Anatomical reconstruction of complete burst vertebral fracture case report—combined reduction methods and armed kyphoplasty with stents filled with bone graft
Highlight box
Key findings
• Surgical methods and outcomes of vertebral fracture treatment that allows for a complete burst fracture to be fully anatomically restored by a posterior minimally invasive approach.
What is known and what is new?
• Minimally invasive techniques concerning the augmentation of the vertebtral body have acquired popularity thanks to their capacity to stabilize the anterior column via the percutaneous posterior pathway.
• We present a detailed description of a surgical technique for a complete burst fracture combining indirect and direct reduction methods and vertebral body interior replacement with stents filled with bone graft.
What is the implication, and what should change now?
• Currently, complete burst fracture can be anatomically restored by a posterior minimally invasive approach with promising clinical e imaging outcomes.
• Long-term prospective studies are necessary in order to prove the effectiveness and advantages of this surgical option.
Introduction
The high rate of morbidity of anterior approaches to spine reconstruction led to an excessive trend to deal with vertebral compression fractures through posterior pedicular instrumentation. Thus, many times in more comminuted fractures, there was a tendency to increase the number of fixed levels with pedicle screws in order to increase stability (1-3). Nevertheless, a deficit of support in the anterior column, a zone where 80% of axial loads occur, necessarily overburdens the posterior instrumentation. This can give rise to loss of vertebral body height and instrumentation failure, as well as local and post-traumatic segmental kyphosis, leading to a clinical and functional impact (1-6). This way, techniques to augment the fractured vertebral body that are minimally invasive have acquired accentuated popularity as their can stabilize the anterior spine using the percutaneous posterior pathway. This allows for positive results regarding relief of symptoms, quality of life, functional indices, and convalescence speed, as well as biomechanics and spine anatomy restoration (2,3,5-14). Expandable intravertebral implants consist of devices capable of controlled self-expansion that are applied percutaneously through posterior transpedicular access. The application of these devices, or armed kyphoplasty, besides enabling the immediate stabilization benefits of kyphoplasty and vertebroplasty, also permits restoration and maintenance of vertebral height (7-13). Once the height of vertebral endplates is restored, these are supported in a mechanical way by the expanded device, which works as an interior sustentaculum, assuring a resistant anterior support of the vertebral body’s height performed by posterior percutaneous transpedicular access (1,7-10,12-14). Our goal is to present a case report and chosen methods to obtain an anatomical surgical reconstruction of complete burst vertebral fracture using pedicular screws instrumentation and expandable intravertebral implants. This article is presented in accordance with the CARE reporting checklist (available at https://jss.amegroups.com/article/view/10.21037/jss-22-117/rc).
Case presentation
We present the case of a 50-year-old male patient, previously autonomous in daily activities, with no relevant medical history, who was been referred to the emergency department after falling from a ladder of about 3 meters high, with trauma to the thoracolumbar region. The patient complains of severe thoracolumbar pain [classified as grade 7/10 on the Visual Analog Pain Scale (VAS) (15)] associated with the inability to move her trunk. There were no radiculalgia or neurological deficits. The patient reports that after the fall he felt sudden and unbearable back pain, which was highly disabling. For a moment he felt completely blocked, unable to react and without feeling his lower limbs. The initial radiograph showed significant flattening of the L1 vertebral body, while the computed tomography (CT) confirmed a complete burst fracture with marked comminution and flattening of about 2/3 of L1 body’s height (Figure 1). There was also a marked posterior wall retropulsion, occupying more than half of the spinal canal. Posterior elements of L1 and adjacent vertebras were intact. Lamina, spinous and facet processes were not fractured and interspinous and interfacetary spaces were normal. The patient was admitted to hospital and the following surgical intervention was performed: L1 laminectomy, percutaneous pedicle instrumentation D11-D12-L2-L3 and indirect fracture reduction, followed by armed kyphoplasty with stents filled with bone graft. Given the accentuated posterior wall retropulsion with neurological compression, even in the absence of neurological deficits, we chose to perform local laminectomy in order to obtain the greatest possible neurological decompression. Using the NForce® system supported by monoaxial screws at D12 and L2 (polyaxial screws at D11 and L3 levels), indirect reduction maneuvers were performed on the cortical ring of the vertebral body by progressive lordosis and distraction (Figure 2). When the desired vertebral reduction was achieved, the rods were applied bilaterally. Next, armed kyphoplasty was performed using expansive VBS® intravertebral implants (Figures 3,4) (7). Initially, the entry points of the transpedicular canals to the vertebral body were obtained by direct visualization laterally to the rods and fluoroscopy support, which identifies the access needles in the lateral portion of the pedicles (Figure 3). It should be noted that in the anteroposterior view, the pedicle entry points are usually partially covered by the previous instrumentation rods (Figure 3). Despite the rods-access needles overlap, it is possible to safely control the position of the needles using fluoroscopic support and progress in the anteroposterior view to the medial border of the pedicles (Figure 3B). Then we progress only after viewing the needles already inside the vertebral body in the lateral view (Figure 3C). After drilling and impacting these bone canals to the vertebral body, the kyphoplasty balloons were placed in the desired position inside the vertebral body and inflated in order to obtain intrasomatic cavities prior to the application of the stents (Figure 3). Then, the VBS® expandable intravertebral implants were applied and expanded in a controlled manner in the same positions where the balloons were previously expanded, thus allowing for a direct reduction to be carried out in the fracture focus. A mixture of granules of regional cancellous bone graft (from the previous laminectomy) and bone bank allograft was then applied through the working cannulas directly inside the stents. The bone granules are applied one by one through the working cannula bilaterally from its entrance to the inside of the stents and impacted progressively until they are completely filled, which occurs when the impactor stops progressing inside the stents. The fluoroscopic anteroposterior view can also confirm the complete filling of the interior of the stents with bone granules, acquiring a darker tone in comparison with the previous acquisitions (Figure 4G,4H). It should be noted that these granules are previously prepared in order to have a diameter smaller than 5mm, which is the usual diameter of the working cannula for applying the stents, so that they can progress without difficulty to the inside of the implants. The patient started progressively getting up and walking the next day and was discharged after 3 days, walking independently. According to patient’s perspective the first stand up after surgery was what surprised him the most. The strength in the trunk has returned and the lower back pain almost disappeared. Radiographs and CT were performed in the postoperative period, which confirmed correct application of instrumentation and satisfactory reduction of the fracture, as well as adequate bone filling inside the stents bilaterally (Figure 1A,1B,1C1,1D1,1E1,1F1,1G1 and Figure 5; vertebral measures improvement at Figure 6). At 3-month follow-up, the patient walked autonomously, with sporadic residual axial pain that he classified as grade 1/10 on VAS, without any other intercurrence. He had no limitations in activities of daily living and an Oswestry Disability Index (ODI) score evaluated as 12% (15,16). After 3 years, the patient is asymptomatic, satisfied, and without limitations in daily activities, with an ODI score of 4%. Control CT at 3 years postoperatively demonstrates vertebral body fracture healing and maintenance of the vertebral heights recorded in the immediate postoperative period (Figure 6), without signs of loosening, migration, or failure of intrasomatic stents or pedicle screws, neither bone graft resorption, which indicates its osseointegration and healing (Figure 5). The predictable disc degeneration gaseous signs below and above the L1 vertebral body should be highlighted, representing the consequence of the disc injury and depressurization occurred when the vertebral endplates were fractured (Figure 5). All procedures performed in this study were in accordance with the ethical standards of the institutional and/or national research committees and with the Helsinki Declaration (as revised in 2013). Written informed consent was obtained from the patient for publication of this case report and accompanying images. A copy of the written consent is available for review by the editorial office of this journal.
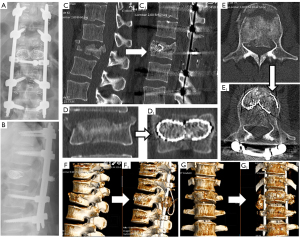
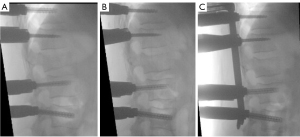
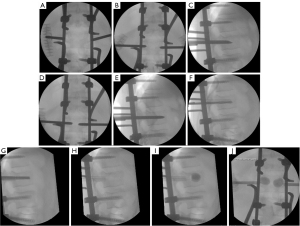
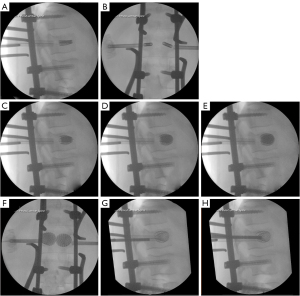
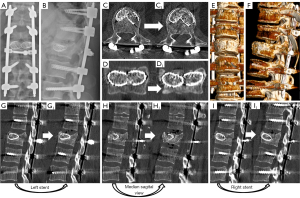
Patient’s perspective
A previously autonomous patient who regularly practices recreational sports, including surfing, reports that after the fall he felt sudden and unbearable back pain, which was highly disabling. He immediately realized that something very serious had happened. For a period that seemed like an eternity, he felt completely blocked, unable to react and without feeling his lower limbs. The patient always felt very anxious during hospitalization and until the surgical intervention. The first stand up after surgery was what surprised him the most. The strength in the trunk has returned and the lower back pain almost disappeared. The recovery was extremely fast and satisfactory. Currently, the patient has returned to surfing and does not have any limitations.
Discussion
Expandable intravertebral implants bring about the concept of direct fracture reduction (Figures 7,8), which is carried on by an expanded implant at the exact fracture site within the vertebral body. If the fracture happens due to compression forces, those implants will work in the reverse way: they expand the vertebral body, which is the opposite mechanism to that responsible for the fracture, thus being an effective method of fracture reduction. Only direct reduction by expandable intrassomatic implants allows for the restoration of the vertebral endplates’ central part. Therefore, these implants are needed in order to reach a complete anatomical reduction of a vertebral body compression fracture (Figures 7,8) (1,2,6,11,17-20). In complete burst fractures [A4 AOSpine-type fractures (21)], such as our clinical case, characterized by severe destruction of the vertebral body, we usually apply two types of reduction. It is firstly indirect (by pedicular instrumentation) and afterwards direct (by expandable implants), aiming at obtaining the best anatomical reconstruction of both the cortical body ring and the entire endplates (Figures 7,8) (12,13).
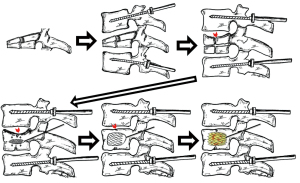
The authors are supportive of the relevance of attaining an anatomical restoration, or approximately, of vertebral bodies which have been affected by compression fractures. This happens through the correction of vertebral height, the morphology of vertebral endplates and the vertebral kyphosis angle. The goal is to assure that the spine is functionally and biomechanically closer to what it was like before being fractured. The recreation of the original position of the commonly affected intervertebral disc is assured by restoring the original anatomy of vertebral endplates, which boosts its suitable pressurization and healing. At the same time, it reduces the invagination of the nucleus pulposus to the interior of the vertebral body, which is likely to compromise bone healing. This permits a more effective physiological load damping, probably reducing or even preventing progression to osteodegenerative and disc alterations of this vertebral segment, as well of as its adjacent levels (2,6,7). Nevertheless, after 3-year follow-up, we can identify clear signs of initial degenerative changes of the adjacent discs to the L1 vertebral body (Figure 5). This demonstrates not only the clear evidence of disc depressurization and injury that occurred when the vertebral endplates were fractured, but also that disc degenerative cascade inevitably initiates and, in theory may only be minimized by surgical anatomical restoration of the endplates, however not prevented.
The injury of intraosseous blood vessels during a vertebral fracture can compromise blood supply of the vertebral body bone tissue and inhibit bone healing, which seems to be the most probable cause of its evolution to non-union, pseudoarthrosis and necrosis, even though there is no unequivocal scientific evidence of this (22). Nonetheless, we still do not have any exam that enables to biologically and vascularly figure out whether a specific pattern of a vertebra fracture leads to the disruption of major intraosseous blood vessels, which would allow to perceive its evolution to pseudarthrosis. The authors consider that in complete burst fractures with high comminution, intraosseous vascularization is impaired. Therefore, we opt to perform, as an initial treatment of this fracture, an immediate interior replacement of the vertebral body, resorting to an armed kyphoplasty with VBS® expandable intravertebral implants. This way, next to performing indirect reduction maneuvers and after restoring the vertebral body’s cortical ring anatomy, whenever bone healing is not expected, the authors choose to reconstruct/replace the vertebral body’s interior right away with cylindrical implants, the stents (Figure 8). The rationale is to assume intraosseous arterial network injury and incapability of healing, so we act ad initium as a preventive method of vertebral body nonunion and pseudarthrosis, avoiding a high-risk fracture to progress to one of the most concerning and unpredictable challenges in spinal traumatology. In addition, the expansion of the stents permits further reduction of the vertebral body, which is a direct reduction. This occurs by multidirectional impaction of the bone trabeculae that is around, particularly through the elevation of vertebral endplates’ central portion (Figure 7). It also ensures the maintenance of the reduction, working as an interior support or sustentaculum. There are authors who defend the intrassomatic application of polymethylmethacrylate (PMMA) cement in what concerns complete burst fractures with bone which is healthy, even in youngsters. In fact, they state that fracture healing occurs even when there is cement, since fracture gaps that exist between cement and bone trabeculae are filled by bone callus (2,6,14,23-25). Notwithstanding, regarding this type of fractures in healthy bone and active middle-aged and young individuals, our option is to fill the stents using bone graft, often with cancellous allograft granules from bone bank. Then, the graft is applied considering the need for minimal impaction so that it does not hinder nutritional diffusion or bone matrix structure until its revascularization. We intend to achieve its colonization by vascular invasion and osteoprogenitor cells, bone incorporation and a vertebra which is biomechanically similar to the ones that are unfractured, aiming at a more physiological vertebrae regarding the distribution of loads than the ones which are filled with PMMA. The latter is a biologically inert cement, which impairs the vertebral body future instrumentation and affects its biological activity and healing, as well as its remodeling. A vertebra which is more rigid due to intrassomatic filling with PMMA cement will change the usual spine balance concerning segmental stiffness and elasticity, which could give rise, in young patients, to accelerated discovertebral degeneration and even adjacent fractures. A different proposal is intra-stent application of biologically active and osteoconductive calcium phosphate cement, which is gradually reabsorbed and then substituted by bone. This does not occur with PMMA, which is organically inactive. That biological version of cement is gradually osteointegrated at the same time that the structure, consistency and height of the vertebral body, together with the calcium phosphate cement, are protected in a mechanical way by expandable intravertebral implants (2,3,6,14,19,26,27). Even so, the authors opt for the intrassomatic filling with bone graft, aiming at assuring a bone matrix that allows for osteoinduction as well as osteoconduction, this way facilitating healing and obtaining a vertebral body with biomechanics and morphology closer to the ones before the fracture, using a metallic interior endoskeleton filled with the integrated bone graft. Even though different authors have studied the isolated intrassomatic application of bone graft (with no intravertebral expandable implants) in fractures, graft resorption and a gradual flattening of those vertebrae was found. This possibly happens because of the mechanical support capacity held by the isolated bone graft is not enough. Therefore, the latter suffers excessive loads, which impairs its incorporation and integrity (4,19,28-31). Thus, we state that the application of bone graft inside the stents is crucial, as it allows for the maintenance of vertebral height and for the protection of the bone graft, reducing its resorption until it is incorporated. This way, a vertebra presenting a metallic endoskeleton, which is completely filled with bone, is obtained. The histological evidence of cases regarding isolated intrassomatic application of bone graft—with no intravertebral expandable implants—although limited, reflected, in several individuals, there were no intrassomatic graft incorporation and there were some microscopic findings of partial graft necrosis, even if there is bone healing clinical evidence and imaging. That probably means that there is likely too much load on the bone graft to be incorporated and which is that is not protected by intravertebral implants, as well as a not well-defined relation established between clinic and histology. Nevertheless, there is the need for long-term prospective studies so as to perceive the assets of the application of intrassomatic bone graft, along with intravertebral implants, in traumatic fractures (4,19,28-31). We chose the expansive intravertebral device VBS® for complete burst fracture as it consists of a reduction and space-filling device that is able to multidirectionally expand, in a centrifugal circumferential direction, both laterally and vertically, and originate two intrasomatic cavities, covered by a casing of impacted trabeculae and the implant metallic net. After being filled with bone graft or cement, they replace a considerable part of the vertebral body interior, which is then filled and stabilized. Furthermore, the cylindrical shaped mesh morphology of these implants permits to replace the damaged interior of the vertebral body more effectively and to fill it properly with bone graft. Moreover, we advocate that the stents’ considerable trabecular impaction surface over other expansive implants allow for a more effective direct reduction and stabilization in severe comminuted fractures, like complete burst type, as it impacts a greater amount of bone trabeculae around them, strengthening the vertebral body’s bone casing (7,12,13).
Conclusions
We present a detailed description and promising clinical and imaging results of a surgical technique of combined indirect and direct reduction, and vertebral body interior replacement with stents filled with bone graft. We regard it as a treatment that allows for a complete burst fracture to be anatomically restored by a posterior and minimally invasion approach. We also demonstrate a fast and full recovery after 3-year follow-up, with the patient maintaining his healed L1 native vertebral body with a similar anatomy and biomechanics to the original one, avoiding the morbidity and risk of serious complications of anterior approaches. Faced with a young and active patient and resorting to the techniques and rationale described, we usually prefer this posterior minimally invasive reconstruction of the vertebral body, leaving corpectomy and total vertebral body replacement for situations that demand anterior decompression of the vertebral canal. Nevertheless, long-term prospective studies are necessary so as to prove the effectiveness and assets of this surgical option versus other common techniques in complete burst fractures. Also, the relevance of intraosseous arterial injury in vertebral fracture prognosis in terms of healing remains to be proven and could be the focus of further studies.
Acknowledgments
Funding: None.
Footnote
Reporting Checklist: The authors have completed the CARE reporting checklist. Available at https://jss.amegroups.com/article/view/10.21037/jss-22-117/rc
Conflicts of Interest: Both authors have completed the ICMJE uniform disclosure form (available at https://jss.amegroups.com/article/view/10.21037/jss-22-117/coif). The authors have no conflicts of interest to declare.
Ethical Statement: The authors are accountable for all aspects of the work in ensuring that questions related to the accuracy or integrity of any part of the work are appropriately investigated and resolved. All procedures performed in this study were in accordance with the ethical standards of the institutional and/or national research committees and with the Helsinki Declaration (as revised in 2013). Written informed consent was obtained from the patient for publication of this case report and accompanying images. A copy of the written consent is available for review by the editorial office of this journal.
Open Access Statement: This is an Open Access article distributed in accordance with the Creative Commons Attribution-NonCommercial-NoDerivs 4.0 International License (CC BY-NC-ND 4.0), which permits the non-commercial replication and distribution of the article with the strict proviso that no changes or edits are made and the original work is properly cited (including links to both the formal publication through the relevant DOI and the license). See: https://creativecommons.org/licenses/by-nc-nd/4.0/.
References
- Krüger A, Schmuck M, Noriega DC, et al. Percutaneous Dorsal Instrumentation of Vertebral Burst Fractures: Value of Additional Percutaneous Intravertebral Reposition-Cadaver Study. Biomed Res Int 2015;2015:434873. [Crossref] [PubMed]
- Verlaan JJ, van Helden WH, Oner FC, et al. Balloon vertebroplasty with calcium phosphate cement augmentation for direct restoration of traumatic thoracolumbar vertebral fractures. Spine (Phila Pa 1976) 2002;27:543-8. [Crossref] [PubMed]
- Korovessis P, Repantis T, Petsinis G, et al. Direct reduction of thoracolumbar burst fractures by means of balloon kyphoplasty with calcium phosphate and stabilization with pedicle-screw instrumentation and fusion. Spine (Phila Pa 1976) 2008;33:E100-8. [Crossref] [PubMed]
- Daniaux H. Transpedicular repositioning and spongioplasty in fractures of the vertebral bodies of the lower thoracic and lumbar spine. Unfallchirurg 1986;89:197-213.
- Marco RA, Kushwaha VP. Thoracolumbar burst fractures treated with posterior decompression and pedicle screw instrumentation supplemented with balloon-assisted vertebroplasty and calcium phosphate reconstruction. J Bone Joint Surg Am 2009;91:20-8. [Crossref] [PubMed]
- Verlaan JJ, Somers I, Dhert WJ, et al. Clinical and radiological results 6 years after treatment of traumatic thoracolumbar burst fractures with pedicle screw instrumentation and balloon assisted endplate reduction. Spine J 2015;15:1172-8. [Crossref] [PubMed]
- Rotter R, Martin H, Fuerderer S, et al. Vertebral body stenting: a new method for vertebral augmentation versus kyphoplasty. Eur Spine J 2010;19:916-23. [Crossref] [PubMed]
- Baeesa SS, Krueger A, Aragón FA, et al. The efficacy of a percutaneous expandable titanium device in anatomical reduction of vertebral compression fractures of the thoracolumbar spine. Saudi Med J 2015;36:52-60. [Crossref] [PubMed]
- Noriega D, Marcia S, Theumann N, et al. A prospective, international, randomized, noninferiority study comparing an implantable titanium vertebral augmentation device versus balloon kyphoplasty in the reduction of vertebral compression fractures (SAKOS study). Spine J 2019;19:1782-95. [Crossref] [PubMed]
- Kerschbaumer G, Gaulin B, Ruatti S, et al. Clinical and radiological outcomes in thoracolumbar fractures using the SpineJack device. A prospective study of seventy-four patients with a two point three year mean of follow-up. Int Orthop 2019;43:2773-9. [Crossref] [PubMed]
- Verlaan JJ, van de Kraats EB, Oner FC, et al. The reduction of endplate fractures during balloon vertebroplasty: a detailed radiological analysis of the treatment of burst fractures using pedicle screws, balloon vertebroplasty, and calcium phosphate cement. Spine (Phila Pa 1976) 2005;30:1840-5. [Crossref] [PubMed]
- Moura DL, Gabriel JP. Expandable Intravertebral Implants: A Narrative Review on the Concept, Biomechanics, and Outcomes in Traumatology. Cureus 2021;13:e17795. [Crossref] [PubMed]
- Moura DFL, Gabriel JP. Intravertebral expandable implants in thoracolumbar vertebral compression fractures. Acta Ortop Bras 2022;30:e245117. [Crossref] [PubMed]
- Maestretti G, Sutter P, Monnard E, et al. A prospective study of percutaneous balloon kyphoplasty with calcium phosphate cement in traumatic vertebral fractures: 10-year results. Eur Spine J 2014;23:1354-60. [Crossref] [PubMed]
- Freyd M. The graphic rating scale. J Educ Psychol 1923;43:83-102.
- Fairbank JC, Pynsent PB. The Oswestry Disability Index. Spine (Phila Pa 1976) 2000;25:2940-52; discussion 2952. [Crossref] [PubMed]
- Harrington RM, Budorick T, Hoyt J, et al. Biomechanics of indirect reduction of bone retropulsed into the spinal canal in vertebral fracture. Spine (Phila Pa 1976) 1993;18:692-9. [Crossref] [PubMed]
- Oner FC, van der Rijt RR, Ramos LM, et al. Changes in the disc space after fractures of the thoracolumbar spine. J Bone Joint Surg Br 1998;80:833-9. [Crossref] [PubMed]
- Crawford RJ, Askin GN. Fixation of thoracolumbar fractures with the Dick fixator: the influence of transpedicular bone grafting. Eur Spine J 1994;3:45-51. [Crossref] [PubMed]
- Noriega DC, Marcia S, Ardura F, et al. Diffusion-Weighted MRI Assessment of Adjacent Disc Degeneration After Thoracolumbar Vertebral Fractures. Cardiovasc Intervent Radiol 2016;39:1306-14. [Crossref] [PubMed]
- Vaccaro AR, Oner C, Kepler CK, et al. AOSpine thoracolumbar spine injury classification system: fracture description, neurological status, and key modifiers. Spine (Phila Pa 1976) 2013;38:2028-37. [Crossref] [PubMed]
- Young WF, Brown D, Kendler A, et al. Delayed post-traumatic osteonecrosis of a vertebral body (Kummell's disease). Acta Orthop Belg 2002;68:13-9.
- Braunstein V, Sprecher CM, Gisep A, et al. Long-term reaction to bone cement in osteoporotic bone: new bone formation in vertebral bodies after vertebroplasty. J Anat 2008;212:697-701. [Crossref] [PubMed]
- Zhang C, Ouyang B, Li P, et al. A Retrospective Study of Thoracolumbar Fractures Treated with Fixation and Nonfusion Surgery of Intravertebral Bone Graft Assisted with Balloon Kyphoplasty. World Neurosurg 2017;108:798-806. [Crossref] [PubMed]
- Togawa D, Bauer TW, Lieberman IH, et al. Histologic evaluation of human vertebral bodies after vertebral augmentation with polymethyl methacrylate. Spine (Phila Pa 1976) 2003;28:1521-7.
- Korovessis P, Hadjipavlou A, Repantis T. Minimal invasive short posterior instrumentation plus balloon kyphoplasty with calcium phosphate for burst and severe compression lumbar fractures. Spine (Phila Pa 1976) 2008;33:658-67. [Crossref] [PubMed]
- Verlaan JJ, Oner FC, Slootweg PJ, et al. Histologic changes after vertebroplasty. J Bone Joint Surg Am 2004;86:1230-8. [Crossref] [PubMed]
- Van Herck B, Leirs G, Van Loon J. Transpedicular bone grafting as a supplement to posterior pedicle screw instrumentation in thoracolumbar burst fractures. Acta Orthop Belg 2009;75:815-21.
- Alanay A, Acaroglu E, Yazici M, et al. Short-segment pedicle instrumentation of thoracolumbar burst fractures: does transpedicular intracorporeal grafting prevent early failure? Spine (Phila Pa 1976) 2001;26:213-7. [Crossref] [PubMed]
- Knop C, Fabian HF, Bastian L, et al. Late results of thoracolumbar fractures after posterior instrumentation and transpedicular bone grafting. Spine (Phila Pa 1976) 2001;26:88-99. [Crossref] [PubMed]
- Tägil M, Johnsson R, Strömqvist B, Aspenberg P. Incomplete incorporation of morselized and impacted autologous bone graft: a histological study in 4 intracorporally grafted lumbar fractures. Acta Orthop Scand 1999;70:555-8. [Crossref] [PubMed]