Fusion assessment in standalone lateral lumbar interbody fusion: 3D-printed titanium versus polyetheretherketone (PEEK) cages
IntroductionOther Section
Lateral lumbar interbody fusion (LLIF) has become increasingly popular in the recent decade due to its safety and effectiveness for the treatment of degenerative spinal diseases (1-4). In past years, there is growing attention on standalone LLIF (SA-LLIF) in particular due to the ability of the procedure to maintain segmental stability through preservation of the anterior and posterior longitudinal ligaments and insertion of large cages that span the apophyseal ring bilaterally (5-9). Irrespective of the technique used, solid bony fusion of the treated level remains the final goal of any lumbar interbody fusion.
Interbody cages are manufactured from different materials such as polyetheretherketone (PEEK) and titanium (Ti), with PEEK representing the most commonly used material in the past because of its good mechanical and chemical properties (10-13). However, one main disadvantage of PEEK is the relatively low osseointegration, radiologically known as the ‘PEEK-Halo effect’, a biofilm layer around the surface of the implant (12,14,15). Therefore, bone tends to grow around the cage in order to bind to the host bone and create a solid fusion mass (13). With advancing technology and manufacturing capabilities, interbody cages are designed with complex features (16). The most advanced cages to date are 3D-printed Ti cages with a porous architecture that mimic trabecular bone. These cages have more compressive shear strength under physical force, maximize bone-to-implant contact and show less early subsidence in a SA-LLIF setting compared to traditionally used PEEK cages (14,17-21). With regards to fusion, 3D-printed Ti cages are reported to be more osteoconductive in laboratory settings compared to PEEK cages (18,22). Furthermore, due to increased friction created by the porous surface, these 3D-printed Ti cages have reduced micromotion that leads to bony on- and in-growth (18,23). The possibility of increased osteoblastic activity is of special interest when it comes to assessing fusion (24).
In this study, we defined two independent groups, an early and a late group based on the availability of postoperative computed tomography (CT) scans. Within those groups, we compared evidence of fusion between 3D-printed Ti cages and PEEK cages. We hypothesized that 3D-printed Ti cages show higher fusion rates at an early timepoint compared to PEEK cages. We present the following article in accordance with the STROBE reporting checklist (available at https://jss.amegroups.com/article/view/10.21037/jss-22-17/rc).
MethodsOther Section
Study population and design
The study was conducted in accordance with the Declaration of Helsinki (as revised in 2013). This study was approved by the Hospital for Special Surgery Institutional Review Board (IRB# 2014-097) and informed consent was waived due to the retrospective nature of this study. Between November 2016 and January 2020, data of consecutive patients undergoing SA-LLIF at a single academic institution were reviewed. The inclusion criteria were as follows: (I) patients aged 18 years and older, (II) patients undergoing SA-LLIF from L1/2 to L4/5, (III) radiological follow-up with CT scans between 6–12 months (early group) and >12 months (late group), and (IV) pre-operative CT scans for the assessment of bone mineral density (BMD). The surgical indications for SA-LLIF were also reviewed.
Data collection
As potential contributing factors for fusion, demographic and surgical variables were collected including age, body mass index (BMI), gender, race, history of smoking, diabetes mellitus, surgical diagnosis, use of recombinant human bone morphogenetic protein-2 (rh-BMP2), level(s) of fusion and cage dimensions. Additionally, volumetric bone mineral density (vBMD) was retrospectively assessed using quantitative computed tomography (QCT; Mindways Software, Inc., Austin, TX, USA) on preoperative CT scans within 6 months prior to surgery as described in a previous study of ours (21). Bone status was assessed according to the “American College of Radiology guidelines for the Performance of Musculoskeletal Quantitative Computed Tomography (Qct)” (25).
Surgical technique and implants
At a single high volume spine center, all patients underwent SA-LLIF performed by one of four fellowship-trained orthopedic spine surgeons with at least 10 years of experience with the LLIF procedure. The appropriate cage size was determined based on preoperative imaging in combination with intraoperative cage templating. All cages in both groups were lordotic. Moreover, all cages were packed with rh-BMP2. The implants were positioned to span the apophyseal ring bilaterally. During the surgical procedure, intraoperative neuromonitoring was performed. Two PEEK cage systems [XLIF (NuVasive, Inc., San Diego, CA, USA)] & the COUGAR system (DePuy Spine Inc., Raynham, MA, USA) or two 3D-printed Ti cages systems [Modulus XLIF (NuVasive, Inc.) & Lateral Spine Truss System (4WEB Medical, Inc., Frisco, TX, USA)] were utilized during the study period.
Fusion assessment
Evidence of fusion was assessed on multiplanar reconstructed CT scans using the hospital’s Picture Archiving and Communication System (PACS) software Sectra IDS7 Version 22.7 (Sectra AB, Linköping, Sweden). Assessment of fusion was independently performed by two researcher-physicians not involved in any of the procedures. Additionally, all findings were compared to the radiological report and the latter used for further evaluation in cases of disagreement. Presence of fully bridged interbody trabecular bone or continuous bone through the cage in any plain was considered as evidence of fusion.
Postoperative follow-up CT imaging was not standardized among surgeons, possibly to reduce preventable radiation exposure. Routine radiological follow-up was carried out using standing plain X-rays at 6 months as a standard of care. In patients fulfilling inclusion criteria for one of the two timepoints, the indication for CT was recorded as “standard of care”, “discomfort/pain” or “fall”.
Statistical analysis
Proportions were used for categorial variables to summarize their distribution.
Comparisons between categorical variables were performed utilizing Fisher’s exact test and the χ2 test. The Shapiro-Wilk test was used to assess for normal distribution of continuous variables. Comparisons between normally distributed continuous variables were performed using the Student t-test, and the Mann-Whitney U test was used for the comparisons between non-normally distributed continuous variables. Statistical analysis was performed with SPSS Statistics version 23.0 (IBM Corp., Armonk, NY, USA). The statistical significance was set as P≤0.05.
ResultsOther Section
Study population
We retrospectively reviewed the data of 157 consecutive patients undergoing SA-LLIF with a minimum of 6-month radiological follow-up. Of these, 91 patients (136 levels) fulfilled our inclusion criteria: 49 patients (72 levels) had a CT scan between 6–12 months postoperatively and formed the early group and 42 patients (64 levels) had a CT scan after a minimum of 12 months postoperatively and formed the late group for fusion assessment (Figure 1). Both the early and late group were independent groups and included different patients based on the availability of CT scans during follow-up. For the early group, CT scans were performed on average 8.2±1.8 months (Ti: 8.4±1.8; PEEK: 8.0±1.8, P=0.376) postoperatively, and for the late group on average 18.9±7.7 months (Ti: 18.8±6.9; PEEK: 18.9±8.4, P=0.756) after surgery.
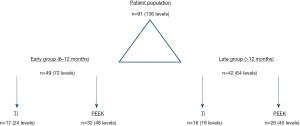
Our predominantly White/Caucasian population (94.5%) consisted of 50 male and 41 females with an average age at surgery of 61.9±12.7 years. vBMD analysis revealed 8 osteoporotic patients. In the early group, no statistically significant difference was found in demographic characteristics between Ti and PEEK cages, whereas in the late group, patients treated with PEEK cages were significantly older compared to those receiving Ti cages (P=0.023). Demographic characteristics and surgical diagnoses stratified for each group and both cage materials are summarized in Tables 1,2.
Table 1
Variables | Early group | Late group | |||||||
---|---|---|---|---|---|---|---|---|---|
Total (n=49) | Ti (n=17) | PEEK (n=32) | P value | Total (n=42) | Ti (n=16) | PEEK (n=26) | P value | ||
Gender, n (%) | |||||||||
Male | 27 (55.1) | 10 (58.8) | 17 (53.1) | 0.769§ | 23 (54.8) | 10 (62.5) | 13 (50.0) | 0.530§ | |
Female | 22 (44.9) | 7 (41.2) | 15 (46.9) | 0.769§ | 19 (45.2) | 6 (37.5) | 13 (50.0) | 0.530§ | |
Race, n (%) | |||||||||
Caucasian/White | 46 (93.9) | 17 (100.0) | 29 (90.6) | 0.428** | 40 (95.2) | 15 (93.8) | 25 (96.2) | 0.325** | |
African-American | 2 (4.1) | 0 | 2 (6.3) | 0.428** | 1 (2.4) | 0 | 1 (3.8) | 0.325** | |
Other | 1 (2.0) | 0 | 1 (3.1) | 0.428** | 1 (2.4) | 1 (6.3) | 0 | 0.325** | |
Age at surgery, years, mean ± SD (range) |
60.6±13.2 (22–83) |
56.8±13.5 (22–81) |
62.6±12.8 (36–83) |
0.143* | 63.5±12.3 (24–82) |
58.1±12.9 (24–77) |
66.9±10.9 (39–82) |
0.023* | |
BMI (kg/m2), mean ± SD (range) | 28.0±4.8 (19.2–41.1) |
27.4±5.0 (19.2–41.1) |
28.3±4.7 (19.6–39.6) |
0.559* | 27.5±4.6 (19.6–42.6) |
27.7±5.6 (23.6–42.6) |
27.4±4.0 (19.6–33.7) |
0.891* | |
Smoking status, n (%) | |||||||||
Never | 27 (55.1) | 11 (64.7) | 16 (50.0) | 0.479** | 22 (52.4) | 10 (62.5) | 12 (46.2) | 0.197** | |
Former | 20 (40.8) | 5 (29.4) | 15 (46.9) | 0.479** | 19 (45.2) | 5 (31.3) | 14 (53.8) | 0.197** | |
Current | 2 (4.1) | 1 (5.9) | 1 (3.1) | 0.479** | 1 (2.4) | 1 (6.2) | 0 | 0.197** | |
Diabetes mellitus, n (%) | 5 (10.2) | 1 (5.9) | 4 (12.5) | 0.646§ | 1 (2.4) | 0 | 1 (3.8) | 1.000§ | |
QCT vBMD (mg/cm3), mean ± SD (range) |
130.0±40.1 (47.1–265.0) |
142.3±44.6 (63.1–265.0) |
123.4±36.6 (47.1–213.6) |
0.119* | 130.2±37.2 (47.1–197.5) |
137.8±26.5 (88.6–183.0) |
125.5±42.3 (47.1–197.5) |
0.252* | |
QCT normal, n (%) | 29 (59.2) | 13 (76.5) | 16 (50.0) | 0.126§ | 26 (61.9) | 12 (75.0) | 14 (53.9) | 0.206§ | |
QCT osteopenia, n (%) | 15 (30.6) | 3 (17.6) | 12 (37.5) | 0.202§ | 13 (31.0) | 4 (25.0) | 9 (34.6) | 0.733§ | |
QCT osteoporosis, n (%) | 5 (10.2) | 1 (5.9) | 4 (12.5) | 0.646§ | 3 (7.1) | 0 | 3 (11.5) | 0.275§ |
§, Fisher’s exact test; *, Student t-test; **, Chi-squared test. Ti, titanium; PEEK, polyetheretherketone; BMI, body mass index; QCT vBMD, quantitative computed tomography volumetric bone mineral density.
Table 2
Variables, n (%) | Early group | Late group | |||||||
---|---|---|---|---|---|---|---|---|---|
Total (n=49) | Ti (n=17) | PEEK (n=32) | P value | Total (n=42) | Ti (n=16) | PEEK (n=26) | P value | ||
Degenerative disc disease | 20 (40.8) | 9 (52.9) | 11 (34.4) | 0.237§ | 15 (35.7) | 9 (56.3) | 6 (23.1) | 0.047§ | |
Spinal stenosis | 34 (69.4) | 12 (70.6) | 22 (68.8) | 1.000§ | 31 (73.8) | 11 (68.8) | 20 (76.9) | 0.720§ | |
Foraminal stenosis | 24 (49.0) | 6 (35.3) | 18 (56.3) | 0.232§ | 19 (45.2) | 7 (43.8) | 12 (46.2) | 1.000§ | |
Spondylolisthesis | 35 (71.4) | 14 (82.4) | 21 (65.6) | 0.323§ | 32 (76.2) | 13 (81.3) | 19 (73.1) | 0.715§ | |
Herniated disc | 4 (8.2) | 1 (5.9) | 3 (9.4) | 1.000§ | 1 (2.4) | 1 (6.3) | 0 | 0.381§ | |
Neurogenic claudication | 2 (4.1) | 2 (11.8) | 0 | 0.116§ | 6 (14.3) | 4 (25.0) | 2 (7.7) | 0.180§ | |
Scoliosis | 13 (26.5) | 4 (23.5) | 9 (28.1) | 1.000§ | 12 (28.6) | 2 (12.5) | 10 (38.5) | 0.090§ |
It must be mentioned that some patients had multiple diagnoses. §, Fisher’s exact test. Ti, titanium; PEEK, polyetheretherketone.
Of 136 treated levels, 54 (39.7%) were single level SA-LLIFs. L4/5 (41.9%) was the level most often treated followed by L3/4 (33.8%). While the early group did not show a significant difference between 3D-printed Ti cages and PEEK cages for the number of levels treaded, the late group had significantly more multilevel procedures with PEEK compared to the 3D-printed Ti cages (P=0.006). Surgical characteristics are shown in Table 3.
Table 3
Variables | Early group | Late group | |||||||
---|---|---|---|---|---|---|---|---|---|
Total | Ti | PEEK | P value | Total | Ti | PEEK | P value | ||
No. of patients | 49 | 17 | 32 | 42 | 16 | 26 | |||
No. of levels | 72 | 24 | 48 | 64 | 19 | 45 | |||
Levels treated, n (%) | |||||||||
1 | 30 (61.2) | 13 (76.5) | 17 (53.1) | 0.087** | 24 (57.2) | 14 (87.4) | 10 (38.5) | 0.006** | |
2 | 16 (32.7) | 2 (11.7) | 14 (43.8) | 0.087** | 14 (33.3) | 1 (6.3) | 13 (50.0) | 0.006** | |
3 | 2 (4.1) | 1 (5.9) | 1 (3.1) | 0.087** | 4 (9.5) | 1 (6.3) | 3 (11.5) | 0.006** | |
4 | 1 (2.0) | 1 (5.9) | 0 | 0.087** | – | – | – | 0.006** | |
Spinal levels, n (%) | |||||||||
L1/2 | 3 (4.2) | 2 (8.3) | 1 (2.1) | 0.273§ | 2 (3.1) | 2 (10.5) | 0 | 0.139§ | |
L2/3 | 13 (18.1) | 4 (16.7) | 9 (18.8) | 1.000§ | 15 (23.4) | 5 (26.3) | 10 (22.2) | 0.746§ | |
L3/4 | 23 (31.9) | 7 (29.2) | 16 (33.3) | 0.764§ | 23 (35.9) | 4 (21.1) | 19 (42.2) | 0.004§ | |
L4/5 | 33 (45.8) | 11 (45.8) | 22 (45.8) | 1.000§ | 24 (37.6) | 8 (42.1) | 16 (35.6) | 0.531§ | |
Cage height (mm), n (%) | |||||||||
8 | 11 (15.3) | 2 (8.3) | 9 (18.8) | 0.316§ | 15 (23.5) | 3 (15.8) | 12 (26.6) | 0.521 | |
10 | 49 (68.1) | 21 (87.5) | 28 (58.3) | 0.016§ | 42 (65.6) | 16 (84.2) | 26 (57.8) | 0.049§ | |
12 | 12 (16.6) | 1 (4.2) | 11 (22.9) | 0.051§ | 7 (10.9) | 0 | 7 (15.6) | 0.094§ | |
Cage width (mm), n (%) | |||||||||
18 | 3 (4.2) | 0 | 3 (6.3) | 0.546§ | 5 (7.8) | 0 | 5 (11.1) | 0.310§ | |
22 | 49 (68.1) | 24 (100.0) | 25 (52.1) | <0.001§ | 47 (73.4) | 19 (100.0) | 28 (62.2) | 0.001§ | |
26 | 20 (27.7) | 0 | 20 (41.6) | <0.001§ | 12 (18.8) | 0 | 12 (26.7) | 0.013§ | |
Cage length (mm), n (%) | |||||||||
40 | 1 (1.4) | 0 | 1 (2.1) | 1.000§ | 10 (15.6) | 2 (10.5) | 8 (17.8) | 0.710§ | |
45 | 9 (12.5) | 3 (12.5) | 6 (12.5) | 1.000§ | 22 (34.4) | 8 (42.1) | 14 (31.1) | 0.406§ | |
50 | 19 (26.4) | 9 (37.5) | 10 (20.8) | 0.161§ | 22 (34.4) | 8 (42.1) | 14 (31.1) | 0.406§ | |
55 | 30 (41.7) | 10 (41.7) | 20 (41.7) | 1.000§ | 9 (14.1) | 1 (5.3) | 8 (17.8) | 0.260§ | |
60 | 12 (16.6) | 2 (8.3) | 10 (20.8) | 0.314§ | 1 (1.5) | 0 | 1 (2.2) | 1.000§ | |
65 | 1 (1.4) | 0 | 1 (2.1) | 1.000§ | – | – | – | – | |
Cage lordosis, n (%) | |||||||||
10° | 70 (97.2) | 22 (91.7) | 48 (100.0) | 0.108§ | 55 (85.9) | 18 (94.7) | 37 (82.2) | 0.260§ | |
15° | 2 (2.8) | 2 (8.3) | 0 | 0.108§ | 9 (14.1) | 1 (5.3) | 8 (17.8) | 0.260§ |
**, Chi-squared test; §, Fisher’s exact test. Ti, titanium; PEEK, polyetheretherketone.
Fusion assessment
The indication for CT scans during the follow-up periods did not differ between early and late groups (Table 4). After an average follow-up of 8.2±1.8 months in the early group, 23/24 (95.8%) of levels treated with 3D-printed Ti cages compared to 30/48 (62.5%) of levels treated with PEEK cages showed evidence of fusion (P=0.002). In the late group, after an average follow-up of 18.9±7.7 months, 18/19 (94.7%) of levels treated with 3D-printed Ti cages and 36/45 (80.0%) of levels treated with PEEK cages showed evidence of fusion (P=0.756) (Figure 2). When comparing multilevel procedures, the early group displayed evidence of fusion in 90.9% of levels treated with 3D-printed Ti cages compared to 58.1% of levels treated with PEEK cages. For the late group, 100.0% of patients treated with 3D-printed Ti cages versus 80.0% with PEEK cages showed evidence of fusion.
Table 4
Variables | Early group | Late group | |||||||
---|---|---|---|---|---|---|---|---|---|
Total | Ti | PEEK | P value | Total | Ti | PEEK | P value | ||
No. of patients | 49 | 17 | 32 | – | 42 | 16 | 26 | – | |
No. of levels | 72 | 24 | 48 | – | 64 | 19 | 45 | – | |
Reason for follow-up CT, n (%) | |||||||||
Standard of care | 18 (36.8) | 6 (35.3) | 12 (37.5) | 0.383* | 6 (14.3) | 2 (12.5) | 4 (15.4) | 0.859* | |
Discomfort/pain | 30 (61.2) | 10 (58.8) | 20 (62.5) | 0.383* | 32 (76.2) | 12 (75.0) | 20 (76.9) | 0.859* | |
Fall | 1 (2.0) | 1 (5.9) | 0 | 0.383* | 4 (9.5) | 2 (12.5) | 2 (7.7) | 0.859* | |
Evidence of fusion per level, n (%) | |||||||||
No | 19 (26.4) | 1 (4.2) | 18 (37.5) | 0.002§ | 10 (15.6) | 1 (5.3) | 9 (20.0) | 0.258§ | |
Yes | 53 (73.6) | 23 (95.8) | 30 (62.5) | 0.002§ | 54 (84.4) | 18 (94.7) | 36 (80.0) | 0.258§ | |
Follow-up (months), mean ± SD (range) |
8.2±1.8 (6.0–11.6) |
8.4±1.8 (6.1–11.6) |
8.0±1.8 (6.0–11.6) |
0.376** | 18.9±7.7 (12.0–38.8) |
18.8±6.9 (12.3–34.3) |
18.9±8.4 (12.0–38.8) |
0.756** |
§, Fisher’s exact test; *, Chi-squared test; **, Mann-Whitney U test. Ti, titanium; PEEK, polyetheretherketone; CT, computed tomography.
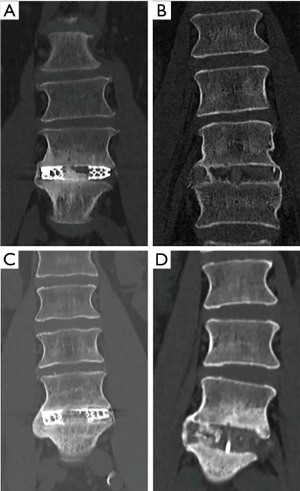
DiscussionOther Section
To the authors knowledge, this is the first study comparing evidence of fusion between 3D-printed Ti cages and PEEK cages at two different timepoints in the setting of SA-LLIF. Our results demonstrated that after an average of 8.2±1.8 months, Ti cages achieved significantly higher fusion rates compared to PEEK cages. However, in the independent late group, no significant difference in fusion rates was found between the two cage materials after an average of 18.9±7.7 months. These findings support the assumption that 3D-printed Ti cages with a porous architecture are more osteoconductive and therefore tend to fuse earlier than PEEK cages.
McGilvray et al. (18) performed a comparative animal study that directly compared PEEK and 3D-printed Ti cages. They displayed significant differences for biochemical, micro-CT and histological performance of the Ti cages, with the porous Ti cages significantly reducing the range of motion (ROM) and increasing bone ingrowth compared to PEEK cages. Similar to equal results were reported in a recently published ovine model study by Van Horn et al. (16) In their micro-CT analysis, the bone volume (in-growth) in the 3D-printed Ti cage was significantly higher after 6 weeks postoperatively compared to the PEEK cage, but not after 12 weeks. Furthermore, in a histological analysis 3D-printed Ti cages showed significantly greater bony on-growth than PEEK cages at 6 and 12 weeks postoperatively. Of note, the bony on-growth was 2.7-times higher in the 3D-printed Ti cages than the PEEK cage at 6 weeks and 2.6-times higher at 12 weeks. However, even though it remains quantitatively unclear how bony in-/on-growth affects spinal fusion, Van Horn et al. (16) concluded that it is reasonable that more bony growth leads to greater stability of the treated segment and thus might prevent pseudarthrosis (16).
In general, non-union rates for lumbar fusion have been reported to be around 0–40%, with modern techniques such as interbody cages, pedicle screw fixation and bone graft substitutes lowering this number to 0–10% (26-29). Many studies have reported fusion rates for LLIF in the past, but only a few have explicitly focused on SA-LLIF (30-35). Investigating fusion rates of PEEK cages, Marchi et al. (35) evaluated 52 patients undergoing single-level SA-LLIF and reported a solid fusion of only 67.3% after 12 months and 86.5% after 24 months. They assessed fusion on X-rays and CT scans and defined fusion as bridging bone connecting the adjacent vertebral bodies through or around the implant (equal to our definition), less than 5° of angular motion, less than or equal to 3 mm of translation and an absence of radiolucent lines around more than 50% of either of the implant surfaces. In a prospective, randomized controlled study by Pimenta et al. (33) including 30 patients undergoing single-level SA-LLIF, a fusion rate of 100% after 36 months was reported that was independent of the bone graft material (Silicate Calcium Phosphate or rh-BMP2) used within the cage. The authors also used X-rays and CT scans for the fusion assessment as described above. In another study including 74 patients with 98 treated levels, the same study group reported a fusion rate of 91% at 12 months (36). A recently published systematic review analyzing 22 SA-LLIF studies treated with PEEK cages for various surgical indications showed an overall fusion rate of 85.6% per person (37). The average follow-up for all included articles was 19.0 months, ranging from 6–62 months. Of note, fusion rates were not reported per treated level, but per person, and fusion assessment was done either on X-rays (11/22 articles) or on CT scans (11/22 articles). Interestingly, this review showed that fusion rates were higher in multilevel procedures (1 level: 89.2%, 2 levels: 90.5%, 3 levels: 96.9%) and fusion rates by each treated level were comparable throughout the lumbar spine (L1/2 96.6%, L2/3 95.8%, L3/4 96.0%, and L4/5 95.5%).
Currently, the literature about porous 3D-printed Ti cages in the setting of SA-LLIF is scarce. However, there are some studies investigating 3D-printed Ti cages with posterior pedicle screw fixation that also report fusion rates. Krafft et al. (38) published the first study evaluating 3D-printed Ti cages in 30 patients (59 levels). While their primary endpoint was cage subsidence, they also reported radiographic signs of fusion per level which was 62.7% after an average follow-up of 11.6 months (range, 3–23 months), which is considerably lower than our SA-LLIF fusion rate. X-rays were used for fusion assessment, however, so their results must be interpreted with caution. Mokawem et al. (11) assessed fusion rates with 3D-printed lamellar Ti cages using silicate-substituted calcium phosphate bone graft in 43 patients (87 levels). At 12 months postoperatively, they reported a fusion rate per level of 100% on CT scans.
There are several limitations to this study. First, both the early and late group were independent groups and included different patients based on the availability of CT scans during follow-up. A longitudinal assessment of fusion was therefore not applicable, and no time course of fusion can be shown. Second, CT scans were not performed as a standard of care due to the involvement of different surgeons. Therefore, patients included in this study represent a subset of our patient population and consequently, there might have been unmeasured factors that led to selection bias. Third, the relatively small sample size of our groups and the single-center retrospective design limits the generalizability of our results further. Given these limitations, any conclusions must be interpreted with appropriate caution.
ConclusionsOther Section
Our study demonstrated that in SA-LLIF 3D-printed Ti cages showed significantly higher fusion rates at an early timepoint compared to PEEK cages. However, the difference in fusion rates between Ti and PEEK cages was found not to be significantly different at a later timepoint in another patient group. In our cohort, this might support the assumption that 3D-printed Ti cages with a porous architecture are possible more osteoconductive compared to PEEK and possible tend to fuse earlier.
AcknowledgmentsOther Section
Funding: None.
FootnoteOther Section
Reporting Checklist: The authors have completed the STROBE reporting checklist. Available at https://jss.amegroups.com/article/view/10.21037/jss-22-17/rc
Data Sharing Statement: Available at https://jss.amegroups.com/article/view/10.21037/jss-22-17/dss
Conflicts of Interest: All authors have completed the ICMJE uniform disclosure form (available at https://jss.amegroups.com/article/view/10.21037/jss-22-17/coif). AAS declares financial interests: Ortho Development Corp, Vestia Ventures MiRUS Investment LLC, ISPH II LLC, ISPH 3 LLC, VBros Venture Partners X Centinel Spine, Clariance Inc., Kuros Bioscience AG, Medical Device Business Services Inc., DePuy Synthes Products Inc., Medical Device Business Services Inc. and Spinal Kinetcs Inc. FPC declares financial interests: NuVasive Inc., Bonovo Orthopedics Inc., Healthpoint Capital Partners LP, ISPH II LLC, Ivy Healthcare Capital Partners LLC, Medical Device Partners II LLC, Medical Device Partners III LLC, Orthobond Corporation, Spine Biopharma LLC, Tissue Differentiation Intelligence LLC, VBVP VI LLC, Woven Orthopedics Technologies, 4Web Medical/4Web Inc., Spine Biopharma LLC and Beatrice & Samuel A. Seaver Foundation. FPG declares financial interests: NuVasive Inc., Ortho Development Corp, Zimmer Biomet Holdings INC, Bonovo Orthopedics Inc., Liventa Bioscience (AF Cell Medical), Paradigm Spine LLC, Healthpoint Capital Partners LP, Alphatec Holdings LLC, LANX Inc., Centinel Spine Inc. (fka Raymedica LLC), Tissue Differentiation Intelligence LLC, Spine Kinetics Inc., DePuy Synthes Spine and NuVasive Inc. APH declares financial interests: 4Web Medical, NuVasive Inc. and Kuros Bioscience B.V. The other authors have no conflicts of interest to disclose.
Ethical Statement: The authors are accountable for all aspects of the work in ensuring that questions related to the accuracy or integrity of any part of the work are appropriately investigated and resolved. The study was conducted in accordance with the Declaration of Helsinki (as revised in 2013). This study was approved by the Hospital for Special Surgery Review Board (IRB# 2014-097) and informed consent was waived due to the retrospective nature of this study.
Open Access Statement: This is an Open Access article distributed in accordance with the Creative Commons Attribution-NonCommercial-NoDerivs 4.0 International License (CC BY-NC-ND 4.0), which permits the non-commercial replication and distribution of the article with the strict proviso that no changes or edits are made and the original work is properly cited (including links to both the formal publication through the relevant DOI and the license). See: https://creativecommons.org/licenses/by-nc-nd/4.0/.
ReferencesOther Section
- Ozgur BM, Aryan HE, Pimenta L, et al. Extreme Lateral Interbody Fusion (XLIF): a novel surgical technique for anterior lumbar interbody fusion. Spine J 2006;6:435-43. [Crossref] [PubMed]
- Rodríguez-Vela J, Lobo-Escolar A, Joven E, et al. Clinical outcomes of minimally invasive versus open approach for one-level transforaminal lumbar interbody fusion at the 3- to 4-year follow-up. Eur Spine J 2013;22:2857-63. [Crossref] [PubMed]
- Park P, Than KD, Mummaneni PV, et al. Factors affecting approach selection for minimally invasive versus open surgery in the treatment of adult spinal deformity: analysis of a prospective, nonrandomized multicenter study. J Neurosurg Spine 2020; Epub ahead of print. [Crossref] [PubMed]
- Ozgur BM, Agarwal V, Nail E, et al. Two-year clinical and radiographic success of minimally invasive lateral transpsoas approach for the treatment of degenerative lumbar conditions. SAS J 2010;4:41-6. [Crossref] [PubMed]
- Li H, Li J, Tao Y, et al. Is stand-alone lateral lumbar interbody fusion superior to instrumented lateral lumbar interbody fusion for the treatment of single-level, low-grade, lumbar spondylolisthesis? J Clin Neurosci 2021;85:84-91. [Crossref] [PubMed]
- Bocahut N, Audureau E, Poignard A, et al. Incidence and impact of implant subsidence after stand-alone lateral lumbar interbody fusion. Orthop Traumatol Surg Res 2018;104:405-10. [Crossref] [PubMed]
- Malham GM, Ellis NJ, Parker RM, et al. Maintenance of Segmental Lordosis and Disk Height in Stand-alone and Instrumented Extreme Lateral Interbody Fusion (XLIF). Clin Spine Surg 2017;30:E90-8. [Crossref] [PubMed]
- Pimenta L, Turner AW, Dooley ZA, et al. Biomechanics of lateral interbody spacers: going wider for going stiffer. ScientificWorldJournal 2012;2012:381814. [Crossref] [PubMed]
- Tempel ZJ, McDowell MM, Panczykowski DM, et al. Graft subsidence as a predictor of revision surgery following stand-alone lateral lumbar interbody fusion. J Neurosurg Spine 2018;28:50-6. [Crossref] [PubMed]
- Han CM, Lee EJ, Kim HE, et al. The electron beam deposition of titanium on polyetheretherketone (PEEK) and the resulting enhanced biological properties. Biomaterials 2010;31:3465-70. [Crossref] [PubMed]
- Mokawem M, Katzouraki G, Harman CL, et al. Lumbar interbody fusion rates with 3D-printed lamellar titanium cages using a silicate-substituted calcium phosphate bone graft. J Clin Neurosci 2019;68:134-9. [Crossref] [PubMed]
- Massaad E, Fatima N, Kiapour A, et al. Polyetheretherketone Versus Titanium Cages for Posterior Lumbar Interbody Fusion: Meta-Analysis and Review of the Literature. Neurospine 2020;17:473. [Crossref] [PubMed]
- McGilvray KC, Waldorff EI, Easley J, et al. Evaluation of a polyetheretherketone (PEEK) titanium composite interbody spacer in an ovine lumbar interbody fusion model: biomechanical, microcomputed tomographic, and histologic analyses. Spine J 2017;17:1907-16. [Crossref] [PubMed]
- Campbell PG, Cavanaugh DA, Nunley P, et al. PEEK versus titanium cages in lateral lumbar interbody fusion: a comparative analysis of subsidence. Neurosurg Focus 2020;49:E10. [Crossref] [PubMed]
- Phan K, Hogan JA, Assem Y, et al. PEEK-Halo effect in interbody fusion. J Clin Neurosci 2016;24:138-40. [Crossref] [PubMed]
- Van Horn MR, Beard R, Wang W, et al. Comparison of 3D-printed titanium-alloy, standard titanium-alloy, and PEEK interbody spacers in an ovine model. Spine J 2021;21:2097-103. [Crossref] [PubMed]
- Chatham LS, Patel VV, Yakacki CM, et al. Interbody Spacer Material Properties and Design Conformity for Reducing Subsidence During Lumbar Interbody Fusion. J Biomech Eng 2017; [Crossref] [PubMed]
- McGilvray KC, Easley J, Seim HB, et al. Bony ingrowth potential of 3D-printed porous titanium alloy: a direct comparison of interbody cage materials in an in vivo ovine lumbar fusion model. Spine J 2018;18:1250-60. [Crossref] [PubMed]
- Willems K, Lauweryns P, Verleye G, et al. Randomized Controlled Trial of Posterior Lumbar Interbody Fusion With Ti- and CaP-Nanocoated Polyetheretherketone Cages: Comparative Study of the 1-Year Radiological and Clinical Outcome. Int J Spine Surg 2019;13:575-87. [Crossref] [PubMed]
- Najeeb S, Bds ZK, Bds SZ, et al. Bioactivity and Osseointegration of PEEK Are Inferior to Those of Titanium: A Systematic Review. J Oral Implantol 2016;42:512-6. [Crossref] [PubMed]
- Adl Amini D, Okano I, Oezel L, et al. Evaluation of cage subsidence in standalone lateral lumbar interbody fusion: novel 3D-printed titanium versus polyetheretherketone (PEEK) cage. Eur Spine J 2021;30:2377-84. [Crossref] [PubMed]
- Rao PJ, Pelletier MH, Walsh WR, et al. Spine interbody implants: material selection and modification, functionalization and bioactivation of surfaces to improve osseointegration. Orthop Surg 2014;6:81-9. [Crossref] [PubMed]
- Yoon BJ, Xavier F, Walker BR, et al. Optimizing surface characteristics for cell adhesion and proliferation on titanium plasma spray coatings on polyetheretherketone. Spine J 2016;16:1238-43. [Crossref] [PubMed]
- Olivares-Navarrete R, Hyzy SL, Gittens RA. Rough titanium alloys regulate osteoblast production of angiogenic factors. Spine J 2013;13:1563-70. [Crossref] [PubMed]
- The American College of Radiology. Acr–Spr–Ssr Practice Parameter for the Performance of Musculoskeletal Quantitative Computed Tomography (Qct). 2018. Available online: https://www.acr.org/-/media/ACR/Files/Practice-Parameters/QCT.pdf?la=en
- Watkins R 4th, Watkins R 3rd, Hanna R. Non-union rate with stand-alone lateral lumbar interbody fusion. Medicine (Baltimore) 2014;93:e275. [Crossref] [PubMed]
- Hsu WK, Nickoli MS, Wang JC, et al. Improving the clinical evidence of bone graft substitute technology in lumbar spine surgery. Global Spine J 2012;2:239-48. [Crossref] [PubMed]
- Hackenberg L, Halm H, Bullmann V, et al. Transforaminal lumbar interbody fusion: a safe technique with satisfactory three to five year results. Eur Spine J 2005;14:551-8. [Crossref] [PubMed]
- Christensen FB, Hansen ES, Eiskjaer SP, et al. Circumferential lumbar spinal fusion with Brantigan cage versus posterolateral fusion with titanium Cotrel-Dubousset instrumentation: a prospective, randomized clinical study of 146 patients. Spine (Phila Pa 1976) 2002;27:2674-83. [Crossref] [PubMed]
- Phillips FM, Isaacs RE, Rodgers WB, et al. Adult degenerative scoliosis treated with XLIF: clinical and radiographical results of a prospective multicenter study with 24-month follow-up. Spine (Phila Pa 1976) 2013;38:1853-61. [Crossref] [PubMed]
- Caputo AM, Michael KW, Chapman TM, et al. Extreme lateral interbody fusion for the treatment of adult degenerative scoliosis. J Clin Neurosci 2013;20:1558-63. [Crossref] [PubMed]
- Rodgers WB, Gerber EJ, Patterson JR. Fusion after minimally disruptive anterior lumbar interbody fusion: Analysis of extreme lateral interbody fusion by computed tomography. SAS J 2010;4:63-6. [Crossref] [PubMed]
- Pimenta L, Marchi L, Oliveira L, et al. A prospective, randomized, controlled trial comparing radiographic and clinical outcomes between stand-alone lateral interbody lumbar fusion with either silicate calcium phosphate or rh-BMP2. J Neurol Surg A Cent Eur Neurosurg 2013;74:343-50. [Crossref] [PubMed]
- Castro C, Oliveira L, Amaral R, et al. Is the lateral transpsoas approach feasible for the treatment of adult degenerative scoliosis? Clin Orthop Relat Res 2014;472:1776-83. [Crossref] [PubMed]
- Marchi L, Abdala N, Oliveira L, et al. Stand-alone lateral interbody fusion for the treatment of low-grade degenerative spondylolisthesis. ScientificWorldJournal 2012;2012:456346. [Crossref] [PubMed]
- Marchi L, Abdala N, Oliveira L, et al. Radiographic and clinical evaluation of cage subsidence after stand-alone lateral interbody fusion. J Neurosurg Spine 2013;19:110-8. [Crossref] [PubMed]
- Manzur MK, Steinhaus ME, Virk SS, et al. Fusion rate for stand-alone lateral lumbar interbody fusion: a systematic review. Spine J 2020;20:1816-25. [Crossref] [PubMed]
- Krafft PR, Osburn B, Vivas AC, et al. Novel Titanium Cages for Minimally Invasive Lateral Lumbar Interbody Fusion: First Assessment of Subsidence. Spine Surg Relat Res 2020;4:171-7. [Crossref] [PubMed]