Three-dimensional printing versus freehand surgical techniques in the surgical management of adolescent idiopathic spinal deformity
Introduction
Three-dimensional (3D) printing technology was first developed in the 1980s (1,2). Through the printing of titanium hardware implants and in preoperative planning and patient education (3), 3D printing has found increasing application within the field of orthopaedic surgery. Patient-specific 3D-printed guides for pedicle screw (PS) insertion in spinal deformity surgery offer an alternative to image-guided, robotic, and freehand methods. The 3D guides are printed using advanced imaging, are customized to patients, and are placed directly onto posterior elements of the vertebral level to be instrumented intraoperatively with openings that mechanically guide drilling, tapping, and screw placement into each pedicle or vertebral body (Figures 1,2). Reported overall accuracy for pedicle screw misplacement ranges from 5–15% (4). Although these estimates are low, Sarwahi et al. (5) argued that this reported screw misplacement does not reflect the potential impact on patient morbidity; after evaluating PS placement on a per-patient basis, the authors found that misplaced screws are of greater concern than previously believed. Recently, Sugawara et al. conducted a prospective multicenter study and found that patient-specific 3D guides offered improved accuracy for PS insertion (6).
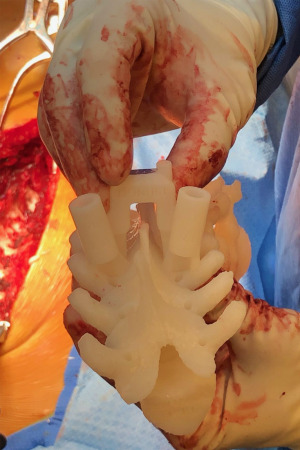
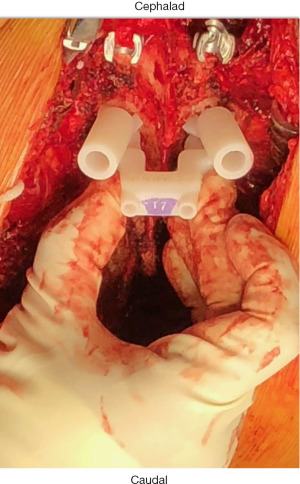
Customized surgical drill guides that can be sterilized and utilized intraoperatively constitute a relatively new application of 3D printing technology (1,7). As such, printing the models remains expensive and the benefits and costs of 3D printed guides in adolescent idiopathic scoliosis (AIS) instrumentation have yet to be characterized or documented in the extant literature (1,7). Our hypothesis asked, if we utilize 3D printing technology in AIS operative fixation, will it change perioperative patient outcomes? Therefore, the objective of this retrospective cohort study was to investigate the benefits and costs of AIS fusion conducted with and without 3D-printed guides at a single institution. We present the following article in accordance with the STROBE reporting checklist (available at https://jss.amegroups.com/article/view/10.21037/jss-22-28/rc).
Methods
This analysis was conducted in accordance with the Declaration of Helsinki (as revised in 2013). The study was given Institutional Review Board (IRB) exemption status by the ethics review board of Yale University, with individual consent waived for this retrospective analysis.
3D guides
Pedicle screws were placed with the assistance of 3D printed guides (Figure 1) until the authors’ institution acquired a 3D fluoroscopy unit, which enabled intraoperative verification of screw placement. Following this, all patients were managed with freehand surgical technique, which was followed by intraoperative 3-dimensional fluoroscopy verification of appropriate screw placement (8). The ZIEHM C-arm was utilized for intraoperative fluoroscopy. FIREFLY® Pedicle Screw Navigation Guides were obtained from Mighty Oak Medical®. Preoperative computed topography (CT) scans were conducted with slice thickness of 0.625 mm and pitch of 1.375. The material used to manufacture patient-specific components is an epoxy resin indicated for use in stereolithography systems. Patient-contacting materials used for non-patient-specific components are manufactured in accordance with ASTM F899 or F136 (9). In the pediatric population, surgery was to occur within six months of CT scanning for FIREFLY® compatibility.
Data collection
This retrospective cohort study included all patients with AIS who underwent spinal fusion at a single institution by a single surgeon from December 2019 through July 2021. Sample size was estimated via continuous outcome superiority trial with significance level (alpha) of 5%, and power (1-beta) of 90% (10). Patients with neuromuscular scoliosis were excluded. Patient data, training level of surgical assist, intraoperative measures including length of surgery, blood loss, and indication for pedicle screw intraoperative replacement were collected. Additionally, perioperative outcome measures including length of hospital stay and curve correction, and total implant and 3D printing costs were collected. Intraoperative blood loss was collected as cell saver volume x3 for consistency. The postgraduate training year (PGY) of the first surgical assistant, including fellows, was recorded. Residents ranged in experience from PGY2 through PGY5. Fellows were considered PGY6 for statistical analyses. To minimize bias, screw placement time was recorded in minutes per spinal level by the neuromonitoring team rather than surgical team. In cases where this data was not available, minutes per screw were calculated by recording the total surgical time for screw placement, again as measured by the neuromonitoring team, and dividing this time by the total number of screws placed. The primary outcome evaluated was intraoperative blood loss. Secondary outcomes included time per screw placement, length of surgery, and length of hospitalization.
When the 3D guide could not be properly aligned intraoperatively on the bony spine, surgical instrumentation was completed with freehand techniques and the spinal level, side, and indicated reason were marked. This was most commonly encountered at the uppermost instrumented vertebra (UIV), where soft tissue constraints led to difficulty placing the guide on the posterior elements with appropriate fidelity of the bone to the 3D printed guide. There were no scenarios where a pedicle screw had to be removed following placement utilizing an appropriately-fitting 3D guide. Freehand pedicle screws were replaced and documented when a screw was found to be malpositioned on 3D fluoroscopic imaging, requiring intraoperative repositioning or removal. If the 3D guide did not fit appropriately intraoperatively, or spinal levels required fusion above or below the level planned by the 3D guide, screws were placed freehand.
Statistical analysis
Shapiro-Wilk test was used to determine data normality. Univariate analysis was conducted with Student’s t-test and Pearson’s chi-squared for normally distributed data. A Wilcoxon rank-sum (Mann-Whitney) test was used for nonparametric data. Multivariate analysis assessed for confounding and effect modification. Poisson modelling was used for multivariate analysis with heteroskedasticity. An interaction term was included to account for the observed relationship between time per screw placement and level of training (PGY year), which was treated as a categorical variable: resident, fellow, or attending. A P value of 0.05 was established for statistical significance. All analyses were conducted with use of STATA MP version 16 (StataCorp).
Results
A total of 29 patients were included in the study, 18 in the 3D cohort and 11 in the freehand (FH) cohort (Table 1). Between cohorts, there were no significant differences in patient age (P=0.93), gender (P=0.15), height (P=0.18), weight (P=0.40), or preoperative curve magnitude (P=0.42) (Table 1). On average, 3D guides were used in cases assisted by surgical trainees of lower training levels, as the median training level for freehand screw placement was PGY6 (i.e., fellow), compared to the median training level of cases done using 3D guide (PGY5; P=0.01) (Table 1). The 3D cohort (mean $26,215, SD =$6,374) had significantly higher implant costs than those of the FH cohort (mean $18,660; FH, SD =$5,587, P=0.003) (Table 1). The 3D cohort also had significantly reduced intraoperative blood loss (mean 357 mL, SD =123), compared to the FH cohort (mean 559 mL FH, SD =273, P=0.01) (Table 1). On univariate analysis, there was no significant difference in screw placement time by screw type (P=0.66) (Table 2). There were no significant differences between cohorts with respect to the length of postoperative hospitalization, number of spinal levels fused, presence of postoperative kyphosis, presence of postoperative lordosis, or coronal curve correction.
Table 1
Factor | Freehand | 3-D | P value | Test |
---|---|---|---|---|
N | 11 | 18 | ||
Age, years, mean (SD) | 14 (1.8) | 14.1 (1.4) | 0.93 | Two sample t-test |
Gender | 0.15 | Pearson’s chi-squared | ||
Male | 6 (55%) | 5 (28%) | ||
Female | 5 (45%) | 13 (72%) | ||
Risser Score | 0.74 | Pearson’s chi-squared | ||
0 | 0 (0%) | 2 (11%) | ||
1 | 2 (18%) | 1 (5%) | ||
2 | 1 (9%) | 1 (5%) | ||
3 | 1 (9%) | 2 (11%) | ||
4 | 6 (55%) | 9 (50%) | ||
5 | 1 (9%) | 3 (17%) | ||
Height, mean (cm) (SD) | 165 (7.7) | 161 (7.2) | 0.18 | Two sample t-test |
Weight, mean (kg) (SD) | 56 (9.2) | 60 (12.2) | 0.40 | Two sample t-test |
Training year of surgical assistant | 0.01 | Pearson’s chi-squared | ||
Resident (PGY 2-5) | 1 (9%) | 13 (72%) | ||
Fellow | 9 (82%) | 3 (17%) | ||
Attending | 1 (9%) | 2 (11%) | ||
Implant cost, mean ($) (SD) | 18,660.4 (5,586.8) | 26,214.9 (6,374.3) | 0.003 | Two sample t-test |
Supply cost, mean ($) (SD) | 3,366.2 (1,174.5) | 3,846.3 (1,453.9) | 0.36 | Two sample t-test |
Length of surgery, mean (hours) (SD) | 4.40 (1.1) | 4.5 (0.8) | 0.84 | Two sample t-test |
Intraoperative blood loss, mean (mL) (SD) | 559 (273) | 357 (123) | 0.01 | Two sample t-test |
Cell saver repletion (mL) (SD) | 179 (124) | 116 (71) | 0.14 | Two sample t-test |
Allogenic blood products used | 1 | 1 | 0.72 | Pearson’s chi-squared |
Length of hospitalization (days) (SD) | 3.1 (0.5) | 3.1 (0.8) | 0.94 | Two sample t-test |
Levels fused, mean (SD) | 11 (1.8) | 9.7 (2.9) | 0.20 | Two sample t-test |
Pre-op curve, mean (SD) | 58 (9.7) | 61 (11.5) | 0.42 | Two sample t-test |
Post-op curve, mean (SD) | 11 (5.1) | 11.4 (4.9) | 0.84 | Two sample t-test |
Curve change, mean (SD) | 47 (11) | 50 (9) | 0.42 | Two sample t-test |
Postoperative kyphosis, mean (SD) | 35.8 (6.4) | 35.4 (6.2) | 0.86 | Two sample t-test |
Postoperative lordosis, mean (SD) | 59.3 (11.7) | 56.3 (9.0) | 0.44 | Two sample t-test |
3-D, three-dimensional; PGY, post-graduate year.
Table 2
Factor | Freehand | 3-D | P value | Test |
---|---|---|---|---|
N | 307 | 263 | ||
Time per screw, median (minutes) (IQR) | 3.7 (1.7) | 4.0 (1.9) | 0.66 | Wilcoxon rank-sum |
3-D, three-dimensional.
Across 29 cases, 570 pedicle screws were placed, including 307 freehand and 263 with 3D guidance (Table 2).
On multivariate analysis, greater number of spinal levels fused was associated with significantly increased intraoperative blood loss (P=0.01), while 3D guided screw placement was associated with significantly decreased intraoperative blood loss (P=0.04) (Table 3). Patient age and training level of first assistant did not serve as statistically significant predictors of intraoperative blood loss (Table 3). Poisson modelling was used to account for data heteroskedasticity with an interaction term to account for a significant interaction between training level of first assist and 3D versus freehand techniques (Table 4, Figure 3). Screw placement time was significantly less for residents when 3D models were used compared to freehand (P<0.001) (Table 4, Figure 3). When the first assist was a fellow or attending level surgeon, freehand technique was significantly faster (P<0.001) (Table 4, Figure 3).
Table 3
Characteristics | Impact on blood loss | 95% CI | P value |
---|---|---|---|
Training level of first assistant | −13.9 | −135.0, 107.2 | 0.82 |
Number of spinal levels fused | 36.7 | 8.2, 65.1 | 0.01* |
Patient age | 1.2 | −46.2, 48.5 | 0.96 |
Screw type (3D vs. freehand) | −164.1 | −325.9, −2.3 | 0.04** |
3D, three-dimensional.*Greater number of spinal levels fused had significantly more blood loss. **Screws placed with 3D models had significantly less blood loss than freehand.
Table 4
Input | Time (minutes) | SE | 95% CI | P value |
---|---|---|---|---|
Freehand | ||||
Resident | 6.6 | 0.24 | 6.1, 7.1 | <0.001 |
Fellow | 3.6 | 0.05 | 3.5, 3.7 | <0.001 |
Attending | 3.9 | 0.13 | 3.6, 4.1 | <0.001 |
3D | ||||
Resident | 3.9 | 0.09 | 3.7, 4.1 | <0.001 |
Fellow | 5.1 | 0.46 | 4.2, 6.0 | <0.001 |
Attending | 4.9 | 0.01 | 4.8, 4.9 | <0.001 |
Poisson modelling was used to analyze the interaction term between screw type and training level of first assist when using freehand versus three-dimensional modelling techniques. 3D, three-dimensional.
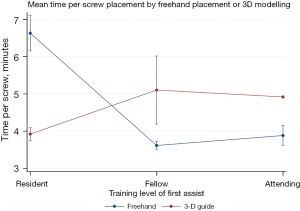
Discussion
This study is among the first (7,11,12) to examine the use of 3D printed technology in the surgical management of adolescent idiopathic scoliosis (AIS). This study suggests that the use of 3D printed guides decreases intraoperative blood loss when compared to the use of standard freehand pedicle screw placement. Additionally, for cases with a resident surgeon acting as first assist, the time per screw placement was significantly less when 3D guides were used compared to freehand technique. The use of 3D printed guides was associated with higher implant costs compared to cases done using traditional freehand technique for screw placement, with no significant difference between groups regarding other intraoperative performance measures or perioperative outcomes other than reduction in intraoperative blood loss.
In 2015, Yang et al. (7) conducted a retrospective study of 126 patients with Lenke 1 AIS who underwent posterior corrective surgery. Fifty of these patients had a preoperative 3D printed spine model made and had “simulated surgery” prior to their operation; the other patients had surgery using traditional freehand technique for screw placement without a preoperatively printed spine model. The patients who underwent posterior corrective surgery without a preoperatively printed 3D model had longer operative times, increased blood loss, greater transfusion volumes, and lower postoperative hemoglobin (7). The authors demonstrated the value of 3D printing in facilitating preoperative planning and enhancing a better preoperative understanding of the unique anatomical features of a malrotated spine. Their study, however, did not specifically examine the intraoperative use of 3D printed guides. Garg et al. (13) compared 10 patients treated with patient-specific-guides with 10 patients treated using freehand technique for screw placement and found greater accuracy and shorter operative times when using patient-specific guides.
Lopez et al. (11) recently conducted a systematic review of the use of 3D printed guides in spinal deformity surgery and found that cases performed with 3D printed guides reported greater accuracy of screw placement, shorter operative times, and no significant difference in estimated blood loss. A study by Talathi et al. (14) also showed that AIS cases first-assisted by a resident had higher blood loss than those assisted by fellows. Our analysis demonstrates that blood loss was significantly reduced when using 3D guides, despite the majority of 3D printed cases being performed with a less experienced first assistant. Other perioperative outcomes were equivalent between groups. A larger cohort study is needed to clarify the mechanism behind this decreased blood loss, as the total length of surgery was similar between groups and all other blood conservation measures (use of perioperative tranexamic acid, hypotensive anesthesia, etc.) were held constant in the present investigation. It is important to note that, in this study, the decreased blood loss in the 3D printed guides group did not translate to fewer blood transfusions between groups. Other perioperative characteristics were equivalent between the two groups. These findings suggest that the use of 3D printed guides may be a valuable training tool that can aid the education and proficiency of younger trainees without compromising patient safety and outcomes.
The total cost of spinal surgery completed with 3D printed guides was significantly more expensive than surgeries completed with freehand techniques, primarily due to implant costs. The increased average cost noted in this study of approximately $7,500 in the 3D-printed group relative to the freehand group is similar to that reported in prior studies (7,11). Costs of 3D printed surgical planning models vary broadly from $175 for a 3D printed template model to $5400 for sophisticated 3D printed spinal phantom training (11) in addition to overall costs of pedicle screws and other permanent implant components. One potential solution to these costs could include the use of alternative materials to manufacture 3D printed guides, which may be less expensive than polymer-based 3D printed models (11). Ultimately, further cost analyses, including cost-effectiveness analyses, may be warranted to determine if the increased cost of the 3D models is justified when similar perioperative outcomes have been demonstrated. It is possible that 3D guides may only be justified in academic training centers where surgical residents are regularly acting as first surgical assists.
It is possible that the true value of 3D printing is fully realized in more complex cases, where significant rotational deformity and dysplastic concave pedicles make safe pedicle screw placement particularly challenging. Pan et al. (15) demonstrated greater accuracy (96% vs. 89%) when using 3D printed mechanical guides in a comparison of spinal deformity cases done with 3D guidance versus those done freehand. Operative time was similar between groups. In contrast to this study, in which the average preoperative coronal curve magnitude of each cohort was 58° or 61° (Table 1), Pan et al. reported average curvatures in excess of 90°. Likewise Yang (7) found that preoperative 3D printing improved outcomes in curvatures in excess of 50°, but not in curvatures below this threshold. Selective utilization of 3D printing for more complex cases may serve as the optimal solution to ensure safety and accuracy while minimizing costs.
Limitations to this study include its relatively small sample size and lack of follow-up. In addition, pedicle screws placed freehand were assessed for accuracy with intraoperative 3D fluoroscopy, whereas the screws placed using 3D printed guides were not, precluding a true comparison between the two groups in terms of accuracy. Moreover, it was found that use of the 3D printed guide was often precluded due to body wall issues, preventing the guide from docking securely on the upper instrumented level. This resulted in the surgeon resorting to the FH method of screw placement or the placement of alternative anchors (hooks) at the top of the construct.
Conclusions
3D printed guides constitute an emerging tool in aiding safe and efficient placement of pedicle screws in pediatric spinal deformity surgery. This study shows that they may decrease intraoperative blood loss and time per screw placement for surgeons in training, but total operative time, curve correction, and other perioperative outcomes appear to be the same when compared to cases done with freehand technique. The associated cost of 3D guides, compared to freehand surgical techniques, are significantly greater due to the cost of product manufacturing. Future research to further evaluate the utility of 3D printed guides in spinal deformity surgery may include cost-effectiveness analyses, large, retrospective randomized studies, and larger cases series with longer patient follow-up. The findings described herein add credence to some of the known benefits associated with the use of 3D printed guides in pediatric spinal deformity correction surgery, which merit further investigation and innovation to refine their utility.
Acknowledgments
Funding: None.
Footnote
Reporting Checklist: The authors have completed the STROBE reporting checklist. Available at https://jss.amegroups.com/article/view/10.21037/jss-22-28/rc
Data Sharing Statement: Available at https://jss.amegroups.com/article/view/10.21037/jss-22-28/dss
Peer Review File: Available at https://jss.amegroups.com/article/view/10.21037/jss-22-28/prf
Conflicts of Interest: All authors have completed the ICMJE uniform disclosure form (available at https://jss.amegroups.com/article/view/10.21037/jss-22-28/coif). DAT serves on the wellness committee for the Pediatric Orthopaedic Society of North America (POSNA) and the quality safety and value committee for the Scoliosis Research Society (SRS). DAT also receives money for lectures and teaching from OrthoPediatrics and is a consultant for Depuy Synthes. The other authors have no conflicts of interest to declare.
Ethical Statement: The authors are accountable for all aspects of the work in ensuring that questions related to the accuracy or integrity of any part of the work are appropriately investigated and resolved. This analysis was conducted in accordance with the Declaration of Helsinki (as revised in 2013). The study was given Institutional Review Board (IRB) exemption status by the ethics review board of Yale University, with individual consent waived for this retrospective analysis.
Open Access Statement: This is an Open Access article distributed in accordance with the Creative Commons Attribution-NonCommercial-NoDerivs 4.0 International License (CC BY-NC-ND 4.0), which permits the non-commercial replication and distribution of the article with the strict proviso that no changes or edits are made and the original work is properly cited (including links to both the formal publication through the relevant DOI and the license). See: https://creativecommons.org/licenses/by-nc-nd/4.0/.
References
- Garg B, Mehta N. Current status of 3D printing in spine surgery. J Clin Orthop Trauma 2018;9:218-25. [Crossref] [PubMed]
- Pugliese L, Marconi S, Negrello E, et al. The clinical use of 3D printing in surgery. Updates Surg 2018;70:381-8. [Crossref] [PubMed]
- Zamborsky R, Kilian M, Jacko P, et al. Perspectives of 3D printing technology in orthopaedic surgery. Bratisl Lek Listy 2019;120:498-504. [Crossref] [PubMed]
- Hicks JM, Singla A, Shen FH, et al. Complications of pedicle screw fixation in scoliosis surgery: a systematic review. Spine (Phila Pa 1976) 2010;35:E465-70. [Crossref] [PubMed]
- Sarwahi V, Wendolowski SF, Gecelter RC, et al. Are We Underestimating the Significance of Pedicle Screw Misplacement? Spine (Phila Pa 1976) 2016;41:E548-55. [Crossref] [PubMed]
- Sugawara T, Kaneyama S, Higashiyama N, et al. Prospective Multicenter Study of a Multistep Screw Insertion Technique Using Patient-Specific Screw Guide Templates for the Cervical and Thoracic Spine. Spine (Phila Pa 1976) 2018;43:1685-94. [Crossref] [PubMed]
- Yang M, Li C, Li Y, et al. Application of 3D rapid prototyping technology in posterior corrective surgery for Lenke 1 adolescent idiopathic scoliosis patients. Medicine (Baltimore) 2015;94:e582. [Crossref] [PubMed]
- Bauer JM, Moore JA, Rangarajan R, et al. Intraoperative CT Scan Verification of Pedicle Screw Placement in AIS to Prevent Malpositioned Screws: Safety Benefit and Cost. Spine Deform 2018;6:662-8. [Crossref] [PubMed]
- Mighty Oak Medical. FIREFLY ® Pedicle Screw Navigation Guide. 2018. Available online: https://www.accessdata.fda.gov/cdrh_docs/pdf16/K162419.pdf
- Pocock SJ. The Size of a Clinical Trial. In: Clinical Trials: A Practical Approach. 1983;123-141.
- Lopez CD, Boddapati V, Lee NJ, et al. Three-Dimensional Printing for Preoperative Planning and Pedicle Screw Placement in Adult Spinal Deformity: A Systematic Review. Global Spine J 2021;11:936-49. [Crossref] [PubMed]
- Moore HG, Samuel AM, Burroughs PJ, et al. Use of intraoperative navigation for posterior spinal fusion in adolescent idiopathic scoliosis surgery is safe to consider. Spine Deform 2021;9:403-10. [Crossref] [PubMed]
- Garg B, Gupta M, Singh M, et al. Outcome and safety analysis of 3D-printed patient-specific pedicle screw jigs for complex spinal deformities: a comparative study. Spine J 2019;19:56-64. [Crossref] [PubMed]
- Talathi NS, Flynn JM, Pahys JM, et al. The Effect of the Level of Training of the First Assistant on the Outcomes of Adolescent Idiopathic Scoliosis Surgery. J Bone Joint Surg Am 2019;101:e23. [Crossref] [PubMed]
- Pan Y, Lü GH, Kuang L, et al. Accuracy of thoracic pedicle screw placement in adolescent patients with severe spinal deformities: a retrospective study comparing drill guide template with free-hand technique. Eur Spine J 2018;27:319-26. [Crossref] [PubMed]