PubMed]
- Schizas C, Theumann N, Burn A, et al. Qualitative grading of severity of lumbar spinal stenosis based on the morphology of the dural sac on magnetic resonance images. Spine (Phila Pa 1976) 2010;35:1919-24. [Crossref] [PubMed]
- Akazawa T, Kotani T, Sakuma T, et al. MRI evaluation of dural sac enlargement by interspinous process spacers in patients with lumbar spinal stenosis: Does it play a role in the long term? J Orthop Sci 2019;24:979-84. [Crossref] [PubMed]
- Nandakumar A, Clark NA, Peehal JP, et al. The increase in dural sac area is maintained at 2 years after X-stop implantation for the treatment of spinal stenosis with no significant alteration in lumbar spine range of movement. Spine J 2010;10:762-8. [Crossref] [PubMed]
- Marcia S, Hirsch JA, Chandra RV, et al. Midterm Clinical and Radiologic Outcomes after Percutaneous Interspinous Spacer Treatment for Neurogenic Intermittent Claudication. J Vasc Interv Radiol 2015;26:1687-93.e1-2.
- Masala S, Marcia S, Taglieri A, et al. Degenerative lumbar spinal stenosis treatment with Aperius™ PerCLID™ system and Falena® interspinous spacers: 1-year follow-up of clinical outcome and quality of life. Interv Neuroradiol 2016;22:217-26. [Crossref] [PubMed]
- Hjaltadottir H, Hebelka H, Molinder C, et al. Axial loading during MRI reveals insufficient effect of percutaneous interspinous implants (Aperius™ PerCLID™) on spinal canal area. Eur Spine J 2020;29:122-8. [Crossref] [PubMed]
- Schizas C, Pralong E, Tzioupis C, et al. Interspinous distraction in lumbar spinal stenosis: a neurophysiological perspective. Spine (Phila Pa 1976) 2013;38:2113-7. [Crossref] [PubMed]
- Byun DH, Shin DA, Kim JM, et al. Finite element analysis of the biomechanical effect of coflex™ on the lumbar spine. Korean J Spine 2012;9:131-6. [Crossref] [PubMed]
- Chen HC, Wu JL, Huang SC, et al. Biomechanical evaluation of a novel pedicle screw-based interspinous spacer: A finite element analysis. Med Eng Phys 2017;46:27-32. [Crossref] [PubMed]
- Wan Z, Li G. X-Stop® Implantation Effectively Limits Segmental Lumbar Extension in-vivo without Altering the Kinematics of the Adjacent Levels. Turk Neurosurg 2015;25:279-84. [PubMed]
- Fairbank JC, Couper J, Davies JB, et al. The Oswestry low back pain disability questionnaire. Physiotherapy 1980;66:271-3. [PubMed]
- Roland M, Morris R. A study of the natural history of back pain. Part I: development of a reliable and sensitive measure of disability in low-back pain. Spine (Phila Pa 1976) 1983;8:141-4. [Crossref] [PubMed]
- Huskisson EC. Measurement of pain. Lancet 1974;2:1127-31. [Crossref] [PubMed]
- Stucki G, Daltroy L, Liang MH, et al. Measurement properties of a self-administered outcome measure in lumbar spinal stenosis. Spine (Phila Pa 1976) 1996;21:796-803. [Crossref] [PubMed]
- Phan K, Rao PJ, Ball JR, et al. Interspinous process spacers versus traditional decompression for lumbar spinal stenosis: systematic review and meta-analysis. J Spine Surg 2016;2:31-40. [Crossref] [PubMed]
- Kim DH, Tantorski M, Shaw J, et al. Occult spinous process fractures associated with interspinous process spacers. Spine (Phila Pa 1976) 2011;36:E1080-5. [Crossref] [PubMed]
- Beyer F, Yagdiran A, Neu P, et al. Percutaneous interspinous spacer versus open decompression: a 2-year follow-up of clinical outcome and quality of life. Eur Spine J 2013;22:2015-21. [Crossref] [PubMed]
- Menchetti PP, Postacchini F, Bini W, et al. Percutaneous surgical treatment in lumbar spinal stenosis with Aperius-PercLID: indications, surgical technique and results. Acta Neurochir Suppl 2011;108:183-6. [Crossref] [PubMed]
- Van Meirhaeghe J, Fransen P, Morelli D, et al. Clinical evaluation of the preliminary safety and effectiveness of a minimally invasive interspinous process device APERIUS(®) in degenerative lumbar spinal stenosis with symptomatic neurogenic intermittent claudication. Eur Spine J 2012;21:2565-72. [Crossref] [PubMed]
- Meyer B, Baranto A, Schils F, et al. Percutaneous Interspinous Spacer vs Decompression in Patients with Neurogenic Claudication: An Alternative in Selected Patients? Neurosurgery 2018;82:621-9. [Crossref] [PubMed]
- Surace MF, Fagetti A, Fozzato S, et al. Lumbar spinal stenosis treatment with Aperius perclid interspinous system. Eur Spine J 2012;21:S69-74. [Crossref] [PubMed]
- Kantelhardt SR, Török E, Gempt J, et al. Safety and efficacy of a new percutaneously implantable interspinous process device. Acta Neurochir (Wien) 2010;152:1961-7. [Crossref] [PubMed]
- Bini W, Miller LE, Block JE. Minimally invasive treatment of moderate lumbar spinal stenosis with the superion interspinous spacer. Open Orthop J 2011;5:361-7. [Crossref] [PubMed]
- Nunley PD, Patel VV, Orndorff DG, et al. Five-year durability of stand-alone interspinous process decompression for lumbar spinal stenosis. Clin Interv Aging 2017;12:1409-17. [Crossref] [PubMed]
- Miller LE, Block JE. Interspinous spacer implant in patients with lumbar spinal stenosis: preliminary results of a multicenter, randomized, controlled trial. Pain Res Treat 2012;2012:823509 [Crossref] [PubMed]
- Brussee P, Hauth J, Donk RD, et al. Self-rated evaluation of outcome of the implantation of interspinous process distraction (X-Stop) for neurogenic claudication. Eur Spine J 2008;17:200-3. [Crossref] [PubMed]
- Hartjen CA, Resnick DK, Hsu KY, et al. Two-Year Evaluation of the X-STOP Interspinous Spacer in Different Primary Patient Populations With Neurogenic Intermittent Claudication Because of Lumbar Spinal Stenosis. Clin Spine Surg 2016;29:305-11. [Crossref] [PubMed]
- Hsu KY, Zucherman JF, Hartjen CA, et al. Quality of life of lumbar stenosis-treated patients in whom the X STOP interspinous device was implanted. J Neurosurg Spine 2006;5:500-7. [Crossref] [PubMed]
- Siddiqui M, Smith FW, Wardlaw D. One-year results of X Stop interspinous implant for the treatment of lumbar spinal stenosis. Spine (Phila Pa 1976) 2007;32:1345-8. [Crossref] [PubMed]
- Staats PS, Chafin TB, Golovac S, et al. Long-Term Safety and Efficacy of Minimally Invasive Lumbar Decompression Procedure for the Treatment of Lumbar Spinal Stenosis With Neurogenic Claudication: 2-Year Results of MiDAS ENCORE. Reg Anesth Pain Med 2018;43:789-94. [Crossref] [PubMed]
- Zucherman JF, Hsu KY, Hartjen CA, et al. A multicenter, prospective, randomized trial evaluating the X STOP interspinous process decompression system for the treatment of neurogenic intermittent claudication: two-year follow-up results. Spine (Phila Pa 1976) 2005;30:1351-8. [Crossref] [PubMed]
- Kuchta J, Sobottke R, Eysel P, et al. Two-year results of interspinous spacer (X-Stop) implantation in 175 patients with neurologic intermittent claudication due to lumbar spinal stenosis. Eur Spine J 2009;18:823-9. [Crossref] [PubMed]
- Errico TJ, Kamerlink JR, Quirno M, et al. Survivorship of coflex Interlaminar-Interspinous Implant. SAS J 2009;3:59-67. [Crossref] [PubMed]
- Moojen WA, Arts MP, Jacobs WC, et al. Interspinous process device versus standard conventional surgical decompression for lumbar spinal stenosis: randomized controlled trial. BMJ 2013;347:f6415. [Crossref] [PubMed]
- Daentzer D, Hurschler C, Seehaus F, et al. Posterior dynamic stabilization in the lumbar spine - 24 months results of a prospective clinical and radiological study with an interspinous distraction device. BMC Musculoskelet Disord 2016;17:90. [Crossref] [PubMed]
- Kovacs FM, Urrútia G, Alarcón JD. Surgery versus conservative treatment for symptomatic lumbar spinal stenosis: a systematic review of randomized controlled trials. Spine (Phila Pa 1976) 2011;36:E1335-51. [Crossref] [PubMed]
- Postacchini R, Ferrari E, Cinotti G, et al. Aperius interspinous implant versus open surgical decompression in lumbar spinal stenosis. Spine J 2011;11:933-9. [Crossref] [PubMed]
- Sobottke R, Röllinghoff M, Siewe J, et al. Clinical outcomes and quality of life 1 year after open microsurgical decompression or implantation of an interspinous stand-alone spacer. Minim Invasive Neurosurg 2010;53:179-83. [Crossref] [PubMed]
- Lønne G, Johnsen LG, Rossvoll I, et al. Minimally invasive decompression versus x-stop in lumbar spinal stenosis: a randomized controlled multicenter study. Spine (Phila Pa 1976) 2015;40:77-85. [Crossref] [PubMed]
- Strömqvist BH, Berg S, Gerdhem P, et al. X-stop versus decompressive surgery for lumbar neurogenic intermittent claudication: randomized controlled trial with 2-year follow-up. Spine (Phila Pa 1976) 2013;38:1436-42. [Crossref] [PubMed]
- Zhong J, O'Connell B, Balouch E, et al. Patient Outcomes After Single-level Coflex Interspinous Implants Versus Single-level Laminectomy. Spine (Phila Pa 1976) 2021;46:893-900. [PubMed]
- Abdel Ghany W, Amer A, Saeed K, et al. Evaluation of Interspinous Spacer Outcomes in Degenerative Lumbar Canal Stenosis: Clinical Study. World Neurosurg 2016;95:556-564.e3. [Crossref] [PubMed]
- Davis RJ, Errico TJ, Bae H, et al. Decompression and Coflex interlaminar stabilization compared with decompression and instrumented spinal fusion for spinal stenosis and low-grade degenerative spondylolisthesis: two-year results from the prospective, randomized, multicenter, Food and Drug Administration Investigational Device Exemption trial. Spine (Phila Pa 1976) 2013;38:1529-39. [Crossref] [PubMed]
- Bonaldi G, Bertolini G, Marrocu A, et al. Posterior vertebral arch cement augmentation (spinoplasty) to prevent fracture of spinous processes after interspinous spacer implant. AJNR Am J Neuroradiol 2012;33:522-8. [Crossref] [PubMed]
- Manfré L. Posterior Arch Augmentation (Spinoplasty) before and after Single and Double Interspinous Spacer Introduction at the Same Level: Preventing and Treating the Failure? Interv Neuroradiol 2014;20:626-31. [Crossref] [PubMed]
- Spinal Simplicity. Minuteman G3R MIS Fusion Plate. Lateral MIS surgical Technique. Spinal Fusion simplified. 2017:1-20.