Patient-specific spinal rods in adult spinal deformity surgery reduce proximal junctional failure: a review of patient outcomes and surgical technique in a prospective observational cohort
Highlight box
Key findings
• Patient-specific spinal rod (PSSR) is a useful option to improve patient reported outcome measures (PROMs) in adult spinal deformity (ASD).
• Distal junctional failure (DJF) was related to absence of interbody cages at the lumbosacral junction.
• PSSR demonstrated only partial correction of sagittal parameters at final follow-up.
What is known and what is new?
• PSSR is a novel option in management of ASD providing an intraoperative deformity correction consistent with the surgical plan.
• This article adds critical patient data to the paucity in the literature. Most notably reporting on DJF/proximal junctional failure/proximal junctional kyphosis, sagittal parameters, and PROMs.
What is the implication, and what should change now?
• Consider the use of surgical specific software as a useful adjunct in planning major deformity correction.
• Consider implications (distal failure) of a wide-based cage at the lumbosacral junction in major deformity surgery.
Introduction
Globally, low back pain is the leading cause of years lived with disability (1). Adult spinal deformity (ASD) is estimated to affect up to 68% of people over the age of 65 years. These deformities may lead to pain, disability, and neurological deficit (2). After failure of non-operative management of ASD, surgical intervention is widely accepted to correct the spinal deformity and improve quality of life (2). Spinal rods used for thoracolumbar posterior fixation are usually manufactured straight and bent manually during surgery. This manual bending can cause under- or over-correction of the deformity or rod breakage (3). Traditionally major deformity surgery is associated with extended operative time, higher blood loss, prolonged recovery, and up to one-fifth of patients reporting decisional regret for undergoing the index procedure (4,5). Pre-bent patient-specific spinal rods (PSSRs) have been developed with software allowing preoperative deformity correction planning and providing the surgeon with an intraoperative deformity correction consistent with the surgical plan.
Notably, there are reduced operation times because PSSR do not require contouring during surgery which results in fewer rod microfractures, decreased fatigue-life and fewer mechanical complications (5-7). Several literature articles, lacking a generalized consensus, report their varying outcomes on sagittal parameters and patient reported outcome measures (PROMs) following PSSR surgery (3,8-12). Spinopelvic sagittal parameters, as defined by Schwab, have been widely accepted targets for ASD surgical correction (13).
Important considerations in ASD surgery are patient comorbidities, postoperative complications, and economic impact (2,14,15). New technology with PSSR aims to provide patient orientated treatment with improved patient understanding, consent and outcomes. With long-term follow-up, systematic reviews and observational studies demonstrate over half (56%) of patients suffer proximal junctional kyphosis (PJK). Proximal junctional failure (PJF) requiring surgery is substantially more common (35%) than distal junctional failure (DJF) (4%) (15-20). However, whilst PJK and PJF have been widely reported, DJF has been less studied (17).
The aim of this study was to report patient satisfaction and clinical and radiological outcomes using PSSR, comparing preoperative planning to postoperative correction and maintenance of the sagittal parameters to mid-term follow-up. Specifically, we investigated rates of junctional complications both proximally (kyphosis/failure) and distally (failure). We present this article in accordance with the STROBE reporting checklist (available at https://jss.amegroups.com/article/view/10.21037/jss-23-85/rc).
Methods
Data collection
Prospective study of all consecutive patients who underwent ASD surgery with PSSR at a single institution between Jan 2019 and December 2022. Inclusion criteria were patients with PSSR surgery at ≥4 levels and thoracolumbar deformity according to the Schwab criteria (13). Patients who were current smokers were excluded from this study. Patients who were diagnosed with low bone mineral density were referred to consultant endocrinologist to optimize bone health before surgery; these patients were included for surgery.
Patient demographics were recorded, including comorbidities of body mass index (BMI) and diabetes status. PROMs included visual analogue scale (VAS) back/leg pain, Oswestry disability index (ODI) and quality of life assessed by the 12-Item Short Form Survey (SF-12) recorded preoperatively, at 6 weeks, 6 months, 12 months, 24 months and final follow-up postoperatively.
Operative data included operation time, blood loss, surgical approach, levels, single or staged approaches and complications. Complications reported by independent consultant physicians, were categorised into perioperative (≤3 months postoperatively) and late (>3 months postoperatively) consistent with Zanirato et al. [2018] (15).
We assessed patient satisfaction with the Ottawa decision regret questionnaire (4). This is a validated 5-item scale where patients indicated their regret/satisfaction by indicating a number from 1 (strongly agree) to 5 (strongly disagree) converted to a 0–100 scale. A score below 40 indicates low decisional regret and a score above 60 indicates high decisional regret.
Surgical technique
The PSSRs were manufactured to a planned sagittal and coronal deformity correction made between the patient, surgeon, and surgical specific machine learning software (UNiD™ Adaptive Spine Intelligence, Medtronic, MN, USA). The patient was consented appropriately for risks, benefits, and length of procedure. The spinal sagittal shape was characterised by preoperative electro optical system (EOS) scans which was analysed by specific software.
A PSSR template was used for intraoperative patient positioning. The number of anterior and posterior operative and interbody fusion levels were dependent upon and unique to the patient-specific surgical plan. Not all patients had an interbody cage placed. All PSSRs were used without modification to shape. All posterior pedicle screws (PASS LP pedicle screw fixation system, Medtronic, MN, USA) were placed by open technique aided by computer-guided navigation. Single stage procedures involved posterior/transforaminal lumbar interbody fusions (P/TLIF) at the lumbosacral junction then Smith-Peterson posterior column osteotomies (PCOs) with posterior fixation. Posterolateral and interbody fusion were optimized using recombinant human bone morphogenetic protein-2 (rhBMP-2), allograft, and synthetic bone graft. Staged approaches used first-stage anterior column interbody fusion with lateral lumbar interbody fusions (LLIF) at the coronal curve apex of the deformity and/or anterior lumbar interbody fusions (ALIF) at L5/S1 +/− L4/5 prior to second-stage posterior decompression and instrumented fusions. Anterior column interbody cages (ALIF/LLIF) were considered wide footprint and PLIF/TLIF were considered narrow footprint cages. Pelvic fixation was undertaken with S2AI screws. No junctional tethers/ligament or cement augmentation was used proximally.
Radiographic analysis
Preoperative radiological investigations included dynamic X-rays, computed tomography (CT), magnetic resonance imaging (MRI), bone density scan (DEXA), and nuclear medicine bone scans. If investigations were not considered clinically relevant, they were not undertaken. Patients at risk of reduced bone density were screened with DEXA scans as per Royal Australasian College of General Practitioners (RACGP) guidelines. Sagittal spinopelvic parameters measured by EOS scans were performed preoperatively then compared to planned correction and postoperative measures as reported by independent radiologists.
Radiological parameters included sagittal vertical axis (SVA), pelvic tilt (PT), pelvic incidence (PI), lumbar lordosis (LL), and mismatch between pelvic incidence and lumbar lordosis (PI-LL) (2). We used the Schwab classification system of ASD to assess the sagittal modifiers, noting postoperative sagittal parameter ideal targets suggested by the literature as SVA <50 mm, PT <20°, and PI-LL <±10° (13). However, the PSSR software modeling used in this study has patient specific planned targets. We recognise thoracic kyphosis as an important sagittal parameter but did not analyse it in this review (21).
Radiographic PJK was defined as a proximal kyphotic angle (PKA) ≥10° and change of 10° degrees greater than the preoperative measurement of the PKA (20). The PKA was a Cobb Angle measurement between the lower endplate of the upper instrumented vertebrae (UIV) and the superior endplate of the vertebrae two levels above the UIV. We defined PJF and DJF as a patient requiring revision surgery (19).
Ethical statement
Institutional approval was granted by Epworth HealthCare (No. EH2020-515). This study was conducted in accordance with the Declaration of Helsinki (as revised in 2013) and The National Statement on Ethical Conduct in Human Research [2023]. This was a review of cases collected under a standard privacy disclosure to patients that their information will be used for ongoing evaluation of outcomes and their identity will be protected in any publication arising from this. Verbal informed consent was obtained at last follow-up.
Statistical analysis
Counts and percentages were reported for categorical data, means and standard deviations for continuous data. If the latter appeared to be skewed, or ordinal, medians and IQRs (interquartile ranges, difference between the 25th and 75th percentiles) were reported. Linear mixed model analysis (22) was used to compare PROMs across time overall, and specifically between baseline and final follow-up, this being the a priori or planned pairwise comparison of greatest clinical interest. Linear mixed model analysis was also used to compare SVA, PT and PI-LL between pre-test and plan, and between plan and post-test, these being the planned pairwise comparisons of greatest clinical interest. Independent samples t-tests were used to assess differences in patients who suffered PJK and associated PJK risk score. Statistical significance was assessed at P<0.05, 2-tailed. Analyses were performed in Stata 17, Stata Corporation, College Station, Texas, 2021.
Results
Twenty patients underwent PSSR within the study period, had a mean age at operation of 71.9 (±6.9, standard deviation) years, 15 (75%) were female, with an overall mean BMI of 27.8 (±4.8) kg/m2. Follow-up time ranged from 7 to 40 months (mean 25.2±8.6). Six patients were ex-smokers. Five patients had osteoporosis (T score <−2.5) and 4 had osteopenia. Patient demographics are displayed in Table 1.
Table 1
Final follow-up demographics | Statistical results |
---|---|
Female, n [%] | 15 [75] |
Age (years), mean (SD) | 71.9 (6.9) |
Follow-up time (months), mean (SD) | 25.2 (8.6) |
BMI (kg/m2), mean (SD) | 27.8 (4.8) |
Ex-smokers, n [%] | 6 [30] |
Osteoporosis/osteopenia, n [%] | 5 [25]/4 [20] |
Previous spine surgery, n [%] | 12 [60] |
Comorbidities, n [%] | |
Cardiovascular disease | 14 [70] |
DVT/PE | 6 [30] |
Asthma | 5 [25] |
Osteoarthritis | 5 [25] |
Hypothyroidism | 4 [20] |
T2DM | 3 [15] |
Depression | 3 [15] |
Anxiety | 1 [5] |
SD, standard deviation; BMI, body mass index; DVT, deep vein thrombosis; PE, pulmonary embolism; T2DM, type-2 diabetes mellitus.
Mean number of operative levels was 9.6 (±3.2). Sixteen of 20 patients had distal pelvic fixation (S2AI), 1 had fixation to S1 and 3 had lower instrumented vertebra (LIV) at L5. UIV were T4 [1], T6 [2], T8 [2], T10 [9], T11 [1], L1 [3], L2 [2]. PSSRs were used without further bending modifications of the rod shape except distal trimming. Supplemental interbody cages were placed T12/L1 to L5/S1 (Table 2). Interbody cages from previous surgeries were revised or remained according to surgical plan. Fifteen (75%) out of 20 operations were two-stage approaches. All surgeries used titanium 5.5 mm rods. Including both 1- and 2-stage surgeries median blood loss was 800 mL (IQR: 1,000 mL) and mean operation time was 590 (±165) min. The two-stage group mean operation time (659.1±155.2 min) was significantly higher than that for the one stage group mean (481.5±121.1 min), [t(26): −2.6, mean difference: 177.6, 95% confidence interval (CI): 30.7 to 324.5, P=0.021].
Table 2
Variables | Operative values |
---|---|
Estimated blood loss (mL), median (IQR) | 800 (1,000) |
Operation time (min), mean (SD) | 590 (165) |
Operative interbody fusion level, n [%] | |
T12/L1 | 2 [10] |
L1/L2 | 4 [20] |
L2/L3 | 5 [25] |
L3/L4 | 3 [15] |
L4/L5 | 7 [35] |
L5/S1 | 9 [45] |
Bone graft, n [%] | |
BMP-2 | 9 [45] |
Allograft | 10 [50] |
Synthetic | 1 [5] |
IQR, interquartile range; SD, standard deviation; BMP-2, bone-morphogenic protein-2.
As seen in Table 3 and Figure 1, using a linear mixed model regression, we found a statistically significant overall improvement between five time points (baseline, 6-week, 6-month, 12-month, and 24-month) (P value <0.001) for mean VAS Back, VAS Leg, ODI, and SF-12 (physical/mental). Linear mixed models revealed a statistically significant improvement in all PROMs between the paired comparison of interest time points from baseline to 12-month (Table 3).
Table 3
PROMs | Baseline, mean (95% CI) |
6-week, mean (95% CI) |
6-month, mean (95% CI) |
12-month, mean (95% CI) |
24-month, mean (95% CI) |
Paired comparison of interest (baseline to 12-month) | |
---|---|---|---|---|---|---|---|
Mean (95% CI) | P | ||||||
VAS Back | 8.6 (7.6–9.5) | 5.9 (4.9–6.8) | 4.4 (3.4–5.4) | 3.5 (2.5–4.6) | 2.7 (1.6–3.8) | −5.1 (−6.3 to −3.9) | <0.001 |
VAS Leg | 6.1 (4.9–7.2) | 4.0 (2.9–5.1) | 2.8 (1.6–4.0) | 2.3 (1.1–3.5) | 1.4 (0.1–2.8) | −3.7 (−5.5 to −1.9) | <0.001 |
ODI | 35.8 (32.9–38.6) | 28.3 (25.4–31.1) | 25.6 (22.6–28.6) | 20.2 (17.1–23.2) | 15.4 (12.0–18.8) | −15.9 (−19.4 to −12.3) | <0.001 |
SF-12 Physical | 25.4 (22.6–28.2) | 34.1 (31.3–36.9) | 36.6 (33.7–39.5) | 42.1 (39.1–45.1) | 44.8 (41.5–48.1) | 17.0 (14.1 to 20.0) | <0.001 |
SF-12 Mental | 41.0 (37.5–44.6) | 48.4 (44.9–51.9) | 52.0 (48.3–55.6) | 54.6 (50.9–58.3) | 53.6 (49.6–57.5) | 14.0 (9.8 to 18.2) | <0.001 |
PROM, patient reported outcome measure; CI, confidence interval; VAS, visual analogue scale; ODI, Oswestry disability index; SF-12,
12-item Short Form Survey.
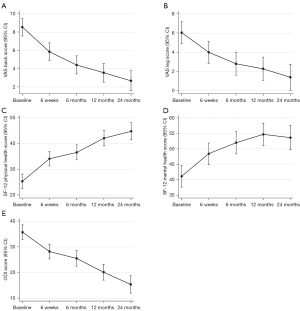
Perioperative (≤3 months) and late (>3 months) complications are reported in Table 4. Four patients (20%) reported decisional regret to undergo the PSSR surgery with a score of more than 60, they all suffered DJF. The remaining patients reported low (less than or equal to 60) regret scores. One patient suffered both DJF and PJF. No rod breakages were observed.
Table 4
Complication | Acute, n [%] | Chronic, n [%] |
---|---|---|
Hypotension | 8 [40] | 0 |
Anemia | 7 [35] | 0 |
Pleural effusion | 4 [20] | 0 |
Delirium | 2 [10] | 0 |
Fluid overload | 2 [10] | 0 |
Deranged LFTs | 2 [10] | 0 |
Pneumothorax | 2 [10] | 0 |
Fibular fracture | 1 [5] | 0 |
Hyponatremia | 1 [5] | 0 |
Cerebral oedema | 1 [5] | 0 |
Constipation | 1 [5] | 0 |
DJF | 0 | 4 [20] |
PJF | 0 | 1 [5] |
LFT, liver function test; DJF, distal junctional failure; PJF, proximal junctional failure.
One patient (5%) exhibited PJF: a 60-year-old woman with osteopaenia suffered continued deformity 2 years postoperatively (Figure S1). EOS scans indicated kyphosis and truncal shift to the right and CT scans indicated a PJF with wedging deformity of T7–T10. The surgeons recognise that the original PSSR plan placed the UIV below the apex of the thoracic curve. We acknowledge this was an error in surgical planning. The patient underwent proximal extensions to T5.
Forty percent demonstrated PJK with a mean PKA of 17.8±13.0 degrees. Our cohort had an overall mean PJK risk score, as defined in Table 5, of 3.7±1.0. We found no difference in PJK risk score [t(14): −1.2, P=0.258, 95% CI: 0.5–1.6] comparing patients without PJK (mean 3.4±1.0) and patients with PJK (mean 4±0.8).
Table 5
PJK risk score | Age, years | LIV position | UIV position | Correction |
---|---|---|---|---|
0 | ≤55 | Above S1 | Lumbar spine | Lordotic change ≤10° |
1 | >55 | At S1 or iliac | Upper thoracic (T1–T6) | Lordotic change >10° |
2 | NA | NA | Lower thoracic (T7–T12) | NA |
PJK, proximal junction kyphosis; LIV, lower instrumented vertebra; UIV, upper instrumented vertebra.
Four patients suffered DJF needing revision surgery (3/4 were fused to pelvis). Of these, three patients had no L5/S1 interbody cages inserted and one patient had a single TLIF cage at L5/S1. No patients suffered DJF with wide footprint cages at L5/S1 (14/16 were fused to pelvis).
There was a statistically significant difference in SVA (mean difference: −65.1, 95% CI: −86.0 to −44.1, P<0.001) and PI-LL (mean difference: −15.0, 95% CI: −21.9 to −8.2, P<0.001) between preoperative and surgical plan means. PT preoperative versus surgical plan narrowly failed to achieve statistical significance (mean difference: −2.5, 95% CI: −5.0 to 0.1, P=0.058). There was a statistically significant difference in SVA (mean difference: 52.0, 95% CI: 31.4 to 72.5, P<0.001), PI-LL (mean difference: 11.8, 95% CI: −5.6 to 18.1, P<0.001) and PT (mean difference: 3.6, 95% CI: 0.1 to 7.1, P=0.045) between the surgical plan and postoperative means (Table 6, Figure 2). The small but accurate differences between the values in Table 6 and the corresponding text are due to the Mixed Models statistical method used. At final follow-up of the entire cohort, ideal SVA (40%), PT (45%), PI-LL (35%) were achieved.
Table 6
Radiographic parameters | Preoperative | Plan | Postoperative |
---|---|---|---|
SVA | 97.3 (74.4–120.1) | 30.4 (6.7–54.1) | 79.3 (56.1–102.6) |
PT | 26.2 (22.6–29.7) | 23.5 (19.8–27.2) | 27.1 (23.5–30.6) |
PI-LL | 19.9 (12.7–27.1) | 4.4 (–3.1–11.8) | 15.7 (8.5–22.9) |
Data were presented as mean (95% confidence interval). SVA, sagittal vertical axis; PT, pelvic tilt; PI, pelvic incidence; LL, lumbar lordosis.
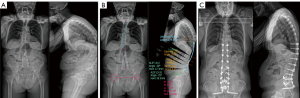
Discussion
Years lived with disability due to low back pain is higher in women, consistent with the majority (75%) of our cohort being female (1). Recent literature has indicated that the use of templates with surgical specific software modelling and PSSR decreases overbending of rods and leads to improved patient outcomes (5,24). We recognise that surgical specific software modelling is a useful adjunct and guide in surgical management of ASD. Each patient has their own spinal balance which must be understood and measured before surgery. It is no longer acceptable to entrust the surgeon’s intuition alone with bending rods in the sagittal plane. However, it is not an absolute and the surgeon must follow established deformity principles and techniques that they are experienced and competent in performing.
Sagittal parameters
Pain and disability in the setting of ASD have been correlated with global coronal alignment, SVA, PT, and PI-LL. One of the main goals of surgery for ASD is to correct these parameters to restore harmonious sagittal alignment to prevent mechanical complications (2,25).
Over 24-month mean follow-up, our study showed that SVA (−18.0 mm) and PI-LL (−4.2 degrees) [but not PT (+0.9 degrees)] sagittal parameters using PSSR improved from baseline. Shorter follow-up studies had similar numbers of PSSR patients: Sadrameli et al. [2020] (19 patients over 16 months) and Barton et al. [2016] (18 patients over one month) (8,11). Our study showed a significant difference (P<0.001) between the preoperative film and surgical plan in SVA and PI-LL consistent with Sadrameli et al. [2020] and Barton et al. [2016]. However, we did not find a difference in PT between preoperative versus surgical plan, although the result narrowly failed to approach statistical significance (P=0.058). Our cohort showed a statistically significant difference between the surgical plan and postoperative achieved sagittal parameters [SVA (P<0.001), PI-LL (P<0.001), PT (P=0.045)] which contrasted to the non-significant difference reported by these 2 publications (P>0.05, suggesting the authors were able to implement the planned sagittal correction).
Prost et al. [2020] highlighted that 52 of 86 patients remained mal-aligned in the sagittal plane at 1-year postoperatively (10). Comparable findings were reported by Kleck et al. [2020], with a significant mean difference in postoperative versus preoperative SVA (−57 mm), PT (−1.9 degrees), PI-LL (−14 degrees) in 14 patients to 24 months follow-up (9). Our series of 20 patients with a similar mean follow-up did not find the same improvements in SVA, PT, and PI-LL postoperatively. Less than half of our patients were aligned in SVA (40%), PT (45%), and PI-LL (35%) at final-follow up.
Solla et al. [2019] followed 60 patients over 12-month finding significant improvements of PI-LL postoperatively but none in SVA and PT. PI-LL was <10 degrees at baseline in 29/60 patients and improved to 50/60 patients at follow-up (12). Similarly, Prost et al. [2020] followed 43 patients having PSSR over 3 months with PT not significantly corrected postoperatively; however, there was a statistically significant decrease in SVA (46%) and PI-LL (60%) at follow-up (10). Our longer follow-up contrasts with these findings by both Solla et al. [2019] and Prost et al. [2020]. We did not find a significant improvement between plan-postoperative SVA, PT, and PI-LL. Despite this our patients did report high satisfaction and statistically significant improvement in PROMs over the follow-up.
With evidenced-based patient-specific care, the traditional Schwab criteria defining ASD (in 50–60-year-olds) is limited in applicability to both older and younger age groups (26). We recognise that PJF and PJK are higher as patient’s age, leading to the development of age-adjusted spinopelvic parameters to attempt to reduce the rate of these complications by Lafage et al. [2017] (27). The applicability of these parameters is still used varyingly in the literature (21).
Scheer et al. [2018] found that only 33% of patients matched their age-adjusted spinopelvic thresholds (28). The remaining were over- or under-corrected. Our findings concur with only 40%, 45%, and 35% correction of spinopelvic SVA, PT, and PI-LL achieved in our series respectively. This appeared satisfactory for patients due to improved PROMs and high patient satisfaction to 2 years follow-up.
Patient reported outcomes
Our study reported a statistically significant overall improvement in PROMs between at all time points and a statistically significant improvement comparing baseline to 1 year follow-up. This demonstrates PSSR as a useful management option for ASD. Adogwa et al. [2022] performed a manual chart review on 91 patients undergoing ASD surgery with a final follow-up of 12 months (4). They reported that 1/5 patients regret the decision for ASD surgery based on the Ottawa decision regret questionnaire. In the low decisional regret group (scores <40), the mean baseline ODI (42.2), VAS Back (6.5), and VAS Leg (5.2) showed improvement to 12 months follow-up with scores of ODI (29.3), VAS Back (4.0), and VAS Leg (3.0). The high regret group (scores >60) showed no statistical difference to the low regret group at 12-month in ODI, VAS Back, and VAS Leg (4). This demonstrated that the decisional regret score is not correlated with PROMs. These results are similar to our findings of significant improvement at 24 months of ODI (15.4), VAS Back (2.7), and VAS Leg (1.4) with the same rate of 1/5 patients reporting high regret scores. We suggest that the decisional regret in our cohort is due to suffering from DJF.
The authors note the paucity of PROMs and patient satisfaction data in the literature surrounding the use of PSSR in management of ASD. To our knowledge, we are the first paper to report on PROMs and patient satisfaction in the postoperative follow-up of patients who have undergone ASD surgery with PSSR.
Surgical technique
The literature reporting on PSSR focuses on sagittal alignment goals and the utility of PCOs, however, often lacks discussion on the intricacies of surgical technique (3,8-12). McDonnell et al. [2021] stated that anterior-posterior (versus posterior-only) surgery, fusion to the sacrum, and younger age (<60 years) were protective of DJF with conventional rod use. The need for an interbody fusion at L5/S1 is controversial with conflicting reports about the benefit and complication rate (17). In our series, all patients who suffered DJF reported high decisional regret. We attribute this decisional regret to the development of DJF and the associated disability and pain.
We found impaction of a wide footprint interbody cage at L4/5 (ALIF, PLIF, or LLIF) or L5/S1 (ALIF or PLIF) lowered DJF rates compared to no cage or a single TLIF cage. This is consistent with findings from Eastlack et al. [2020] who highlighted that the use of an ALIF at L5/S1 is protective of lumbosacral junction failure compared to use of TLIF or no interbody cage (29). Likewise, Park et al. [2021] found ALIF superior to TLIF at the lumbosacral junction (30). Charles et al. [2020] suggested anterior fusion combined with posterior instrumentation was protective against non-union and DJF (31). Whilst this literature does not report DJF rates with PSSR we believe that restoration of lordosis and the additional stability from a wider footprint cage at the junction protects against DJF (32). We note all patients who did not have a wide footprint cage placed at L4/5 and/or L5/S1 reported high regret scores. This difference reflects better outcomes with cages at L4/5, L5/S1 or both.
The addition of multi-level LLIF in severe coronal deformities provides better correction of Cobb angles and SVA than posterior-only operations (33,34). We concurred with these International Spine Study Group findings that LLIF is a powerful first-stage correction of major thoracolumbar coronal apex deformities.
Seventy-five percent of our patients (15/20) underwent 2-stage surgeries with a first-stage interbody fusion/s then second-stage posterior osteotomies and instrumented fusions. In ASD surgery PCOs have been validated to reduced PJF rates with conventional rods and achieve better alignment of sagittal parameters with PSSR, which is consistent in our study with all patients having PCOs (10,12,35). Recent publications also report lower revisions and improved clinical/radiological outcomes in two stage surgery (36,37). We did not compare differences in outcomes between 1- and 2-stage operations.
Operation time was consistent across our cohort with a mean of 590±165 min and a median estimated blood loss of 800 mL (IQR: 1,000 mL). Using PSSR our operative time did not decrease, and the EBL was reduced. The total operation time of our cohort was more but EBL less than the results reported in a recent systematic review of a mean operation time of 370 (±161) min and mean EBL of 1,828 (±957) mL (15). Similarly, using PSSR Sadrameli et al. [2020] reported an operation time of 411 (±93) min with EBL of 861 (±354) mL (11). It is important to highlight the increased operation time with an accepted EBL, whilst different from the current literature, it notes a possible barrier in implementation of PSSR. The surgeons believe the time saving from eliminating the need for rod bending was counteracted by the need for increased time whilst performing PCOs to fit the PSSR.
Systematic reviews of ASD surgery report major complication rates of deep wound infections (2.2%) and new neurological deficits (2.3%) (15); however, our study found none. Our results remain unclear to whether the implementation of PSSR would influence these complication rates. Excessive blood loss (>2,000 mL) in our series occurred in only one patient, and this did not correlate negatively with poorer outcomes or regret score. Perioperative cases of anaemia, hypotension, pleural effusion, delirium, deranged liver function tests were comparatively higher than the literature (15), reflecting independent physician care of our patients. Other rates of perioperative complications were consistent with the literature.
In our study, all posterior pedicle screws were placed open and not by percutaneous technique. This optimized the fusion bed for posterolateral bone grafting. Pedicle screws were inserted with the aid of computer-guided navigation to optimize screw placement accuracy. Pelvic fixation was undertaken with S2AI screws to ensure coronal alignment of the S2AI screws with the pre-contoured PSSR, obviating the need for connecting rods to more laterally placed iliac screws. Sacroiliac joint (SIJ) fusions in this study were achieved concurrently with S2AI screws and no supplementary impacted device, bone graft, or screws. Posterolateral and interbody fusion was optimized using rhBMP-2, allograft, and one case of synthetic graft.
Rod materials
Our series reported the use of 20 patients with titanium (Ti) PSSR, all with a diameter of 5.5 mm. Currently, four rod materials are used in ASD surgery; stainless steel (SS), Ti, cobalt chromium (CoCr), and ultrahigh strength stainless steel (UHSS) (38). Serhan et al. [2013] show that the correctional force produced by a Ti 30-degree pre-bent rod was approximately 67% than that of CoCr and UHSS rods. However, the Ti rod was best (90%) at maintaining its original shape. This highlights titanium’s resistance to deforming despite the superior corrective ability of CoCr and UHSS rods (38). Shega et al. [2020] systematically reviewed 11 studies comparing Ti against CoCr rods (39). They found the CoCr was better than the Ti rod for effective correction of spinal deformity and postoperative stability of the spine. Moreover, incidence of rod fracture was higher in titanium rods but PJK occurred more with CoCr. Shega et al. [2020] did not report on the efficacy of Ti in PSSR surgery. However, Bowden et al. [2022] performed a systematic review and found no differences in postoperative outcomes or complications when using different rod materials or diameters (40). Our experience with PSSR is limited to Ti only.
Proximal and distal junctional complications
Late complications experienced were DJF [4/20 (20%)], PJK [8/20 (40%)], PJF [1/20 (5%)]. This is higher than the reported DJF rate of 3.6% but less than the reported PJF rate of up to 35% (17,19). Our cohorts PJK rate was less than findings that over half of ASD surgical patients develop PJK (20). An increasing PJK leads to a positive sagittal balance highlighted by Glassman et al. [2005] which leads to an increase in symptoms of ASD (41).
Significant risk factors for development of PJK in ASD surgery (without PSSR use) have been identified and divided into three categories (radiological, surgical and patient-related) (42-44). Radiological parameters include maligned preoperative and postoperative sagittal parameters. Surgical risk factors consist of disruption of: (I) posterior longitudinal ligament; (II) posterior tension band (supraspinous/interspinous ligaments); (III) the paraspinal musculature at the UIV; (IV) facet joint violation; (V) compression fracture at the most instrumented vertebra; and (VI) instrumentation failure at the proximal construct. Thirdly, patient-related factors include poor bone quality, high frailty, high BMI, and increased age. Ideally, we would analyse the impact of these factors on PJK in our own cohort. However, our small sample size limits the ability to do so without additional errors. Hence, we used Lafage et al. [2020] PJK risk scoring system which includes 5 of the aforementioned risk factors but not all of them (23).
Lafage et al. [2020] developed a simple risk scoring system for PJK at 2 years follow-up (23). The authors used 5 factors corresponding to a 6-scale risk score (Table 5). The factors were defined as: (I) age >55 years, (II) fusion including S1/ilium, (III) UIV in upper thoracic spine (T1-T6), (IV) UIV in lower thoracic spine (T7–T12), and (V) >10° of lordotic correction. A score of 5 corresponded to an increased odds of 11.0 and a score of 4 corresponded to 5.3 increased odds of developing PJK. We report a mean PJK risk score of 3.7±1.0 and found no significance between the PJK risk score and development of PJK (P=0.258). Our non-significant results suggest that our cohort does not align with the PJK risk score previously validated by Lafage et al. [2020] (23). But we think it pertinent to investigate these results in the future and consider analysing the effects of each separate factor especially the position of the UIV.
Strengths and limitations
The strengths of this study include a prospective study design that assesses PROMs, radiographic sagittal parameters, and operative outcomes. Patients with reduced bone density were assessed preoperatively by a consultant endocrinologist. Most patients had 24-month EOS imaging with parameters assessed and measured by independent radiologists. This allowed distal and proximal evaluation of the construct.
The literature reporting ASD most commonly uses ODI and SRS-22 to assess PROMs. Other PROMs include HRQoL indices and the ASD-Frailty Index. These have been shown to predict surgical outcomes in ASD surgery (45,46). However, a systematic review conducted by Archer et al. [2022] indicated there was insufficient evidence to appropriately assess the measurement properties of PROMs in adult scoliosis surgery (47). This may explain the inconsistency in our favourable PROMs and less than ideal sagittal parameters postoperatively. Future studies should consider the limitations while using these validated PROMs.
This study represents the experience of a single surgeon past their learning curve of conventional ASD surgery with over 100 cases experience and a well-developed, systematic, and safe approach to the operation (48). Several limitations are present in this non-randomized, observational single-centre case series. The small cohort limits extrapolation and inclusion of potential confounders. However, this series presents a patient sample with average of over 2 years follow-up. Larger multi-centre studies are planned to investigate further the utility of PSSR.
Conclusions
In this series, PSSR improved PROMs and treated ASD to a mean of 25 months. Sagittal parameters planned preoperatively correlated with postoperative correction but demonstrated partial loss with PJK over time. However, PJF was reduced and there were no rod breakages. Decisional regret was reported in patients who suffered DJF and required reoperation. All other patients were satisfied with their surgical outcomes. PSSR is a useful option for patients unable to tolerate major anterior and posterior reconstructive surgery. We found DJF occurred with PSSR but was related to the absence of interbody cages at the lumbosacral junction.
Acknowledgments
We wish to thank Dr Christopher J. Kleck (Department of Orthopedics, University of Colorado, Anschutz Medical Campus, Aurora, CO, USA) and Dr Michael A. Johnson (Spine Society of Australia, North Sydney, NSW, Australia) for reviewing and advice on this manuscript.
Funding: None.
Footnote
Reporting Checklist: The authors have completed the STROBE reporting checklist. Available at https://jss.amegroups.com/article/view/10.21037/jss-23-85/rc
Data Sharing Statement: Available at https://jss.amegroups.com/article/view/10.21037/jss-23-85/dss
Peer Review File: Available at https://jss.amegroups.com/article/view/10.21037/jss-23-85/prf
Conflicts of Interest: All authors have completed the ICMJE uniform disclosure form (available at https://jss.amegroups.com/article/view/10.21037/jss-23-85/coif). G.M.M. has disclosures of Globus Medical (consultancy), Device Technologies (travel), LifeHealthcare (consultancy, travel), National Surgical (travel), and SeaSpine (travel). The other authors have no conflicts of interest to declare.
Ethical Statement: The authors are accountable for all aspects of the work in ensuring that questions related to the accuracy or integrity of any part of the work are appropriately investigated and resolved. Institutional approval was granted by Epworth HealthCare (No. EH2020-515). This study was conducted in accordance with the Declaration of Helsinki (as revised in 2013) and The National Statement on Ethical Conduct in Human Research (2023). This was a review of cases collected under a standard privacy disclosure to patients that their information will be used for ongoing evaluation of outcomes and their identity will be protected in any publication arising from this. Verbal informed consent was obtained at last follow-up.
Open Access Statement: This is an Open Access article distributed in accordance with the Creative Commons Attribution-NonCommercial-NoDerivs 4.0 International License (CC BY-NC-ND 4.0), which permits the non-commercial replication and distribution of the article with the strict proviso that no changes or edits are made and the original work is properly cited (including links to both the formal publication through the relevant DOI and the license). See: https://creativecommons.org/licenses/by-nc-nd/4.0/.
References
- Wu A, March L, Zheng X, et al. Global low back pain prevalence and years lived with disability from 1990 to 2017: estimates from the Global Burden of Disease Study 2017. Ann Transl Med 2020;8:299. [Crossref] [PubMed]
- Diebo BG, Shah NV, Boachie-Adjei O, et al. Adult spinal deformity. Lancet 2019;394:160-72. [Crossref] [PubMed]
- Prost S, Pesenti S, Farah K, et al. Adult Spinal Deformities: Can Patient-Specific Rods Change the Preoperative Planning into Clinical Reality? Feasibility Study and Preliminary Results about 77 Cases. Adv Orthop 2020;2020:6120580. [Crossref] [PubMed]
- Adogwa O, Caruso JP, Eldridge CM, et al. Decisional Regret Among Older Adults Undergoing Corrective Surgery for Adult Spinal Deformity: A Single Institutional Study. Spine (Phila Pa 1976) 2022;47:E337-46. [Crossref] [PubMed]
- Patel AV, White CA, Schwartz JT, et al. Emerging Technologies in the Treatment of Adult Spinal Deformity. Neurospine 2021;18:417-27. [Crossref] [PubMed]
- Thayaparan GK, Owbridge MG, Thompson RG, et al. Designing patient-specific solutions using biomodelling and 3D-printing for revision lumbar spine surgery. Eur Spine J 2019;28:18-24. [Crossref] [PubMed]
- Wallace N, Schaffer NE, Aleem IS, et al. 3D-printed Patient-specific Spine Implants: A Systematic Review. Clin Spine Surg 2020;33:400-7. [Crossref] [PubMed]
- Barton C, Noshchenko A, Patel V, et al. Early Experience and Initial Outcomes With Patient-Specific Spine Rods for Adult Spinal Deformity. Orthopedics 2016;39:79-86. [Crossref] [PubMed]
- Kleck CJ, Calabrese D, Reeves BJ, et al. Long-Term Treatment Effect and Predictability of Spinopelvic Alignment After Surgical Correction of Adult Spine Deformity With Patient-Specific Spine Rods. Spine (Phila Pa 1976) 2020;45:E387-96. [Crossref] [PubMed]
- Prost S, Farah K, Pesenti S, et al. "Patient-specific" rods in the management of adult spinal deformity. One-year radiographic results of a prospective study about 86 patients. Neurochirurgie 2020;66:162-7. [Crossref] [PubMed]
- Sadrameli SS, Boghani Z, Steele Iii WJ, et al. Utility of Patient-Specific Rod Instrumentation in Deformity Correction: Single Institution Experience. Spine Surg Relat Res 2020;4:256-60. [Crossref] [PubMed]
- Solla F, Barrey CY, Burger E, et al. Patient-specific Rods for Surgical Correction of Sagittal Imbalance in Adults: Technical Aspects and Preliminary Results. Clin Spine Surg 2019;32:80-6. [Crossref] [PubMed]
- Schwab F, Ungar B, Blondel B, et al. Scoliosis Research Society-Schwab adult spinal deformity classification: a validation study. Spine (Phila Pa 1976) 2012;37:1077-82. [Crossref] [PubMed]
- Kim HJ, Yang JH, Chang DG, et al. Adult Spinal Deformity: Current Concepts and Decision-Making Strategies for Management. Asian Spine J 2020;14:886-97. [Crossref] [PubMed]
- Zanirato A, Damilano M, Formica M, et al. Complications in adult spine deformity surgery: a systematic review of the recent literature with reporting of aggregated incidences. Eur Spine J 2018;27:2272-84. [Crossref] [PubMed]
- Doodkorte RJP, Vercoulen TFG, Roth AK, et al. Instrumentation techniques to prevent proximal junctional kyphosis and proximal junctional failure in adult spinal deformity correction-a systematic review of biomechanical studies. Spine J 2021;21:842-54. [Crossref] [PubMed]
- McDonnell JM, Ahern DP, Wagner SC, et al. A Systematic Review of Risk Factors Associated With Distal Junctional Failure in Adult Spinal Deformity Surgery. Clin Spine Surg 2021;34:347-54. [Crossref] [PubMed]
- Mendoza-Lattes S. Proximal Junctional Kyphosis and Proximal Junctional Failure Following Spinal Deformity Surgery. Tech Orthop 2021;36:1.
- Vercoulen TFG, Doodkorte RJP, Roth A, et al. Instrumentation Techniques to Prevent Proximal Junctional Kyphosis and Proximal Junctional Failure in Adult Spinal Deformity Correction: A Systematic Review of Clinical Studies. Global Spine J 2022;12:1282-96. [Crossref] [PubMed]
- Watanabe K, Yagi M, Fujita N, et al. Proximal Junctional Kyphosis and Proximal Junctional Failure in the Treatment for Adult Spinal Deformity: Definitions and Epidemiology. Tech Orthop 2020;36:2-6.
- Ou-Yang D, Burger EL, Kleck CJ. Pre-Operative Planning in Complex Deformities and Use of Patient-Specific UNiD(™) Instrumentation. Global Spine J 2022;12:40S-4S. [Crossref] [PubMed]
- Twisk JWR. Applied mixed model analysis: a practical guide. Cambridge: Cambridge University Press; 2019.
- Lafage R, Beyer G, Schwab F, et al. Risk Factor Analysis for Proximal Junctional Kyphosis After Adult Spinal Deformity Surgery: A New Simple Scoring System to Identify High-Risk Patients. Global Spine J 2020;10:863-70. [Crossref] [PubMed]
- Sardi JP, Ames CP, Coffey S, et al. Accuracy of Rod Contouring to Desired Angles With and Without a Template: Implications for Achieving Desired Spinal Alignment and Outcomes. Global Spine J 2023;13:425-31. [Crossref] [PubMed]
- Terran J, Schwab F, Shaffrey CI, et al. The SRS-Schwab adult spinal deformity classification: assessment and clinical correlations based on a prospective operative and nonoperative cohort. Neurosurgery 2013;73:559-68. [Crossref] [PubMed]
- Lafage R, Schwab F, Challier V, et al. Defining Spino-Pelvic Alignment Thresholds: Should Operative Goals in Adult Spinal Deformity Surgery Account for Age? Spine (Phila Pa 1976) 2016;41:62-8. [Crossref] [PubMed]
- Lafage R, Schwab F, Glassman S, et al. Age-Adjusted Alignment Goals Have the Potential to Reduce PJK. Spine (Phila Pa 1976) 2017;42:1275-82. [Crossref] [PubMed]
- Scheer JK, Lafage R, Schwab FJ, et al. Under Correction of Sagittal Deformities Based on Age-adjusted Alignment Thresholds Leads to Worse Health-related Quality of Life Whereas Over Correction Provides No Additional Benefit. Spine (Phila Pa 1976) 2018;43:388-93. [Crossref] [PubMed]
- Eastlack RK, Soroceanu A, Mundis GM Jr, et al. Use of ALIF at the lumbosacral junction results in less lumbopelvic fixation failure than TLIF or no interbody fusion following correction of adult spinal deformity. Spine J 2020;20:S1. [Crossref] [PubMed]
- Park SJ, Park JS, Lee CS, et al. Metal failure and nonunion at L5-S1 after long instrumented fusion distal to pelvis for adult spinal deformity: Anterior versus transforaminal interbody fusion. J Orthop Surg (Hong Kong) 2021;29:23094990211054223. [Crossref] [PubMed]
- Charles YP, Ntilikina Y. Scoliosis surgery in adulthood: what challenges for what outcome? Ann Transl Med 2020;8:34. [Crossref] [PubMed]
- Roberts SB, Tsirikos AI. Assessing outcomes of surgical treatment in adult spinal deformity: a critical approach. Orthop Trauma 2021;35:369-75.
- Strom RG, Bae J, Mizutani J, et al. Lateral interbody fusion combined with open posterior surgery for adult spinal deformity. J Neurosurg Spine 2016;25:697-705. [Crossref] [PubMed]
- Theologis AA, Mundis GM Jr, Nguyen S, et al. Utility of multilevel lateral interbody fusion of the thoracolumbar coronal curve apex in adult deformity surgery in combination with open posterior instrumentation and L5-S1 interbody fusion: a case-matched evaluation of 32 patients. J Neurosurg Spine 2017;26:208-19. [Crossref] [PubMed]
- Passias PG, Krol O, Passfall L, et al. Three-Column Osteotomy in Adult Spinal Deformity: An Analysis of Temporal Trends in Usage and Outcomes. J Bone Joint Surg Am 2022;104:1895-904. [Crossref] [PubMed]
- Yamato Y, Hasegawa T, Yoshida G, et al. Planned two-stage surgery using lateral lumbar interbody fusion and posterior corrective fusion: a retrospective study of perioperative complications. Eur Spine J 2021;30:2368-76. [Crossref] [PubMed]
- Haddad S, Vila-Casademunt A, Yilgor Ç, et al. Combined anterior-posterior versus all-posterior approaches for adult spinal deformity correction: a matched control study. Eur Spine J 2022;31:1754-64. [Crossref] [PubMed]
- Serhan H, Mhatre D, Newton P, et al. Would CoCr rods provide better correctional forces than stainless steel or titanium for rigid scoliosis curves? J Spinal Disord Tech 2013;26:E70-4. [Crossref] [PubMed]
- Shega FD, Zhang H, Manini DR, et al. Comparison of Effectiveness between Cobalt Chromium Rods versus Titanium Rods for Treatment of Patients with Spinal Deformity: A Systematic Review and Meta-Analysis. Adv Orthop 2020;2020:8475910. [Crossref] [PubMed]
- Bowden D, Michielli A, Merrill M, et al. Systematic review and meta-analysis for the impact of rod materials and sizes in the surgical treatment of adult spine deformity. Spine Deform 2022;10:1265-78. [Crossref] [PubMed]
- Glassman SD, Bridwell K, Dimar JR, et al. The impact of positive sagittal balance in adult spinal deformity. Spine (Phila Pa 1976) 2005;30:2024-9. [Crossref] [PubMed]
- Kim HJ, Yang JH, Chang DG, et al. Proximal Junctional Kyphosis in Adult Spinal Deformity: Definition, Classification, Risk Factors, and Prevention Strategies. Asian Spine J 2022;16:440-50. [Crossref] [PubMed]
- Kim JS, Phan K, Cheung ZB, et al. Surgical, Radiographic, and Patient-Related Risk Factors for Proximal Junctional Kyphosis: A Meta-Analysis. Global Spine J 2019;9:32-40. [Crossref] [PubMed]
- Lee J, Park YS. Proximal Junctional Kyphosis: Diagnosis, Pathogenesis, and Treatment. Asian Spine J 2016;10:593-600. [Crossref] [PubMed]
- Gum JL, Carreon LY, Glassman SD. State-of-the-art: outcome assessment in adult spinal deformity. Spine Deform 2021;9:1-11. [Crossref] [PubMed]
- Laverdière C, Georgiopoulos M, Ames CP, et al. Adult Spinal Deformity Surgery and Frailty: A Systematic Review. Global Spine J 2022;12:689-99. [Crossref] [PubMed]
- Archer JE, Baird C, Gardner A, et al. Evaluating measures of quality of life in adult scoliosis: a systematic review and narrative synthesis. Spine Deform 2022;10:991-1002. [Crossref] [PubMed]
- Kim HJ, Yang JH, Chang DG, et al. Adult Spinal Deformity: A Comprehensive Review of Current Advances and Future Directions. Asian Spine J 2022;16:776-88. [Crossref] [PubMed]