Negotiating for new technologies: guidelines for the procurement of assistive technologies in spinal surgery: a narrative review
Introduction
The availability of assistive technologies in spine surgery continues to evolve rapidly (1-4). The use of navigation and robotic technology has demonstrated accurate placement of pedicle screws (5-14), enhancing safety and reducing re-operation rates (15,16) whilst allowing surgical teams to reduce their radiation exposure.
There are limitations to computer assisted navigation. These systems make certain assumptions regarding the true position of patient anatomy relative to a patient anchored reference marker and an acquired image. In addition, these technologies project simulated images based on the perceived location of instruments in relation to the reference frame. Any failure of the surgeon or system to recognize an unintended change in these variables can result in inaccuracy. It is paramount that surgeons use visual anatomic landmarks, tactile feedback, and knowledge of the assumptions made by these technologies to identify and troubleshoot when found to be inaccurate.
With continued advancements in imaging and software, computer assisted navigation will become further integrated with instrumented spinal surgeries. Young spine surgeons are now routinely exposed to these technologies in training (4,17,18). These surgeons will be the force for widespread adoption of these technologies as hospitals and practices continue to evolve with this paradigm shift. This paper serves as a reference for surgeons and hospitals to help decide which technology to adopt whilst highlighting some key elements of negotiation typically required. We present the following article in accordance with the Narrative Review reporting checklist (available at https://jss.amegroups.com/article/view/10.21037/jss-21-107/rc).
Methods
The nature of peer review publications, which typically compare one or two systems within the technology type, has resulted in the absence of a summative reference that compares all available assistive technologies. A resource with this collective information can be extremely valuable in directing surgeons and administrators when considering the acquisition of these technologies (19).
This is a narrative review which (I) presents an up-to-date summary of the currently available assistive technologies in spinal surgery; (II) makes comment on the utility of imaging, navigation, and robotics; (III) makes recommendations for the utility of the platform based on hospital size and (IV) discuss factors involved in negotiating for the purchase of these new technologies. All prices are stated in US dollars.
This narrative review was conducted using the following search strategy (Table 1). PubMed was used as the sole database for the review. The search was conducted on August 1st, 2021. MeSH terms used were: robotics, technology, imaging, and spine. Free-text terms used were: computer-assisted and navigation. The time-frame of results was from 1990–2021. Article types reviewed included clinical trials, randomized controlled trials, reviews, systematic reviews, and meta-analysis. Non-English articles were excluded. Articles were selected collectively by the three primary authors. Consensus was obtained collectively after analysis of each article regarding whether to include in the review.
Table 1
Items | Specification |
---|---|
Date of search | August 1st, 2021 |
Databases and other sources searched | PubMed |
Search terms used | MeSH terms: |
Robotics | |
Technology | |
Imaging | |
Spine | |
Free-text terms: | |
Computer-assisted | |
Navigation | |
Timeframe | 1990–2021 |
Inclusion and exclusion criteria | Inclusion: |
Clinical Trial articles | |
Randomized Controlled Trial articles | |
Review articles | |
Systematic Review articles | |
Meta-analysis articles | |
Exclusion: | |
Non-English publications | |
Selection process | Articles were selected collectively by the three primary authors. Consensus was obtained collectively after review of each article |
Any additional considerations | None |
Discussion
Imaging [three-dimensional (3D) fluoroscopy and mobile computerized tomography (CT)]
3D fluoroscopy (Figure 1)
3D fluoroscopy scanners are cheaper, lighter, smaller, and more maneuverable than mobile CT scanners. Radiation generation is not a helpful differentiator between these technologies because 3D fluoroscopy radiation can exceed that of mobile CT. 3D scanners offer a lesser image quality but are faster than mobile CT. In addition, 3D fluoroscopy can be utilized as a 2D-flouroscopy unit providing additional utilization. The limitations of the smaller field of view (FOV) can be overcome by performing multiple image acquisitions if using for longer constructs, but this will reduce efficiency.
Second-generation 3D fluoroscopy scanners, the RFD 3D (Ziehm, Nuremberg, Germany), Cios Spin (Siemens AG, Erlangen, Germany), and the O-arm II (Medtronic Navigation, Louisville, CO, USA) have made substantial improvements in both image resolution and FOV compared with first-generation 3D scanners Orbic (Siemens), Vario 3D (Ziehm), and O-arm I (Medtronic), which are now obsolete. A new mobile 3D imaging device, the Loop-X (BrainLab, Feldkirchen, Germany) has just been approved for use in 2021.
The superior image resolution and wider FOV provide continued utility for the use of mobile CT in spine surgery. The benefit over second generation 3D fluoroscopy may be negligible for 1 or 2 level adult degenerative lumbar fusions, but more helpful in long construct/complex surgeries or in patients with a high BMI (20). Although the larger mobile CT scanner are technically maneuverable, specialist or integrated tables are required hence they usually cannot be moved in and out of ORs during a procedure unlike 3D systems which is a limitation. By contrast, the Ziehm RFD 3D and Cios SPIN are similar in size to standard 2D imaging systems and easily used across multiple theatres. The LoopX and O-arm II are larger but may be able to be utilised between theatres depending on setup.
There is a commercial imperative for companies that do not make navigation or robotics platforms to integrate an imaging system with as many of these platforms as possible. Conversely, a trend towards bundled integrated imaging, navigation and robotics systems may allow bundling of equipment and more favourable pricing. At this time, Siemens, Ziehm, and Samsung do not make navigation or robotics platforms for spine, whilst the Airo, O-arm II and Loop-X are all often supplied in conjunction with guidance systems.
Ziehm RFD 3D CMOS
As an imaging only company that is smaller than Samsung and Siemens, the Ziehm RFD 3D CMOS is a system with strong compatibility across navigation and robotics platforms. The 20 cm FOV means it will have utility with universal registration technologies, although their use may be challenging in larger BMI patients if the universal registration mechanism sits above the skin edge. The system is easily mobile between theatres and functions well as a standard 2D-fluoroscopy system. The Ziehm RFD image quality is similar to the O-arm II and Siemens CIOS, although being from a smaller company the user interface may be less familiar to some radiographers. It is approximately $350,000–400,000.
Siemens CIOS Spin
The second-generation Siemens Cios Spin replaces the Siemens Orbic. The Cios Spin provides a significant improvement in image quality, but is limited by a small FOV of 16 cm for 3D scans (despite having 30-cm detectors for 2D). This limits utility for navigation, robotics and complex long construct surgery. It resembles in size the standard 2D X-ray machines found in theatres and is highly mobile. Of all 3D systems, radiographers are most likely to be familiar with the Siemens interface as it is relatively standard across X-ray, CT and MRI. Cios costs $350,000–400,000 and currently offers automatic registration with both BrainLab navigation and NuVasive Pulse.
Medtronic O-arm II
The Medtronic O-arm II is the most commonly utilised imaging platform for spinal fusion worldwide. Image stacking allows two scans to be completed side by side, broadening the FOV in the transverse plane, meaning the whole pelvis can be imaged if required. The footprint of the O-arm is similar to other 3D scanners, although its overall size makes 2D imaging harder. Longer 48 cm 2D imaging allows for imaging of most long scoliosis constructs. The O-arm II has radiation doses similar to mobile CT scanners (2–2.5 mSv) (21). The O-arm II requires an assisted-drive mechanism due to its weight, with optional manual motion. The O-arm automatically registers with Stealth Navigation and Robotics and costs approximately $620,000–690,000.
BrainLab LoopX
The LoopX is a new platform designed to improve imaging workflow by providing 3D as well as 2D imaging using a novel motorised design. The appearance is more like mobile CT scanners and the 48 cm FOV is larger than other mobile 3D options. Positions can be programmed for a combination of 2D and 3D imaging with the scanner designed to move in and away from the operative field as required. The large 121 cm gantry provides flexibility with positioning. Improved 2D functionality still requires surgical teams to wear lead gowns: the avoidance of which has helped popularized using 3D imaging, navigation and robotics (22). Clinical experience is limited, although highest spatial resolution in its class means image quality should be similar to other 3D modalities. The LoopX will likely be optimised for use with BrainLab only. Price estimate is $570,000–590,000.
3D-fluoroscopy imaging systems are demonstrated in Figure 1.
Mobile CT (Figure 2)
Airo 32-slice mobile CT
The Mobius Airo (Stryker, Kalamazoo, MI. USA) has a 100-cm scan length, 56-cm FOV, and a 107-cm bore. The Airo requires and integrated table, which keeps the scanner close to the operative area and may help with scanning efficiency but restricts operating table types and options. Airo offers automatic registration with BrainLab navigation. Additionally, the Airo has the ability to used as a traditional diagnostic CT scanner when not being used in the operating room. Airo costs $1,100,000.
BodyTom 32-slice mobile CT
The Samsung BodyTom (Neurologica, Danvers, MA, USA) has the largest scan length (200 cm) and a similar FOV of (60 cm), but its 85-cm bore is narrower than the Airo and LoopX. In contrast to Airo’s integrated table, BodyTom uses a detached cantilevered carbon fibre table. No specific table type or brand is required. The BodyTom can be removed from OR after scanning, but due to its size it is not typically feasible to remove the scanner from the OR during surgery. BodyTom is well setup for general radiology use and functions as a standalone diagnostic CT scanner. It does not have automatic registration hence requires a universal registration device for use with navigation and robotics which is a limitation. The BodyTom costs $1,100,000.
Mobile CT imaging systems are demonstrated in Figure 2.
Mitigating radiation exposure
Spinal fusion patients are likely to be exposed to significant amounts of radiation (23). It is common for patients to receive a preoperative CT scan, intraoperative 3D fluoroscopy or mobile CT scan(s) and a postoperative CT scan to check instrumentation. These scans are common routine clinical practice, with patient radiation exposure justified by effective planning, safety enhancements of navigation and robotics, and post-operative assessment. The use of robotics and intraoperative 3D or CT minimises or eliminates exposure of the surgical team to radiation as they can be effectively shielded or leave the room (24-26).
Methods to reduce radiation exposure at each episode of care include: (I) surgeons requesting lower dose protocols for preoperative imaging; (II) intraoperatively it is possible to use lower dose settings on 3D or CT scanners; (III) systems that eliminate or reduce the need for the number of 3D fluoroscopy or CT scans per case. Examples of this include 7D Flash Navigation and BrainLab Region Match, or using mobile CT over 3D fluoroscopy scans when imaging of longer spinal segments is required; (IV) reviewing the need for post-instrumentation or post-operative CT if mobile CT or 3D is utilised during a spinal fusion and the same information would be provided.
Navigation (Figures 3,4)
Navigation systems are emerging requirement for spinal centres and should form the core of any assistive technology setup. As the systems mature, the software, hardware and associated instrumentation of major navigation platforms is increasingly standardised, making them harder to differentiate based on core features alone, whilst providing peace of mind from a purchasing perspective.
The most widely used navigation systems are Medtronic Stealth, BrainLab and Stryker Navigation. Novel systems are 7D Surgical, Augmedics XVision and NuVasive Pulse which offer some niche features not available in the existing platforms.
Medtronic Stealth S8
The Stealth system from Medtronic (Minneapolis, MN, USA) is the most widely used navigation system (27) and typically used with the O-arm. Like other navigation systems, Stealth provides the best integration with Medtronic implants. In 2017, Medtronic and the FDA issued warnings about using non-Medtronic instrumentation with NavLock instruments. Stealth provides the most comprehensive range of instruments for spinal fusion outside of pedicle screws, including interbody instruments and navigated high-speed burrs. A Stealth S8 costs approximately $365,000–505,000.
BrainLab
BrainLab (Munich, Germany) is an independent navigation company, not owned by a device manufacturer. BrainLab is unique in that it allows for use with various imaging modalities. Most spinal implant companies offer BrainLab compatible instrumentation. Surface matching is similar to traditional point-based fiducial type registration but allows surgeons to identify larger regions rather than specific points using a remote control on the navigation pointer. Automatic registration is available with the Airo CT, Loop X, Cios and Ziehm RFD. Limitations to BrainLab include a lack of an integrated navigated drill and fewer options for navigated instruments as compared to Stealth. BrainLab Curve Navigation is compatible with the Cirq robotic arm and costs $290,000–435,000.
Stryker
Stryker Navigation (Kalamazoo, MI, USA) has battery-powered instruments (rather than lighter reflective spheres) which gives the instruments a significantly different feel to other navigation platforms. SpineMask is a novel adhesive cutaneous frame that does not require invasive attachment to the spine. It has utility in percutaneous cases whilst avoiding morbidity with a non-invasive reference array may expand the use of navigation for surgical procedures involving decompression alone (28,29). Stryker Navigation is highly geared towards use with Stryker Implants and costs $215,000–350,000.
7D Surgical
7D Surgical (Toronto, Canada) uses Flash optical tracking registration to map and register patient anatomy using visible light only with no intraoperative radiation. Eliminating the need for 3D or CT imaging for registration and specialised carbon fibre tables may significantly reduce setup costs if these are not already available. The rapid registration can be repeated without the need for repeat image acquisition. Registration times have been shown to be faster compared with those of contemporary navigation systems (30). Rapid multiple registrations make 7D Surgical an ideal platform for long construct work. While this novel optical registration is limited to open cases with visualized anatomy, the platform can also integrate with 3D or CT imaging utilising standard registration mechanisms as required for MIS procedures. Price is $450,000–550,000.
Augmedics XVision
Augmedics XVision provides surgeons with augmented reality headsets. Transitioning to heads up display compared to the use of a monitor at the foot or head of the patient should bring ergonomic advantages permitting the surgeon to visualise the navigated instrument and spine anatomy simultaneously. This new platform provides basic spinal instrumentation for pedicle screw insertion and only offers universal registration (requiring 3D or CT imaging). In place of a large navigation system, Augmedics utilises a small computer that can be mounted on an intravenous pole saving significant space, with surgeon specific headsets providing personalised equipment for surgeons. The platform is significantly cheaper than contemporary navigation platforms at approximately $100,000–150,000.
NuVasive Pulse
NuVasive Pulse (San Diego, CA, USA) expands the utility of existing navigation platforms by integration of surgical assistance technologies already offered by NuVasive into a single system. The Pulse system physically resembles other navigation system but makes use of the required floor space for navigation by integrating neuromonitoring, rod contouring guide and surgical planning tools. Pulse includes a 2D X-ray navigation-like option, LessRay, which can reduce reliance on X-ray for procedures utilising standard fluoroscopy. Standard 3D based navigation features are combined with 2D imaging for interbody work. 3D integration is currently only available with Siemens CIOS Spin. The system is optimised for use with NuVasive instrumentation and costs $365,000–505,000.
Spinal navigation systems are demonstrated in Figures 3,4.
Robotics (Figure 5)
Current robotic platforms incorporate freehand navigation in addition to pre-planned robotic assisted trajectory alignment for pedicle screws and interbody device placement. The MazorX (Medtronic) and Excelcius GPS (Globus) combine navigation and robotics functionality into a single platform and offer comprehensive registration options with 2D, 3D and CT imaging.
Both platforms require pre-planning for screw trajectories, typically completed based on pre-operative CT to save operative time. The efficiency benefit of pre-operative screw planning is subjective and likely to be a surgeon specific preference. The combination of robotic arms with navigated instrumentation allows K-wireless percutaneous screws.
The smaller and cheaper Cirq acts more like a surgical assistant, saving a trajectory defined by navigation during the operation using a table mounted robotic arm that requires BrainLab’s Curve Navigation system.
MazorX
The MazorX Stealth Edition (Medtronic) combines the Mazor Robotics technology with Stealth Navigation into a single platform and offers automatic registration with the O-arm II. The MazorX is table-mounted with the robotic arm and MazorX planning functionality complemented by integration with navigated stealth instrumentation, including high speed burrs and interbody equipment. MazorX is utilized with Medtronic implants and costs $1,000,000–1,100,000.
Excelsius GPS
The Excelsius GPS robot (Globus Medical, Philadelphia, PA, USA) combines a robotic arm with navigated instrumentation. Software updates released in 2021 have provided increased robotic arm efficiency plus the ability to perform navigation-assisted interbody work. The system can be used with or without robotic functionality, with the comprehensive pre-planning options accessible for freehand navigation and a range of instrumentation comparable to other navigation platforms. The Excelsius GPS automatically registers with the recently announced Excelsius3D mobile 3D imaging platform and is optimised for Globus prostheses (31). It costs $1,000,000–1,100,000, without the associated 3D imaging platform.
BrainLab Cirq
Unlike the Excelsius GPS and MazorX, Cirq is a smaller robot and attaches to operative table rails. It is available in active drill guide alignment tool or cheaper passive retaining arm for the BrainLab navigation platform. The Cirq is a less obtrusive system than the larger robots, with a focus on providing additional stability for navigated procedures. The Cirq does not allow for tapping or screw placement through the end effector, only assists with trajectory guidance during drilling and placing a K-wire. As a simpler adjuvant offering than larger integrated robotics platforms, it is also cheaper and costs a $180,000 for the passive version and $360,000 for the active version. Like BrainLab navigation, Cirq is favourably implant agnostic.
Robotic platforms are demonstrated in Figure 5.
Guidelines
Guidelines for the acquisition of new assistive technologies for spinal fusion are provided in Table 2.
Table 2
1 | Open-platform navigation and robotics systems that provide surgeons with access to all software and hardware features regardless of implant choice are preferred |
2 | Imaging systems with maximum compatibility with navigation and robotics platforms are optimal |
3 | Navigation systems that offer a universal registration mechanism should become standard |
4 | 3D fluoroscopy provides the greatest benefit when speed, operating efficiency, and mobility are required |
5 | Mobile CT is more useful when imaging of long constructs, high BMI, or cervico-thoracic anatomy is needed |
6 | Radiation safety awareness that new 3D-fluoroscopy units can deliver radiation doses comparable to those of CT is needed |
7 | Robotic arm platforms may offer marketing advantages and be preferred by some surgeons over navigation |
8 | Hospitals should be aware of a trend towards navigation and robotics platforms primarily being compatible with the (single) implant manufacturing company |
3D, three-dimensional; CT, computerized tomography; BMI, body mass index.
Recommendations based on hospital size (Figure 6)
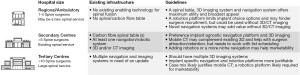
Appropriateness of these technologies may vary based on hospital size and the complexity of the spine surgery service line at individual facilities. Small centres with a small or developing spine service may lack ancillary equipment such as a carbon fibre table and 2D fluoroscopy equipment. In addition, the operating facilities may not be sufficiently sized for use, transport, and storage of larger equipment. For these institutions, devices with maximal utility and ease of use are important. A 3D fluoroscopy imaging system (that allows for 2-D fluoroscopy acquisition) combined with a navigation system would be a cost-effective and intuitive transition to navigation.
Secondary centres with pre-existing spine surgery service lines will likely possess some existing infrastructure such as carbon fibre tables, 2-D imaging systems, and possibly older generation navigation equipment. These institutions may benefit from 3D fluoroscopy imaging and navigation systems with agnostic implant integration to accommodate varying surgeon preference. Mobile CT may complement already existing latest generation imaging systems. Robotics may provide marketability advantages.
Large tertiary centres will already have extensive capital including several imaging and navigation platforms. These systems will be more amenable to investing in more cutting-edge technologies with lower utilization rates. A robotics platform is likely to be required at these facilities for marketability and surgeon recruitment. Robotics could provide significant advantages in these settings with ability to perform complex single position surgeries made more difficult with traditional navigation (32,33).
Regardless of institution size, the most important aspect is surgeon preference and commitment to the adoption of the technology. Surgeons prioritise workflow efficiency. These technologies require a learning-curve and surgeons need to be involved with the purchase decision to increase their willingness to invest this time upfront. Each platform has a slightly different workflow which may not be universally congruent amongst surgeons.
Guidelines for adopting new technology based on hospital size are summarized in Figure 6.
Negotiating
Spinal implant companies continue to expand into the navigation and robotics market meaning fewer implant agnostic options for hospitals. Implant companies leverage these large capital purchases with implant contracts to maximize revenue generation potential (34,35). BrainLab and Augmedics are the only platforms not manufactured by spinal implant companies. Surgeons’ preferences for implant systems will heavily impact the decision making around navigation and robotic platforms. This is due to a more seamless integration of some implants with systems owned by the device companies. Larger centres are likely to require multiple systems to satisfy larger surgeon groups who use a range of implant suppliers. Where a navigation or robotics system is optimised for use with a particular implant supplier, there should be cost savings for the hospital through commercial arrangements with the implant vendor to supply enabling technology at a reduced cost.
These new technologies are large capital expenditures that will undergo significant analysis by hospital management before purchasing. It is important to understand the perspectives of the hospitals to effectively approach these discussions.
Historically, financials have dominated hospital administration decisions for capital purchases (36). Simply advocating that an expensive piece of equipment is better for patient care does not always lead to acquiring the latest technologies. Quality care continues to be intricately linked with financials with the rise of value-based healthcare and capitated payment models (37,38). Quality will continue to be a sustainable business strategy as the adoption of alternative payment methods gains emphasis.
Physician preference items (PPI) such as spinal implants can account for 40–60% of total supply costs (18). Surgeons need to be at the forefront of the decision to invest in these technologies and understand their leverage. Surgeons maintain a unique position in the health care infrastructure where their approach to care has significant ramifications on both the quality and cost of care. Computer-assisted technologies are no longer simply adjuncts to spinal care. In the coming decades, we could see them become as essential to the performance of spinal procedures as the instruments themselves.
These new technologies can assist surgeons adopt more minimally invasive techniques that can allow for faster recovery times. Minimally invasive surgery can translate into reduced rate of surgical site infection (SSI) (39), a commonly tracked quality metric. In some health care systems, faster recovery and reduced length of stay (40) can significantly reduce the costs of care.
In addition, navigation and robotics can help increase the accuracy of instrumentation placement and confirm placement of instrumentation (5-12). This can translate into reduced rate of significantly costly hardware complications and revisions (15,16,41).
There are significant marketing advantages to these technologies. Some of these technologies will be important factors in attracting top surgeons to hospitals. As patients become more actively involved in choosing where they consume healthcare, they may favour hospitals that are utilizing some of these advanced technologies (42).
Surgeons interested in incorporating these technologies in their practice should understand the importance of negotiating for these technologies upfront when beginning relationships with hospitals. The best negotiation outcomes come when shared interest in maximized (43). The hospital and surgeon should work together to realize the mutual gain from these technologies. Surgeons should maintain conviction that their training and practice has allowed the use of these technologies to provide safer and more effective care for patients.
Conclusions
Assistive technologies and prostheses for spinal fusion are evolving rapidly. This article serves as an encompassing reference to the current technologies. These technologies will play a significant role in the delivery of spinal health care in the future. All stakeholders stand to benefit from the increased value these technologies bring to patient care.
Acknowledgments
Funding: None.
Footnote
Reporting Checklist: The authors have completed the Narrative Review reporting checklist. Available at https://jss.amegroups.com/article/view/10.21037/jss-21-107/rc
Peer Review File: Available at https://jss.amegroups.com/article/view/10.21037/jss-21-107/prf
Conflicts of Interest: All authors have completed the ICMJE uniform disclosure form (available at https://jss.amegroups.com/article/view/10.21037/jss-21-107/coif). GMM serves as an unpaid editorial board member of Journal of Spine Surgery, and receives consulting fees from LifeHealthcare Australia and Globus Medical. TAWQ holds shares in SeaSpine and is a fulltime employee for an organization that promotes several pieces of the technology discussed in the manuscript. VJR has no conflicts of interest to declare.
Ethical Statement: The authors are accountable for all aspects of the work in ensuring that questions related to the accuracy or integrity of any part of the work are appropriately investigated and resolved.
Open Access Statement: This is an Open Access article distributed in accordance with the Creative Commons Attribution-NonCommercial-NoDerivs 4.0 International License (CC BY-NC-ND 4.0), which permits the non-commercial replication and distribution of the article with the strict proviso that no changes or edits are made and the original work is properly cited (including links to both the formal publication through the relevant DOI and the license). See: https://creativecommons.org/licenses/by-nc-nd/4.0/.
References
- Elswick CM, Strong MJ, Joseph JR, et al. Robotic-Assisted Spinal Surgery: Current Generation Instrumentation and New Applications. Neurosurg Clin N Am 2020;31:103-10. [Crossref] [PubMed]
- Joseph JR, Smith BW, Liu X, et al. Current applications of robotics in spine surgery: a systematic review of the literature. Neurosurg Focus 2017;42:E2. [Crossref] [PubMed]
- McKenzie DM, Westrup AM, O'Neal CM, et al. Robotics in spine surgery: A systematic review. J Clin Neurosci 2021;89:1-7. [Crossref] [PubMed]
- Liounakos JI, Chenin L, Theodore N, et al. Robotics in Spine Surgery and Spine Surgery Training. Oper Neurosurg (Hagerstown) 2021;21:35-40. [Crossref] [PubMed]
- Kim HJ, Jung WI, Chang BS, et al. A prospective, randomized, controlled trial of robot-assisted vs freehand pedicle screw fixation in spine surgery. Int J Med Robot 2017; [Crossref] [PubMed]
- Hyun SJ, Kim KJ, Jahng TA, et al. Minimally Invasive Robotic Versus Open Fluoroscopic-guided Spinal Instrumented Fusions: A Randomized Controlled Trial. Spine (Phila Pa 1976) 2017;42:353-8. [Crossref] [PubMed]
- Han X, Tian W, Liu Y, et al. Safety and accuracy of robot-assisted versus fluoroscopy-assisted pedicle screw insertion in thoracolumbar spinal surgery: a prospective randomized controlled trial. J Neurosurg Spine 2019; Epub ahead of print. [Crossref] [PubMed]
- Kim HJ, Kang KT, Chun HJ, et al. Comparative study of 1-year clinical and radiological outcomes using robot-assisted pedicle screw fixation and freehand technique in posterior lumbar interbody fusion: A prospective, randomized controlled trial. Int J Med Robot 2018;14:e1917. [Crossref] [PubMed]
- Tarawneh AM, Salem KM. A Systematic Review and Meta-analysis of Randomized Controlled Trials Comparing the Accuracy and Clinical Outcome of Pedicle Screw Placement Using Robot-Assisted Technology and Conventional Freehand Technique. Global Spine J 2021;11:575-86. [Crossref] [PubMed]
- Li HM, Zhang RJ, Shen CL. Accuracy of Pedicle Screw Placement and Clinical Outcomes of Robot-assisted Technique Versus Conventional Freehand Technique in Spine Surgery From Nine Randomized Controlled Trials: A Meta-analysis. Spine (Phila Pa 1976) 2020;45:E111-9. [Crossref] [PubMed]
- Fan Y, Du JP, Liu JJ, et al. Accuracy of pedicle screw placement comparing robot-assisted technology and the free-hand with fluoroscopy-guided method in spine surgery: An updated meta-analysis. Medicine (Baltimore) 2018;97:e10970. [Crossref] [PubMed]
- Mason A, Paulsen R, Babuska JM, et al. The accuracy of pedicle screw placement using intraoperative image guidance systems. J Neurosurg Spine 2014;20:196-203. [Crossref] [PubMed]
- Du JP, Fan Y, Wu QN, et al. Accuracy of Pedicle Screw Insertion Among 3 Image-Guided Navigation Systems: Systematic Review and Meta-Analysis. World Neurosurg 2018;109:24-30. [Crossref] [PubMed]
- Hecht N, Yassin H, Czabanka M, et al. Intraoperative Computed Tomography Versus 3D C-Arm Imaging for Navigated Spinal Instrumentation. Spine (Phila Pa 1976) 2018;43:370-7. [Crossref] [PubMed]
- Schröder ML, Staartjes VE. Revisions for screw malposition and clinical outcomes after robot-guided lumbar fusion for spondylolisthesis. Neurosurg Focus 2017;42:E12. [Crossref] [PubMed]
- Liounakos JI, Kumar V, Jamshidi A, et al. Reduction in complication and revision rates for robotic-guided short-segment lumbar fusion surgery: results of a prospective, multi-center study. J Robot Surg 2021;15:793-802. [Crossref] [PubMed]
- Urakov TM, Chang KH, Burks SS, et al. Initial academic experience and learning curve with robotic spine instrumentation. Neurosurg Focus 2017;42:E4. [Crossref] [PubMed]
- Xiang L, Zhou Y, Wang H, et al. Significance of preoperative planning simulator for junior surgeons' training of pedicle screw insertion. J Spinal Disord Tech 2015;28:E25-9. [Crossref] [PubMed]
- Malham GM, Wells-Quinn T. What should my hospital buy next?-Guidelines for the acquisition and application of imaging, navigation, and robotics for spine surgery. J Spine Surg 2019;5:155-65. [Crossref] [PubMed]
- Navarro-Ramirez R, Lang G, Lian X, et al. Total Navigation in Spine Surgery; A Concise Guide to Eliminate Fluoroscopy Using a Portable Intraoperative Computed Tomography 3-Dimensional Navigation System. World Neurosurg 2017;100:325-35. [Crossref] [PubMed]
- Medtronic. O-arm. Dose Considerations. Available online: https://www.medtronic.com/us-en/healthcare-professionals/products/neurological/surgical-imaging-systems/o-arm/dose-considerations.html
- Wood MJ, McMillen J. The surgical learning curve and accuracy of minimally invasive lumbar pedicle screw placement using CT based computer-assisted navigation plus continuous electromyography monitoring - a retrospective review of 627 screws in 150 patients. Int J Spine Surg 2014;8:27. [Crossref] [PubMed]
- Su AW, McIntosh AL, Schueler BA, et al. How Does Patient Radiation Exposure Compare With Low-dose O-arm Versus Fluoroscopy for Pedicle Screw Placement in Idiopathic Scoliosis? J Pediatr Orthop 2017;37:171-7. [Crossref] [PubMed]
- Villard J, Ryang YM, Demetriades AK, et al. Radiation exposure to the surgeon and the patient during posterior lumbar spinal instrumentation: a prospective randomized comparison of navigated versus non-navigated freehand techniques. Spine (Phila Pa 1976) 2014;39:1004-9. [Crossref] [PubMed]
- Kim CW, Lee YP, Taylor W, et al. Use of navigation-assisted fluoroscopy to decrease radiation exposure during minimally invasive spine surgery. Spine J 2008;8:584-90. [Crossref] [PubMed]
- Jiang B, Pennington Z, Azad T, et al. Robot-Assisted versus Freehand Instrumentation in Short-Segment Lumbar Fusion: Experience with Real-Time Image-Guided Spinal Robot. World Neurosurg 2020;136:e635-45. [Crossref] [PubMed]
- iData Research. U.S. Surgical Robotics and Navigation Market 2019-2025. Available online: https://idataresearch.com/product/surgical-robotics-and-navigation-market/
- Vaishnav AS, Louie P, Gang CH, et al. Technique, Time Demand, Radiation Exposure, and Outcomes of Skin-anchored Intraoperative 3D Navigation in Minimally Invasive Posterior Cervical Laminoforaminotomy. Clin Spine Surg 2022;35:31-7. [Crossref] [PubMed]
- Malham GM, Parker RM. Early experience of placing image-guided minimally invasive pedicle screws without K-wires or bone-anchored trackers. J Neurosurg Spine 2018;28:357-63. [Crossref] [PubMed]
- Jakubovic R, Guha D, Gupta S, et al. High Speed, High Density Intraoperative 3D Optical Topographical Imaging with Efficient Registration to MRI and CT for Craniospinal Surgical Navigation. Sci Rep 2018;8:14894. [Crossref] [PubMed]
- U.S. FDA. 510(k) Premarket Notification. Available online: https://www.accessdata.fda.gov/scripts/cdrh/cfdocs/cfpmn/pmn.cfm?ID=K171651
- Naeem K, Farber SH, Bhargava M, et al. Prone Single-Position Lateral Lumbar Interbody Fusion With Posterior Decompression and Pedicle Screw Fixation for the Treatment of Grade II Spondylolisthesis: 2-Dimensional Operative Video. Oper Neurosurg (Hagerstown) 2021;21:E119-20. [Crossref] [PubMed]
- Walker CT, Godzik J, Xu DS, et al. Minimally Invasive Single-Position Lateral Interbody Fusion With Robotic Bilateral Percutaneous Pedicle Screw Fixation: 2-Dimensional Operative Video. Oper Neurosurg (Hagerstown) 2019;16:E121. [Crossref] [PubMed]
- Seeking Alpha. Medtronic plc (MDT) CEO Omar Ishrak on Q4 2019 Results - Earnings Call Transcript. Available online: https://seekingalpha.com/article/4266146-medtronic-plc-mdt-ceo-omar-ishrak-on-q4-2019-results-earnings-call-transcript
- The Motley Fool. Globus Medical (GMED) Q1 2021 Earnings Call Transcript, 2021. Available online: https://www.fool.com/earnings/call-transcripts/2021/05/05/globus-medical-gmed-q1-2021-earnings-call-transcri/
- Engelman DT, Boyle EM Jr, Benjamin EM. Addressing the imperative to evolve the hospital new product value analysis process. J Thorac Cardiovasc Surg 2018;155:682-5. [Crossref] [PubMed]
- Casalino LP, Khullar D. Value-Based Purchasing and Physician Professionalism. JAMA 2019;322:1647-8. [Crossref] [PubMed]
- CMS. CMS’ Value-Based Programs. Available online: https://www.cms.gov/Medicare/Quality-Initiatives-Patient-Assessment-Instruments/Value-Based-Programs/Value-Based-Programs
- Parker SL, Adogwa O, Witham TF, et al. Post-operative infection after minimally invasive versus open transforaminal lumbar interbody fusion (TLIF): literature review and cost analysis. Minim Invasive Neurosurg 2011;54:33-7. [Crossref] [PubMed]
- Goldstein CL, Macwan K, Sundararajan K, et al. Perioperative outcomes and adverse events of minimally invasive versus open posterior lumbar fusion: meta-analysis and systematic review. J Neurosurg Spine 2016;24:416-27. [Crossref] [PubMed]
- Menger RP, Savardekar AR, Farokhi F, et al. A Cost-Effectiveness Analysis of the Integration of Robotic Spine Technology in Spine Surgery. Neurospine 2018;15:216-24. [Crossref] [PubMed]
- Boys JA, Alicuben ET, DeMeester MJ, et al. Public perceptions on robotic surgery, hospitals with robots, and surgeons that use them. Surg Endosc 2016;30:1310-6. [Crossref] [PubMed]
- Hall L. eidtor. Negotiation Strategies for Mutual Gain: The Basic Seminar of the Program on Negotiation at Harvard Law School. Thousand Oaks, CA, USA: SAGE Publications, Inc., 1993. Available online: http://sk.sagepub.com/books/negotiation