The use of carbon fiber/polyetheretherketone (CF/PEEK) in pedicle screw fixation for spinal neoplasms—potential advantages in postoperative imaging and radiotherapy planning
Highlight box
Key findings
• Carbon fiber/polyetheretherketone (CF/PEEK) screws have non-inferior dosimetric prediction accuracy and minimal attenuation on collapsed-cone algorithm planning systems compared to titanium screws. Computed tomography (CT) imaging quality with CF/PEEK fixation is improved with less artefact compared to titanium.
What is known and what is new?
• Previous studies have demonstrated improvements in dose agreement and reductions in dose perturbation by using CF/PEEK instead of titanium pedicle screws in theoretical Monte Carlo simulations and proton radiotherapy. This does not reflect the clinical context of using commercial planning systems that use approximation algorithms to reduce computation time.
• This study shows that CF/PEEK retains non-inferior dosimetric prediction accuracy compared to titanium with the collapsed cone algorithm. Artefact on postoperative CT imaging is quantitatively reduced in extent with CF/PEEK.
What is the implication, and what should change now?
• We advocate increased use of CF/PEEK pedicle fixation over titanium pedicle fixation in patients with spinal neoplasms who require postoperative radiotherapy.
Introduction
Background
Spinal neoplasms can cause pain, pathological fractures, spinal instability and cord compression, all of which cause significant disability if not managed (1-4). A common surgical approach is posterior decompression, tumor debulking and pedicle fixation to stabilize the spine. Stabilization is required when a patient develops spinal instability—“a syndrome of movement-related pain, symptomatic or progressive vertebral deformity and neurological compromise under physiological load” (1)—or when extensive bony and soft tissue resection is necessary for tumour excision (5).
Most pedicle fixation systems are made of titanium. However, titanium generates significant metal-related imaging artefacts—bright streaking and dark bands—on computed tomography (CT) that mask normal tissue (6) and complicate postoperative visualization of the spine. Furthermore, high-density metal implants create errors in estimating dose drop-off with dose calculation algorithms based on converting CT-Hounsfield units (CT-HU) to tissue density, distorting radiation dose and compromising tumor control (7,8).
Radiolucent alternatives to titanium would allow more accurate radiotherapy planning and delivery, and postoperative monitoring for local recurrence. Carbon fiber-polyetheretherketone (CF/PEEK) is a novel material that has suitable biomechanical, biocompatible and osseointegrative properties for pedicle fixation (9,10). Preliminary single-arm studies have demonstrated safe usage of CF/PEEK pedicle screws and other instrumentation for primary and metastatic spinal neoplasms, with improved postoperative imaging quality allowing early detection of recurrence where long-term follow-up is anticipated (11-13). However, a systematic review by Khan et al. (14) concluded that there was insufficient evidence that postoperative imaging quality translated to benefits in oncological outcomes such as local control and survival, making it difficult to justify its usage given its high cost and underdeveloped instrumentation relative to titanium. In Australia, there is no cost difference between CF/PEEK and titanium as materials for pedicle fixation systems.
Rationale and knowledge gap
Early studies have demonstrated improvements in dose agreement and reductions in dose perturbation by using CF/PEEK instead of titanium pedicle screws. With photon beam radiotherapy, Nevelsky et al. (15) observed dose differences between measured doses and Monte Carlo simulated doses were less than 6% for CF/PEEK versus 31% for titanium plates. Improvements in CT and magnetic resonance imaging (MRI) visualization have also been demonstrated in water phantom models (16).
While Monte Carlo simulations are considered gold standard for modelling photon transport, their long computation times limit their clinical utility. There is a lack of data evaluating the performance of CF/PEEK in a more practical setting. In clinical practice, patients undergo a planning CT, where metal artefact reducing protocols are often applied as standard. A commercial treatment planning system (TPS) is then used to generate a plan for irradiation. Three-dimensional (3D) conventional external beam radiotherapy is still commonly used in the treatment of bony metastases (2,12). Additionally, artefact reduction has not yet been evaluated in CF/PEEK-exclusive fixation systems, which may demonstrate further benefit in qualitative and quantitative interpretations of postoperative CT imaging.
Objective
This study aims to investigate and quantify the benefit of using CF/PEEK pedicle fixation compared to titanium fixation in radiotherapy planning and delivery for spinal neoplasms in the context of external photon beam radiotherapy with a commercial TPS. Radiotherapy planning was investigated by quantifying the extent of imaging artefact on plannings CTs in both lumbar spine models and patient scans at various anatomical landmarks. Radiation delivery was assessed by comparing the accuracy of TPS predicted dose to measured radiation doses for both materials. We present this article in accordance with the SQUIRE reporting checklist (available at https://jss.amegroups.com/article/view/10.21037/jss-23-93/rc).
Methods
This project was undertaken after ethical approval was granted by the South East Sydney Human Research Ethics Committee (reference number 2019/ETH12613), on 4th May 2020. This project was conducted in accordance with the Declaration of Helsinki (as revised in 2013). Informed consent was obtained from the patients involved in the retrospective case series part of this study.
Dosimetric analysis
To measure the effect of the different materials on photon beam dose delivered by a megavolt linear accelerator (Elekta, Stockholm, Sweden), each pedicle screw was cast in a 10 cm × 10 cm × 2 cm volume of silicone tissue-equivalent material (Eurosil-4 pink, SynTec, Schouten Group, the Netherlands). The casts containing the CF/PEEK (CarboClearTM, CarboFix Orthopedics, Herziliya, IL, USA) and titanium screws (SmartLoc, A-Spine, New Taipei City) were centered on the beam’s central axis, between 50 mm of Solid Water® (RMI457, Gammex) above and 100 mm of Solid Water® below (Figure 1). These screws were chosen as the tulips have the same composition as the screws. A radiotherapy plan was generated—a 6 megavolt (MV), 10 cm × 10 cm single field fixed photon beam with a source-to-phantom entrance distance of 100 cm—using the collapsed cone algorithm on the TPS (RayStation® v9B, RaySearch Laboratories, Stockholm, Sweden). Material override was performed for the titanium screws, because the RayStation CT-HU-to-mass density curve calibration does not extend to titanium’s CT-HU value of 6,000 CT-HU. This involved manually contouring the titanium screws and reassigning them to its density of 4.540 g/cm3. Visible artefact volume was also contoured and reassigned to the density of water (1 g/cm3). The CF/PEEK screws did not require material override.
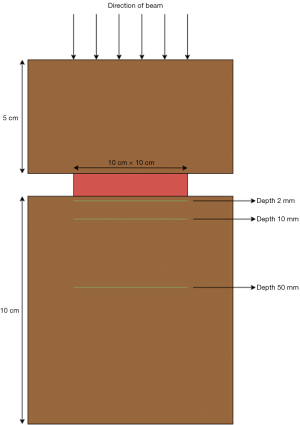
A standard, homogeneous dose of 200 monitor units (MUs) was delivered to the model. 10 cm × 10 cm External Beam Therapy 3 (EBT3) GafChromicTM films (Ashland, Advanced Materials, Bridgewater, NJ, USA) were placed at depths of 2, 10, and 50 mm relative to the screw, sandwiched between Solid Water® slabs, orthogonal to the beam direction. These distances were chosen so that the GafChromicTM films could be placed between Solid Water® blocks, which are manufactured with depths of 2, 10 and 50 mm.
A dose calibration curve of optical density change in the radiochromic film to received dose was created for the 6 MV beam by delivering known doses up to 200 centigray (cGy) to the isocenters of separate EBT3 GafChromicTM films at a fixed depth of 1.5 cm (Figure 2). EBT3 films were scanned using an Expression 10000XL-A3 flatbed scanner (Epson, Nagano, Japan). Red-green-blue (RGB) film images were collected at 48 bits at a spatial resolution of 75 dpi (0.34 mm/pixel). The average optical density change was taken from three scans of each film. Red channel data from the mean RGB image was correlated to the prescribed dose to generate a calibration curve.
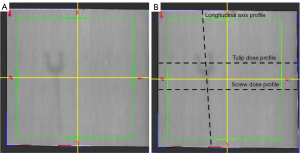
The same method was then used with the 10 cm × 10 cm EBT3 films to generate two-dimensional absolute dose maps using the calibration curves, measured at 1 mm × 1 mm intervals.
The dose maps from RayStation and the EBT3 films at 2, 10 and 50 mm relative to the screw and silicone cast were exported to Excel to evaluate dose agreement. The transverse (tulip and screw) and longitudinal axes of the screws were identified, and the predicted doses (with and without material override for the titanium screws) (RayStation) were subtracted from the absolute measured (EBT3) doses. The absolute values of these differences were calculated to produce an absolute dose difference profile. A smaller absolute dose difference would indicate better agreement between predicted dose and measured dose.
Artefact image quality
Pedicle fixation construct water phantom models
Two lumbar spine models (Sawbones®) were instrumented with either CF/PEEK or titanium screws and used to investigate the influence of implant material on the image quality of planning CT scans. Both models had 6 screws inserted—four screws in the left pedicles and two screws in the right pedicles—connected by two longitudinal rods made of the same material as the pedicle screws (Figures 3,4).
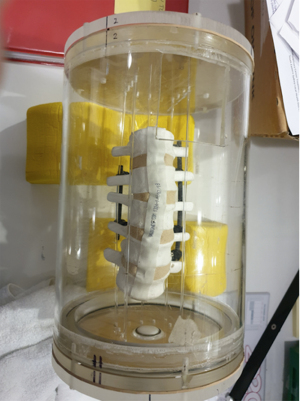
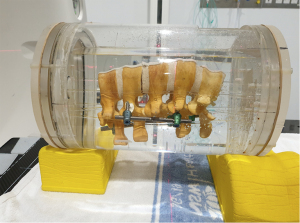
A cylindrical water phantom was created to house the spine models. Plastic rods elevated the models above the base of the tank to simulate physiological distance of the spine above the CT bed. After positioning, the water phantoms were filled with water. Scans were obtained using single energy metal artefact reduction (SEMAR) protocols (Toshiba America Medical Systems, Tustin, CA, USA) at 120 kV and 3 mm slices (Aquilion ONETM, Canon Medical Systems).
Scans were transferred onto RayStation, and the presence of artefact was assessed by manually identifying four spherical regions of interest (ROI) of radius 0.5 cm at each instrumented vertebral body level—in the vertebral canal, and 0.5 cm posterior, medial and lateral to the protruding tulips of the screw, at each vertebral body level with instrumentation (Figure 5). For the vertebral canal ROI, a sphere with 0.5 cm radius that would fit in the vertebral canal was identified. For the ROIs defined relative to the tulips, the transverse and longitudinal axes through the tulip (as indicated by the dotted lines in Figure 5) were drawn, and the center chosen such that the circumference of the ROI would be 0.5 cm away from the extremity of the tulip. The standard deviations of CT-HU values in each ROI were obtained from the software and used to calculate an artefact index (AI) for each region.
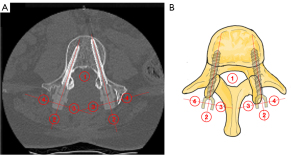
The formula for AI is given as:
SDROI is the standard deviation of the CT-HU values assigned to all of the voxels in the ROI. This is compared to the standard deviation of CT-HU in a sphere taken at another point in the water phantom where there was no discernible artefact (SDbackground). If SDROI < SDbackground, AI was recorded as 0. Smaller AI values imply less artefact present in that ROI.
Retrospective case series
A retrospective review of medical records from December 2009 to May 2020 was performed to identify a non-consecutive, unmatched case series of cancer patients who underwent CF/PEEK or titanium pedicle screw fixation.
Artefact volume was calculated using the same ROI definitions as the pedicle fixation construct models. SDbackground was defined by sampling the same regions in a control patient who had undergone surgical resection of a spinal neoplasm with no spinal implants, and had a CT scan for postoperative radiotherapy planning available.
Statistical analysis
Statistical significance was defined as P<0.05 for all statistical tests performed. Statistical tests were performed using IBM SPSS Statistics, version 26. All graphs were generated using R Studio, version 1.3.1056.
A F-test was performed to compare the variances of the dose difference profiles between the titanium and CF/PEEK screws. An unpaired t-test was then used to compare the mean absolute dose difference between the titanium (with and without material override separately) and the CF/PEEK screw. One-tailed exact significance values were calculated.
Normality assumption testing of the AIs in the water phantom models and patient plans was performed using the Shapiro-Wilk test. Due to small sample size and failure of some samples to meet normality assumptions, AIs in the titanium and CF/PEEK groups were compared using the Mann-Whitney U test (Wilcoxon 2 sample rank-sum test). One-tailed exact significance values were calculated.
Results
Dosimetric analysis
Figure 6 shows the dose profiles through the tulip, screw and longitudinal axis of the screw at 6 MV.
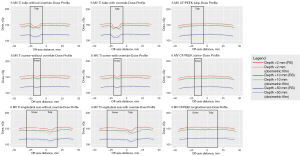
Dose attenuation by the titanium screw was observable across all three profiles, with and without manual reassignment of titanium density. There was minimal dose attenuation by the CF/PEEK screws.
CF/PEEK screws displayed non-inferior dose agreement between the measured dose profiles and the RayStation-generated dose profiles compared to titanium screws (see Tables 1-3). At a depth of 2 mm, there was a statistically significant reduction in absolute dose difference across all measured axes (tulip, screw and longitudinal axis). At greater depths, there was no statistically significant reduction in absolute dose difference compared to titanium.
Table 1
Titanium material | Mean (SD) | CF mean (SD) | P |
---|---|---|---|
Tulip (absolute dose difference, cGy) | |||
Ti (without override) | 4.23 (2.46) | 3.08 (2.11) | 0.001* |
Ti (override) | 4.42 (2.26) | <0.001* | |
Screw (absolute dose difference, cGy) | |||
Ti (without override) | 2.99 (1.62) | <0.001* | |
Ti (override) | 3.21 (1.60) | <0.001* | |
Longitudinal axis (absolute dose difference, cGy) | |||
Ti (without override) | 4.80 (3.74) | 2.05 (1.34) | <0.001* |
Ti (override) | 4.19 (2.94) | <0.001* |
*, statistically significant differences (i.e., P<0.05). SD, standard deviation; CF, carbon fiber.
Table 2
Titanium material | Mean (SD) | CF mean (SD) | P |
---|---|---|---|
Tulip (absolute dose difference, cGy) | |||
Ti (without override) | 2.07 (1.97) | 2.50 (1.80) | 0.148 |
Ti (override) | 2.06 (1.94) | 0.132 | |
Screw (absolute dose difference, cGy) | |||
Ti (without override) | 1.87 (1.13) | 1.84 (1.41) | 0.910 |
Ti (override) | 2.80 (3.10) | 0.015* | |
Longitudinal axis (absolute dose difference, cGy) | |||
Ti (without override) | 3.40 (2.32) | 2.99 (1.69) | 0.194 |
Ti (override) | 3.55 (2.83) | 0.122 |
*, statistically significant differences (i.e., P<0.05). SD, standard deviation; CF, carbon fiber.
Table 3
Titanium material | Mean (SD) | CF mean (SD) | P |
---|---|---|---|
Tulip (absolute dose difference, cGy) | |||
Ti (without override) | 1.58 (1.18) | 1.64 (1.09) | 0.723 |
Ti (override) | 1.98 (1.35) | 0.091 | |
Screw (absolute dose difference, cGy) | |||
Ti (without override) | 1.43 (0.93) | 1.64 (1.05) | 0.179 |
Ti (override) | 1.48 (1.08) | 0.338 | |
Longitudinal axis (absolute dose difference, cGy) | |||
Ti (without override) | 2.61 (1.61) | 2.64 (1.87) | 0.900 |
Ti (override) | 2.51 (1.65) | 0.647 |
SD, standard deviation; CF, carbon fiber.
Percentage dose differences between measured and predicted doses (relative to the measured dose) were also non-inferior for CF/PEEK screws compared to the titanium screws (see Tables 4-6). Similarly, statistically significant reductions were observed across all measured axes at a depth of 2 mm, but not at greater depths.
Table 4
Titanium material | Mean (SD) | CF mean (SD) | P |
---|---|---|---|
Tulip (relative dose difference, %) | |||
Ti (without override) | 2.88 (1.75) | 2.07 (1.46) | <0.001* |
Ti (override) | 2.99 (1.59) | <0.001* | |
Screw (relative dose difference, %) | |||
Ti (without override) | 1.99 (1.10) | 1.33 (0.83) | <0.001* |
Ti (override) | 2.14 (1.18) | <0.001* | |
Longitudinal axis (relative dose difference, %) | |||
Ti (without override) | 3.35 (2.80) | 1.34 (0.89) | <0.001* |
Ti (override) | 2.91 (2.18) | <0.001* |
*, statistically significant differences (i.e., P<0.05). SD, standard deviation; CF, carbon fiber.
Table 5
Titanium material | Mean (SD) | CF mean (SD) | P |
---|---|---|---|
Tulip (relative dose difference, %) | |||
Ti (without override) | 1.46 (1.44) | 1.68 (1.19) | 0.145 |
Ti (override) | 1.44 (1.40) | 0.124 | |
Screw (relative dose difference, %) | |||
Ti (without override) | 1.28 (0.77) | 1.25 (0.95) | 0.424 |
Ti (override) | 1.93 (2.15) | 0.007* | |
Longitudinal axis (relative dose difference, %) | |||
Ti (without override) | 2.36 (1.62) | 2.01 (1.13) | 0.054 |
Ti (override) | 2.45 (1.92) | 0.038* |
*, statistically significant differences (i.e., P<0.05). SD, standard deviation; CF, carbon fiber.
Table 6
Titanium material | Mean (SD) | CF mean (SD) | P |
---|---|---|---|
Tulip (relative dose difference, %) | |||
Ti (without override) | 1.34 (1.01) | 1.37 (0.90) | 0.422 |
Ti (override) | 1.68 (1.18) | 0.031* | |
Screw (relative dose difference, %) | |||
Ti (without override) | 1.21 (0.79) | 1.38 (0.87) | 0.099 |
Ti (override) | 1.25 (0.93) | 0.186 | |
Longitudinal axis (relative dose difference, %) | |||
Ti (without override) | 2.26 (1.44) | 2.18 (1.54) | 0.358 |
Ti (override) | 2.17 (1.42) | 0.481 |
*, statistically significant differences (i.e., P<0.05). SD, standard deviation; CF, carbon fiber.
The difference in the predicted dose profiles with and without material reassignment was very minor.
Artefact image quality
Pedicle fixation construct water models
Results are summarized in Table 7. All data met normality assumptions using the Shapiro Wilk test at the 5% significance.
Table 7
Region | Median AI | N | Shapiro-Wilk test | Mann-Whitney U (Wilcoxon Rank-Sum test) |
||||
---|---|---|---|---|---|---|---|---|
Statistic | P | Median difference | Z | Exact one-tailed sig. (P) | ||||
Vertebral canal (Ti) | 41.44 | 4 | 0.846 | 0.213 | 32.03 | −2.309 | 0.029* | |
Vertebral canal (CF) | 9.41 | 4 | 0.932 | 0.604 | ||||
Posterior to screw (Ti) | 79.39 | 6 | 0.684 | 0.004 | 75.87 | −2.882 | 0.002* | |
Posterior to screw (CF) | 3.52 | 6 | 0.909 | 0.432 | ||||
Medial to screw (Ti) | 50.53 | 5 | 0.913 | 0.483 | 40.97 | −2.739 | 0.004* | |
Medial to screw (CF) | 9.56 | 6 | 0.859 | 0.187 | ||||
Lateral to screw (Ti) | 51.83 | 5 | 0.913 | 0.484 | 47.23 | −2.562 | 0.009* | |
Lateral to screw (CF) | 4.603 | 6 | 0.876 | 0.251 |
*, statistically significant differences (i.e., P<0.05). Ti, titanium; CF, carbon fiber; AI, artefact index; sig., significance.
The median AIs were all lower in the regions of interest around the CF/PEEK models compared to the titanium models. There was a statistically significant reduction in AI in all regions of interest.
Retrospective case series
Three patients with titanium fixation and one patient with CF/PEEK fixation had radiotherapy plans available for review on the treatment planning software. The radiotherapy plans were based on CT scans that were performed during the period of 2017–2020. There were 18 titanium screws across 11 vertebral levels for the three patients with titanium screws. There were 5 CF/PEEK screws across 3 vertebral levels for the patient with CF/PEEK fixation. In one patient with titanium fixation, two regions of interest medial to the screw were excluded due to overlap with a titanium crossbar. Results are presented in Table 8.
Table 8
Region | Median AI | N | Shapiro-Wilk test | Mann-Whitney U (Wilcoxon Rank-Sum test) |
||||
---|---|---|---|---|---|---|---|---|
Statistic | P | Median difference | Z | Exact one-tailed sig. (P) | ||||
Vertebral canal (Ti) | 66.76 | 11 | 0.920 | 0.318 | 60.21 | −2.569 | 0.005* | |
Vertebral canal (CF) | 6.55 | 3 | 0.779 | 0.066 | ||||
Posterior to screw (Ti) | 75.55 | 18 | 0.848 | 0.008 | 63.44 | −3.354 | <0.001* | |
Posterior to screw (CF) | 12.11 | 5 | 0.898 | 0.401 | ||||
Medial to screw (Ti) | 118.60 | 16 | 0.894 | 0.065 | 99.78 | −2.807 | 0.003* | |
Medial to screw (CF) | 19.82 | 5 | 0.960 | 0.810 | ||||
Lateral to screw (Ti) | 119.10 | 18 | 0.904 | 0.069 | 85.80 | −3.130 | <0.001* | |
Lateral to screw (CF) | 33.30 | 5 | 0.962 | 0.824 |
*, statistically significant differences (i.e., P<0.05). Ti, titanium; CF, carbon fiber; AI, artefact index; sig., significance.
The median AIs in the ROIs in the CF/PEEK patients were all lower than the titanium patients. There was a statistically significant reduction in AI in all regions of interest.
Discussion
Dosimetric analysis
Key findings
CF/PEEK offers modestly improved dosimetric accuracy over titanium at small depths and is non-inferior at greater depths.
Predictions of dose profiles and hardware-induced dose attenuation incorporating manual reassignment of titanium density were closer to the experimentally measured profiles, showing the importance of knowing prosthetic material composition during radiotherapy planning (17).
Dose attenuation is improved with CF/PEEK versus titanium, with minimal attenuation relative to the rest of the dose profile at depths up to 50 mm, even with the larger mass of the tulip.
Strengths and limitations
This study employed radiochromic films to measure the dose received at several depths beneath a pedicle screw embedded in a tissue-equivalent bolus. Radiochromic films have high spatial resolution and can measure radiation dose in two dimensions, allowing precise comparison with TPS-calculated dose profiles. Converting optical density change to dose using the red channel method is more sensitive and has less total noise than the multi-channel method for doses less than 400 cGy (18,19).
The observed difference between measured and predicted doses can also be explained by the presence of signal noise in the experimental measurements. Signal noise may be fixed (dust, micro-scratches, spontaneous polymerization) or random (quantum noise in the radiation field, lamp fluctuations in the flatbed scanner) (19), and the curvature of radiochromic films can introduce errors of 1–4% (20), though this was mitigated by compressing the films between the solid water blocks. Mathematical smoothing techniques, e.g., weighted three-point algorithm (8) and Fourier analysis, can reduce the impact of signal noise in future studies.
Limitations of this study include the simplistic comparison of a single screw, and signal noise in the radiochromic film measurements. However, the results of this study are consistent with other studies, demonstrating that CF/PEEK pedicle fixation is superior to titanium fixation in dose agreement and dose homogeneity, though the benefit may not be as much compared to particle beam therapy (8,21,22).
Comparison with similar research
Nevelsky et al. (15) measured point dose differences of <1% between measured doses and Monte Carlo simulated doses for CF/PEEK and titanium plates. Poel et al. (21) also demonstrated greater overdose with a titanium construct, up to 8%, compared to approximately 5% with CF/PEEK, however, this was performed in proton therapy using different algorithms.
With dose attenuation, Nevelsky et al. (15) measured dose attenuation of <0.5% at depths of 1–6 mm when a beam was passed across the CF/PEEK screw, while dose attenuation by the titanium screw ranged from 7.2–12.5% at the same depths.
To the best of the authors’ knowledge, the literature on the dosimetric properties of CF/PEEK have used Monte Carlo simulations to calculate dose distribution. While Monte Carlo simulations are the current gold-standard for calculating dose distribution, they require significant computational time, making them unsuitable in a practical setting. The collapsed cone algorithm exponentially reduces computational time by reducing the number of scatter directions that photons are simulated. When used to calculate a radiation plan on the same model, Pereira (23) found that a Monte Carlo plan could take more than 2 hours to compute, depending on the set level of statistic uncertainty, compared to less than 4 minutes with a collapsed cone algorithm. Literature investigating the collapsed cone algorithm show that dose agreement with Monte Carlo simulations is generally excellent in water and heterogeneous media (24). However, in the presence of high atomic number interfaces such as titanium prostheses, the collapsed cone algorithm underestimates the dose by 5–22% received at the prosthetic interface (25). This dosimetric error is rectified with CF/PEEK screws, which demonstrate a statistically significant reduction in absolute dose difference 2 mm from the screw interface.
Implications and actions needed
A plan may no longer fall within safety margins of 95–107% of the prescribed dose if titanium pedicle fixation induces dose attenuation greater than 5% (26). Minimizing dose attenuation reduces the risk of radiation underdose distal to the pedicle fixation construct, giving radiation oncologists greater confidence that the target volume receives the intended tumor control, and the adjacent normal tissue doses are kept within tolerance doses.
Further studies with more complex pedicle fixation modelling should investigate additional modalities such as stereotactic and modulated radiotherapy, which will further quantify the benefit of CF/PEEK fixation in a range of common clinical situations.
Artefact image quality
Key findings
The results of the models and patient plans indicate CF/PEEK achieves a statistically significant reduction in AI over titanium, in important areas such as the vertebral canal and the tissue around the protruding tulips (see Figure 7).
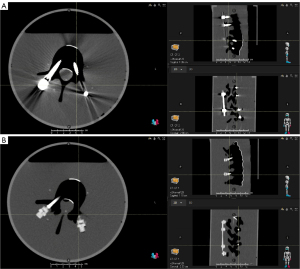
Strengths and limitations
There are limitations with the AI metric. Measurement of artefact volume and spatial distribution, which is not reflected in AI, remains pertinent for a planner who has to manually contour artefact and override the tissue density to the appropriate preset.
Comparison with similar research
Other studies such as Ringel et al. (27) and Poel et al. (21) reported significant reductions of 50–90% in artefact volume, defining artefact as voxels where CT-HU deviation from normal tissue exceeded 100 CT-HU. While artefact volume reduction is certainly an important consideration in image quality, especially for postoperative monitoring (28,29), the severity of artefact within the target volume can magnify CT-HU to tissue density conversion errors and thus dosage calculation error. A study by Thomas (30) noted that errors of more than 8% in CT-HU values would correspond to 1% error in dosimetry, compounding error to dose agreement and calculation.
Explanations of findings
In this study, the AI was chosen as a quantitative metric evaluating the reduction in artefact offered by CF/PEEK fixation. No other studies, to the best of the authors’ knowledge, quantify CF/PEEK’s artefact reduction effect using AI. AI was used by Dong et al. (31) to compare different kilovoltage protocols in monochromatic CT in removing metal artefact generated by titanium pedicle screws. In this study, the formula used to calculate AI was modified to use the absolute value of the differences of squares, allowing inclusion of results where the standard deviation of CT-HU in the region of interest was higher than the standard deviation of CT-HU in the control.
Both artefact reduction protocols and CF/PEEK implants have a global artefact removal effect, justifying the validity of AI.
Implications and actions needed
Radiolucent implants reduce artefact volume and intensity, improving subjective CT image quality while bypassing the need for material reassignment, which is laborious, susceptible to error and inter-planner variability (7). Additionally, CF/PEEK removes the need for metal artefact reduction algorithms (e.g., sinogram completion and model-based iterative approaches), which can degrade spatial resolution and introduce their own artefact (7,32) while increasing computational time of generating a planning CT.
Further studies should also image in other planes where other anatomical landmarks are more visible, e.g., the neural foramina in sagittal view. The neural foramen is a site that the surgeon often inspects to assess for postoperative recurrence, and is susceptible to artefact.
Conclusions
CF/PEEK pedicle fixation is a new system which has potential benefits in multiple aspects of management for patients who require surgery and postoperative photon beam radiotherapy for a spinal neoplasm. These main benefits are due to the radiolucent nature of CF/PEEK, which addresses the key disadvantage of imaging artefacts and dose perturbation caused by titanium constructs. This preliminary study demonstrates that CF/PEEK pedicle screws have non-inferior dose prediction accuracy in TPSs using the collapsed-cone algorithm, and reduces dose attenuation, compared to titanium screws. Additionally, CF/PEEK constructs result in significantly improved imaging quality of planning CTs due to reduction in artefact index. These dosimetric and imaging benefits can save substantial time by eliminating the need to manually contour implants and artefacts.
We recommend the use of CF/PEEK pedicle fixation over titanium pedicle fixation in patients with spinal neoplasms who require postoperative radiotherapy. Further studies are needed to evaluate the potential benefit of CF/PEEK fixation in more complex radiotherapy situations.
Acknowledgments
The authors would like to acknowledge the departments of neurosurgery (Professor Rajesh Reddy, Dr. Peter Wilson) and radiation oncology (Dr. Wenchang Wong, Dr. Stephen Thompson, Professor Michael Jackson) at Prince of Wales Hospital for supporting this project and providing a list of patients whose data could be included for this study. We are grateful to Aidan McEvoy for providing the lumbar spine models and pedicle screws used in this study. We thank David Stewart who developed a script to obtain CT-HU standard deviations from planning CTs. We also thank Associate Professor Boaz Shulruf for providing statistical support and Wen Jie Choy for reviewing the final manuscript.
Funding: None.
Footnote
Reporting Checklist: The authors have completed the SQUIRE reporting checklist. Available at https://jss.amegroups.com/article/view/10.21037/jss-23-93/rc
Data Sharing Statement: Available at https://jss.amegroups.com/article/view/10.21037/jss-23-93/dss
Peer Review File: Available at https://jss.amegroups.com/article/view/10.21037/jss-23-93/prf
Conflicts of Interest: All authors have completed the ICMJE uniform disclosure form (available at https://jss.amegroups.com/article/view/10.21037/jss-23-93/coif). R.M. serves as the Editor-in-Chief of Journal of Spine Surgery. The other authors have no conflicts of interest to declare.
Ethical Statement: The authors are accountable for all aspects of the work in ensuring that questions related to the accuracy and integrity of any part of the work are appropriately investigated and resolved. This project was undertaken after ethical approval was granted by the South East Sydney Human Research Ethics Committee (reference number 2019/ETH12613), on 4th May 2020. This project was conducted in accordance with the Declaration of Helsinki (as revised in 2013). Informed consent was obtained from the patients involved in the retrospective case series part of this study.
Open Access Statement: This is an Open Access article distributed in accordance with the Creative Commons Attribution-NonCommercial-NoDerivs 4.0 International License (CC BY-NC-ND 4.0), which permits the non-commercial replication and distribution of the article with the strict proviso that no changes or edits are made and the original work is properly cited (including links to both the formal publication through the relevant DOI and the license). See: https://creativecommons.org/licenses/by-nc-nd/4.0/.
References
- Laufer I, Rubin DG, Lis E, et al. The NOMS framework: approach to the treatment of spinal metastatic tumors. Oncologist 2013;18:744-51. [Crossref] [PubMed]
- Spratt DE, Beeler WH, de Moraes FY, et al. An integrated multidisciplinary algorithm for the management of spinal metastases: an International Spine Oncology Consortium report. Lancet Oncol 2017;18:e720-30. [Crossref] [PubMed]
- Graber JJ, Nolan CP. Myelopathies in patients with cancer. Arch Neurol 2010;67:298-304. [Crossref] [PubMed]
- Abrahm JL. Assessment and treatment of patients with malignant spinal cord compression. J Support Oncol 2004;2:377-88, 391; discussion 391-3, 398, 401.
- Kurd MF, Vaccaro AR. Spinal instability: Trauma and tumor in the thoracolumbar spine. Seminars in Spine Surgery. 2013;25:110-8.
- Boas FE, Fleischmann D. CT artifacts: causes and reduction techniques. Imaging in Medicine. 2012;4:229-40.
- Giantsoudi D, De Man B, Verburg J, et al. Metal artifacts in computed tomography for radiation therapy planning: dosimetric effects and impact of metal artifact reduction. Phys Med Biol 2017;62:R49-80. [Crossref] [PubMed]
- Mastella E, Molinelli S, Magro G, et al. Dosimetric characterization of carbon fiber stabilization devices for post-operative particle therapy. Phys Med 2017;44:18-25. [Crossref] [PubMed]
- Devine DM, Hahn J, Richards RG, et al. Coating of carbon fiber-reinforced polyetheretherketone implants with titanium to improve bone apposition. J Biomed Mater Res B Appl Biomater 2013;101:591-8. [Crossref] [PubMed]
- Kang KT, Koh YG, Son J, et al. Biomechanical evaluation of pedicle screw fixation system in spinal adjacent levels using polyetheretherketone, carbon-fiber-reinforced polyetheretherketone, and traditional titanium as rod materials. Composites Part B: Engineering. 2017;130:248-56.
- Alvarez-Breckenridge C, de Almeida R, Haider A, et al. Carbon Fiber-Reinforced Polyetheretherketone Spinal Implants for Treatment of Spinal Tumors: Perceived Advantages and Limitations. Neurospine 2023;20:317-26. [Crossref] [PubMed]
- Trungu S, Ricciardi L, Forcato S, et al. Percutaneous carbon-PEEK instrumentation for spine tumors: a prospective observational study. J Neurosurg Sci 2023;67:303-10. [Crossref] [PubMed]
- Trungu S, Ricciardi L, Forcato S, et al. Anterior Corpectomy and Plating with Carbon-PEEK Instrumentation for Cervical Spinal Metastases: Clinical and Radiological Outcomes. J Clin Med 2021;10:5910. [Crossref] [PubMed]
- Khan HA, Ber R, Neifert SN, et al. Carbon fiber-reinforced PEEK spinal implants for primary and metastatic spine tumors: a systematic review on implant complications and radiotherapy benefits. J Neurosurg Spine 2023;39:534-47. [Crossref] [PubMed]
- Nevelsky A, Borzov E, Daniel S, et al. Perturbation effects of the carbon fiber-PEEK screws on radiotherapy dose distribution. J Appl Clin Med Phys 2017;18:62-8. [Crossref] [PubMed]
- Krätzig T, Mende KC, Mohme M, et al. Carbon fiber-reinforced PEEK versus titanium implants: an in vitro comparison of susceptibility artifacts in CT and MR imaging. Neurosurg Rev 2021;44:2163-70.
- Rijken JD, Colyer CJ. Dose uncertainties associated with a set density override of unknown hip prosthetic composition. J Appl Clin Med Phys 2017;18:301-6. [Crossref] [PubMed]
- Micke A, Lewis DF, Yu X. Multichannel film dosimetry with nonuniformity correction. Med Phys 2011;38:2523-34. [Crossref] [PubMed]
- González-López A, Vera-Sánchez JA, Ruiz-Morales C. The incidence of the different sources of noise on the uncertainty in radiochromic film dosimetry using single channel and multichannel methods. Phys Med Biol 2017;62:N525-36.
- Palmer AL, Bradley DA, Nisbet A. Evaluation and mitigation of potential errors in radiochromic film dosimetry due to film curvature at scanning. J Appl Clin Med Phys 2015;16:5141. [Crossref] [PubMed]
- Poel R, Belosi F, Albertini F, et al. Assessing the advantages of CFR-PEEK over titanium spinal stabilization implants in proton therapy-a phantom study. Phys Med Biol 2020;65:245031. [Crossref] [PubMed]
- Müller BS, Ryang YM, Oechsner M, et al. The dosimetric impact of stabilizing spinal implants in radiotherapy treatment planning with protons and photons: standard titanium alloy vs. radiolucent carbon-fiber-reinforced PEEK systems. J Appl Clin Med Phys 2020;21:6-14. [Crossref] [PubMed]
- Pereira S. A comparative analysis of Monte Carlo and collapsed cone dose calculation algorithm for Monaco 3D treatment plans. Florida Atlantic University; 2022.
- Ahnesjö A. Collapsed cone convolution of radiant energy for photon dose calculation in heterogeneous media. Med Phys 1989;16:577-92. [Crossref] [PubMed]
- Paulu D, Alaei P. Evaluation of dose calculation accuracy of treatment planning systems at hip prosthesis interfaces. J Appl Clin Med Phys 2017;18:9-15. [Crossref] [PubMed]
- 7. Prescribing, Recording, and Reporting. Journal of the ICRU 2017;14:101-9.
- Ringel F, Ryang YM, Kirschke JS, et al. Radiolucent Carbon Fiber-Reinforced Pedicle Screws for Treatment of Spinal Tumors: Advantages for Radiation Planning and Follow-Up Imaging. World Neurosurg 2017;105:294-301. [Crossref] [PubMed]
- Boriani S, Tedesco G, Ming L, et al. Carbon-fiber-reinforced PEEK fixation system in the treatment of spine tumors: a preliminary report. Eur Spine J 2018;27:874-81. [Crossref] [PubMed]
- Cofano F, Di Perna G, Monticelli M, et al. Carbon fiber reinforced vs titanium implants for fixation in spinal metastases: A comparative clinical study about safety and effectiveness of the new "carbon-strategy". J Clin Neurosci 2020;75:106-11. [Crossref] [PubMed]
- Thomas SJ. Relative electron density calibration of CT scanners for radiotherapy treatment planning. Br J Radiol 1999;72:781-6. [Crossref] [PubMed]
- Dong Y, Shi AJ, Wu JL, et al. Metal artifact reduction using virtual monochromatic images for patients with pedicle screws implants on CT. Eur Spine J 2016;25:1754-63. [Crossref] [PubMed]
- Gjesteby L, De Man B, Jin Y, et al. Metal Artifact Reduction in CT: Where Are We After Four Decades? IEEE Access 2016;4:5826-49.