Expandable cages that expand both height and lordosis provide improved immediate effect on sagittal alignment and short-term clinical outcomes following minimally invasive transforaminal lumbar interbody fusion (MIS TLIF)
Highlight box
Key findings
• This study presents the utilization of an expandable cage designed to expand both disc height and lordosis, demonstrating significantly improved segmental lordosis and disc angle compared to conventional expandable cages that only increase height.
What is known and what is new?
• Minimally invasive transforaminal lumbar interbody fusion has been referred to as a “kyphosing operation” due to challenges in achieving lordotic restoration or addressing sagittal alignment issues, even when using expandable cages that increase disc height.
• This study is the first to report the advantages of that expandable cage explicitly designed not only for height augmentation but also for restoration of segmental lordosis when compared to conventional expandable cages.
What is the implication, and what should change now?
• In cases where surgeons aim to restore segmental lordosis, considering an expandable cage that addresses both height and lordosis expansion is a viable option. However, to prevent early subsidence, it is imperative to avoid applying excessive force to achieve greater disc height or lordosis.
Introduction
“Kyphosing procedure” is a term often used to highlight the potential drawback of minimally invasive transforaminal lumbar interbody fusion (MIS TLIF) in terms of its negative impact on postoperative spinal alignment (1-3). Although MIS TLIF offers several benefits such as reduced collateral injuries to back muscles, less hospitalization time, decreased postoperative pain, minimized blood loss during surgery, and effectiveness comparable to open fusion surgery, several studies have shown that some patients failed to achieve substantial improvements in segmental lordosis after the surgery (4-9). To address this issue, the introduction of the expandable cage aims to obtain improvement in the disc height, facilitate cage insertion without damaging the vertebral endplate, and potentially achieve more effective increases in lordosis compared to the conventional static cage (10). Despite the use of expandable cages in MIS TLIF, studies have shown that while disc height can be effectively increased, there is still limited improvement observed in postoperative sagittal alignment including segmental and lumbar lordosis. Moreover, it has been observed that patients who had preoperative lordotic segment often experience a kyphotic change after undergoing MIS TLIF (11-13). Therefore, different designs of expandable cages were developed to address this problem (14). The combination of height and lordosis expansion is technically challenging and has not been addressed until recently. The expandable cages with controlled expansion of both height and lordosis were invented to optimize postoperative alignment by increasing segmental lordosis of the fusion segment (15). Nonetheless, research on this cage design was lacking, especially its effectiveness on spinal alignment restoration. This study aimed to compare the outcomes of two different expandable cages in MIS TLIF: the conventional expandable cages that solely expand disc height, and the newer cages that additionally increase segmental lordosis. In this study, our primary focus was on analyzing the postoperative radiologic outcomes to demonstrate the true mechanical effect of the expandable cages on sagittal parameters without the effect of subsidence over time, and also suggest the technique to prevent early subsidence following the use of expandable cages for MIS TLIF. Our subsequent long-term study in the future will focus on the fusion rate, late subsidence, long-term maintenance of the lordotic alignment, and the effect on the adjacent level. We present this article in accordance with the STROBE reporting checklist (available at https://jss.amegroups.com/article/view/10.21037/jss-23-106/rc).
Methods
Study design and patients
The present study retrospectively reviewed consecutive patients who underwent MIS TLIF with expandable cages at Weill Cornell Medicine, Department of Neurosurgery, New York-Presbyterian Hospital between January 2019 and March 2023. The surgical indications included patients who had degenerative lumbar diseases, presented with radiculopathy and mechanical back pain that did not respond to conservative treatment. Patients who underwent revision surgery at the same level for pseudarthrosis, had non-degenerative pathologies such as spinal tumors, or had incomplete data were excluded from the study. The patients were categorized into two groups based on the types of expandable cages used in their MIS TLIF surgeries: (I) Group H, which used cages that expand only height, and (II) Group HL, which used cages that expand both height and lordosis. All surgeries were performed by a single senior surgeon (R.H.) who had extensive experience in MIS TLIF surgery, and all patients received the same postoperative protocol.
This study was performed in accordance with the ethical standards of the Declaration of Helsinki (as revised in 2013) and was approved by the Weill Cornell Medicine (WCM) Institutional Review Board (IRB) (approval number: 1912021199) and individual consent for this retrospective analysis was waived.
Outcome measures
Demographic characteristics were collected including age, gender, body mass index (BMI), American Society of Anesthesiologists (ASA) score, diagnosis, operated level, baseline pain and disability, and length of hospitalization. Clinical and radiographic outcomes following MIS TLIF were recorded during a three-month visit. Patient-reported outcomes including postoperative Oswestry disability index (ODI) and visual analog scale of back (VAS-B) and leg pain (VAS-L) were collected. Radiographic variables including anterior and posterior disc height (DH), lumbar lordosis (LL), segmental lordosis (SL), disc angle (DA), and vertebral slippage were recorded. Global and regional sagittal parameters consisting of sagittal vertical axis (SVA), pelvic incidence (PI), pelvic tilt (PT) and sacral slope (SS) were measured, and changes between pre- and postoperative values were calculated. Postoperatively, plain radiographs of the lumbar spine and whole spine were taken in a standing position to compare with preoperative images. Additionally, cage subsidence was assessed using computed tomography (CT) scans, which were defined as the presence of at least 2 mm of cage protrusion through the vertebral endplates (16). Clinical outcome measures and complications were documented during each follow-up evaluation.
Types of expandable cages in the study (Figure 1)
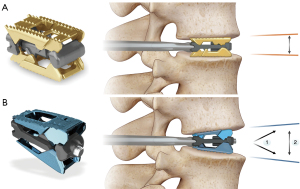
Expandable cage with controlled expansion of height (Group H)
This type of cage is an all-titanium expandable lumbar interbody spacer designed for posterior approaches (RISE®, Globus Medical, Inc. Audubon, PA, USA). The cage is available in three different widths (8, 10 and 12 mm) and three lengths (22, 26, and 30 mm), with fixed 4° lordotic profile. The cage can be inserted at an initial contracted height as low as 7 mm to minimize endplate disruption and impaction forces. The surgeon can then control continuous expansion up to 7 mm to restore disc height and optimize endplate-to-endplate fit.
Expandable cage with controlled expansion of both height and lordosis (Group HL)
The other type of cage is an expandable posterior lumbar interbody spacer with 3D printed titanium endplates (SABLE®, Globus Medical, Inc., Audubon, PA, USA). It is designed to minimize endplate disruption, maximize ability to restore sagittal alignment, optimize fusion with in situ graft delivery, and can be backfilled with bone graft. The cage mechanically expands the lordosis first, followed by an increase in disc height. The cage provides up to 22° of lordosis and continuously expands up to 8 mm. The available widths and lengths are similar to those of the cage used in Group H.
Surgical technique
After informed consent was obtained, all patients were scheduled for navigated MIS TLIF. The surgical procedure was performed according to the standards outlined in the “Step-by-Step Guide: Key Steps in MIS TLIF Procedure” developed by the AO-Spine Minimally Invasive Spine Surgery Task Force (8). The surgery was performed with the patient in a prone position under general anesthesia. Navigation guidance was utilized throughout the procedure, from skin incision to rod placement, to assist with the surgery. At the beginning of the procedure, the intraoperative navigation system (Brainlab AG, Feldkirchen, Germany) was set up, and the reference array was securely placed on the contralateral iliac crest. Image acquisition was then carried out with intraoperative CT scan. Navigator-assisted percutaneous pedicular screw insertion (Viper Prime, DePuy Synthes, Raynham, MA, USA) was performed with 3-dimensional navigation (Brainlab GmbH, Munich, Germany). Afterwards, a separate medial fascial incision was made to place a table-mounted tubular retractor over the facet joint and lamina. Unilateral facetectomy was performed and the resected bone was morselized to be used as autologous bone graft. Ipsilateral and over-the-top decompression of neural structures was thoroughly done under microscope. With protection of the nerve roots, the disc space was identified and discectomy was performed. Meticulous vertebral endplate preparation was carefully done to avoid iatrogenic endplate injury. Bone graft substitute was packed into the prepared disc space anteriorly. An expandable cage with autologous bone graft was implanted under microscope with the aid of navigation guidance. The expansion of the cage was carefully controlled by the surgeon to optimize the endplate-to-endplate fit for an appropriate disc height and lordosis. Intraoperative CT scan was obtained to confirm the accurate placement of pedicular screws, adequate area of decompression, and optimal expansion of the cage. Once the position of all implants was confirmed, bilateral rods were inserted. Any bleeding was carefully inspected and controlled. Subsequently, the surgical wound was closed layer by layer. Postoperative images were obtained to measure radiographic variables (Figure 2).
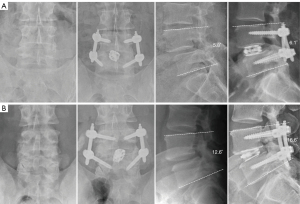
Statistical analysis
Statistical analysis was performed with SPSS 28.0.0 (IBM SPSS, Chicago, IL, USA). Normality was assessed via the Shapiro-Wilk test to determine the appropriate statistical test. In this study, the data followed the normal distribution so that parametric statistical techniques were employed. Standard descriptive statistics were reported for demographic data, perioperative data, functional and radiographic outcomes as means with standard deviations, frequency and percentages, where applicable. Continuous variables between two groups were compared using χ2-sample Student t-test and categorical variables were compared using Chi-square test. Any significant differences between demographic or baseline values were reported. For all analysis, the statistical significance was set at a threshold of P value less than 0.05.
Results
Demographics
The study included a total of 149 patients who underwent MIS TLIF using expandable cages, of which 40 patients received cages that only expanded height (Group H), and 109 patients received cages that expanded both height and lordosis (Group HL) (Figure 3). Baseline demographic characteristics was not different between groups, including average age (64.5±11.2 years), predominantly male gender (85/149, 57%), and average BMI (27.6±5.2 kg/m2). The average length of hospital stay was 2.8±1.6 days. The most frequently operated level was L4–5 (58.4%), followed by L5–S1 (21.5%). The most common diagnosis was spondylolisthesis (57.7%), followed by spinal stenosis (26.2%). These baseline demographics were similar between the two groups (Table 1).
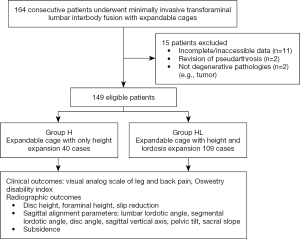
Table 1
Characteristics | Total (n=149) | Types of expandable cages | ||
---|---|---|---|---|
Group H (n=40) | Group HL (n=109) | P value | ||
Age (years) | 64.5±11.2 | 64.9±11.4 | 64.1±11.3 | 0.73 |
Gender (male) | 85 (57.0) | 23 (57.5) | 62 (56.9) | 0.29 |
BMI (kg/m2) | 27.6±5.2 | 27.4±6.3 | 27.7±4.8 | 0.08 |
ASA score | 2.3±0.6 | 2.4±0.5 | 2.3±0.5 | 0.07 |
Length of stay (days) | 2.8±1.6 | 3.1±1.4 | 2.7±1.6 | 0.25 |
Operated level | 0.75 | |||
L2–3 | 6 (4.0) | 2 (5.0) | 4 (3.7) | |
L3–4 | 24 (16.1) | 5 (12.5) | 19 (17.4) | |
L4–5 | 87 (58.4) | 24 (60.0) | 63 (57.8) | |
L5–S1 | 32 (21.5) | 9 (22.5) | 23 (21.1) | |
Diagnosis | 0.17 | |||
Spondylolisthesis | 86 (57.7) | 26 (65.0) | 60 (55.0) | |
Spinal stenosis | 39 (26.2) | 7 (17.5) | 32 (29.4) | |
Degenerative scoliosis | 13 (8.7) | 4 (10.0) | 9 (8.3) | |
Others (ASD, DDD, HNP) | 11 (7.4) | 3 (7.5) | 8 (7.3) | |
ODI | ||||
Preoperative | 41.5±15.0 | 37.4±17.3 | 43.2±12.6 | 0.09 |
Postoperative | 39.6±17.4 | 35.3±16.0 | 41.1±17.8 | 0.27 |
VAS-L | ||||
Preoperative | 7.0±2.3 | 7.4±1.8 | 6.8±2.5 | 0.13 |
Postoperative | 2.4±2.4 | 2.4±1.7 | 2.4±2.5 | 0.99 |
VAS-B | ||||
Preoperative | 7.3±1.9 | 7.6±1.9 | 7.2±1.9 | 0.16 |
Postoperative | 4.1±2.6 | 4.2±2.9 | 4.1±2.5 | 0.95 |
Data are expressed as mean ± standard deviation or frequency (percentages). Group H, expandable cages with only height expansion; Group HL, expandable cages with lordosis and height expansion. BMI, body mass index; ASA, American Society of Anesthesiologists; ASD, adjacent segment disease; DDD, degenerative disc disease; HNP, herniated nucleus pulposus; ODI, Oswestry disability index; VAS-L, visual analog scale of leg pain; VAS-B, visual analog scale of back pain.
Clinical outcomes
Both groups demonstrated significant improvement in pain relief, including both VAS-B and VAS-L at three months following the surgery. However, between Group H and HL, there was no significant difference in postoperative VAS-B (4.2±2.9 and 4.1±2.5, respectively, P=0.95) and VAS-L between both groups (2.4±1.7 and 2.4±2.5, respectively, P=0.99). Similarly, there was no difference in postoperative ODI between Group H and HL (35.3±16.0 and 41.1±17.8, respectively, P=0.27).
Intervertebral disc height, foraminal height, and slip reduction
After surgery, there was a significant improvement in all measured variables, including anterior disc height, posterior disc height, and foraminal height. However, the improvement in these parameters did not differ significantly between the two groups. In Group H and HL, the increases in anterior disc height were 3.3±3.8 and 4.5±3.1 mm, respectively (P=0.21), and the increases in posterior disc height were 3.7±2.8 and 3.0±2.6 mm, respectively (P=0.52). Additionally, there was no significant difference between the groups in changes in foraminal height, which were 2.3±1.7 and 2.1±1.8 mm, respectively (P=0.87). In spondylolisthesis, reduction in vertebral slippage was not different between both types of cages (2.9±2.2 and 2.5±2.0, respectively, P=0.72).
Sagittal alignment and spinopelvic parameters
Group HL had a significantly better increase in segmental lordosis compared to Group H (4.4°±3.5° vs. 2.1°±4.8°, P=0.01). Similarly, Group HL achieved a greater disc angle than Group H (6.3°±3.8° vs. 2.2°±4.3°, P<0.001). However, there was no significant difference in the restoration of lumbar lordosis between the two groups (2.5±4.8 vs. 0.4±5.7, P=0.20, Table 2, Figure 4).
Table 2
Variables | Total (n=149) | Types of expandable cages | ||
---|---|---|---|---|
Group H (n=40) | Group HL (n=109) | P value | ||
Anterior DH (mm) | ||||
Baseline | 9.2±3.9 | 9.4±3.5 | 9.2±4.0 | 0.22 |
Postoperative | 13.4±3.1 | 12.6±2.8 | 13.7±3.1 | 0.39 |
Changes (increase) | 4.2±3.3 | 3.3±3.8 | 4.5±3.1 | 0.21 |
Posterior DH (mm) | ||||
Baseline | 5.0±2.2 | 5.0±2.7 | 4.9±2.0 | 0.05 |
Postoperative | 8.1±2.4 | 8.7±2.2 | 7.9±2.4 | 0.75 |
Changes (increase) | 3.2±2.7 | 3.7±2.8 | 3.0±2.6 | 0.52 |
FH (mm) | ||||
Baseline | 15.7±2.5 | 15.8±3.3 | 15.6±2.2 | 0.43 |
Postoperative | 8.1±2.4 | 18.2±3.4 | 17.9±1.9 | 0.66 |
Changes (increase) | 2.2±1.8 | 2.3±1.7 | 2.1±1.8 | 0.87 |
Lumbar lordosis (°) | ||||
Baseline | 44.8±13.5 | 46.5±13.3 | 44.3±13.5 | 0.37 |
Postoperative | 46.6±12.9 | 46.9±11.7 | 46.5±13.3 | 0.87 |
Changes (increase) | 2.0±5.2 | 0.4±5.7 | 2.5±4.8 | 0.20 |
Segmental lordosis (°) | ||||
Baseline | 16.7±7.0 | 18.0±8.0 | 16.4±6.8 | 0.36 |
Postoperative | 20.5±6.6 | 19.7±6.0 | 20.8±6.8 | 0.33 |
Changes (increase) | 3.9±4.0 | 2.1±4.8 | 4.4±3.5 | 0.01* |
Disc angle (°) | ||||
Baseline | 5.6±4.4 | 5.9±4.2 | 5.5±4.5 | 0.61 |
Postoperative | 10.9±4.6 | 8.1±3.8 | 11.8±4.5 | <0.001* |
Changes (increase) | 5.3±4.3 | 2.2±4.3 | 6.3±3.8 | <0.001* |
Subsidence | 37 (24.8) | 10 (25.0) | 27 (24.8) | 0.83 |
Data are expressed as mean ± standard deviation or frequency (percentages). *, P<0.05. Group H, expandable cages with only height expansion; Group HL, expandable cages with lordosis and height expansion. DH, disc height; FH, foraminal height.
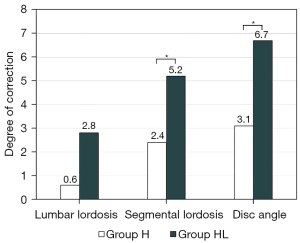
Changes of global sagittal balance as measured by the sagittal vertical axis (SVA) were not significantly different between before and after surgery either in Group H or HL (4.5±13 vs. 6.1±15 mm, P=0.08). Similarly, changes in PT were also not different between both groups (1.8±5.7 vs. −1.4±9.7 mm, P=0.053).
Subsidence and other complications
The incidence subsidence was 24.8%, with 25.0% occurring in Group H and 24.8% in Group HL with no difference between both groups (P=0.83) (Figure 5). After excluding the first 20 cases during the learning phase, the subsidence rates decreased to 20% in Group H and 18.0% in Group HL, showing no significant difference between the groups (P=0.74) (Table 3). Other complications included CSF leak (2.7%), transient urinary retention (2.0%), lung atelectasis (2.0%), hematoma (0.7%), and postoperative myocardial infarction (0.7%).
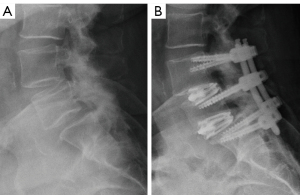
Table 3
Variables | Types of expandable cages | ||
---|---|---|---|
Group H (n=20) | Group HL (n=89) | P value | |
Lumbar lordosis (°) | |||
Baseline | 45.0±11.9 | 45.1±13.1 | 0.95 |
Postoperative | 45.6±10.7 | 47.5±13.2 | 0.54 |
Changes (increase) | 0.6±6.4 | 2.8±4.6 | 0.075 |
Segmental lordosis (°) | |||
Baseline | 17.3±7.6 | 16.2±6.6 | 0.59 |
Postoperative | 19.7±5.9 | 21.3±6.6 | 0.31 |
Changes (increase) | 2.4±3.0 | 5.2±3.2 | 0.003* |
Disc angle (°) | |||
Baseline | 5.0±5.1 | 5.6±4.4 | 0.6 |
Postoperative | 8.0±4.2 | 12.2±4.3 | <0.001* |
Changes (increase) | 3.1±5.9 | 6.7±3.7 | <0.001* |
Subsidence | 4 (20.0) | 16 (18.0) | 0.74 |
Data are expressed as mean ± standard deviation or frequency (percentages). *, P<0.05. Group H, expandable cages with only height expansion; Group HL, expandable cages with lordosis and height expansion.
Discussion
This study indicates that, by utilizing expandable cages that are designed specifically to increase the lordotic angle, there is a significant better improvement of the disc angle and segmental lordosis when compared to the expandable cages that increase only disc height. However, there are no differences in clinical outcomes and other radiographic parameters including lumbar lordosis between both types of expandable cages. Furthermore, with any types of expandable cages, there is always a risk of subsidence when excessive force is applied to increase the disc height or lordosis. Therefore, surgical expertise and a learning curve are essential for optimizing the results of this procedure.
The restoration of spinal sagittal alignment following spinal fusion has been shown to be associated with improved surgical outcomes including improvement of pain and function, and quality of life of the patient postoperatively (17,18). Additionally, achieving optimal sagittal alignment has also been found to alleviate the excessive stress to the adjacent segment that usually leads to accelerated degeneration following the fusion (19,20). Several studies have consistently demonstrated that MIS TLIF offers a minimally invasive procedure that effectively relieve radiculopathy by decompression of neural elements and treat mechanical back pain by stabilizing the unstable segment of the spine, while decreasing blood loss, hospital stay, and complications when compared to open fusion surgery (4,21-25). However, studies have shown that MIS TLIF may not effectively increase segmental lordosis and could not contribute to lordotic sagittal alignment restoration, with some studies even referring the operation as a “kyphosing procedure”. The use of static cages in MIS TLIF limits the capacity of lordotic correction, compared to lateral (LLIF) or anterior lumbar interbody fusion (ALIF) where larger lordotic cages can be inserted (26-28). Therefore, expandable cages designed to expand to increase disc height have been implemented in MIS TLIF (10,14,29). Despite this, several studies still have found no significant difference in the lordosis attained when compared to the use of static cages (12,29-31). This may be due to the fact that, in order to increase disc angle and segmental lordosis, the cage must have a hyperlordotic shape. As a result, a new expandable cage design was developed to increase both height and lordosis (32).
Results of this study have validated the efficacy of the new expandable cage in achieving an increase in segmental lordosis for the fusion segment. These findings indicated that sagittal alignment can be improved with MIS TLIF by utilizing an expandable cage with a specific design to expand both disc height and lordosis. However, for single-level MIS TLIF, we did not observe a significant improvement in overall lumbar lordosis and global sagittal alignment. Furthermore, the extent of improvement in local lordosis did not have a substantial impact on clinical outcomes, which demonstrated no differences between the two types of cages. Therefore, it is important to highlight that although the expandable cage within Group HL led to a statistically significant improvement in segmental lordosis, the meaningful clinical significance of this improvement in terms of overall balance restoration might be limited from one-level MIS TLIF with any type of expandable cage. Nevertheless, the outcomes of this study demonstrated the mechanical advantages of this particular design of expandable cage in terms of restoring sagittal alignment when compared to both static cages and expandable cages that only increase in height. This design holds the potential to create a more favorable and harmonious sagittal alignment, where even a small increase in segmental lordotic angle could potentially lower the risk of adjacent segment degeneration (ASD) (19,33). In addition, we believed that multiple-level surgery will provide a substantial increase in segmental lordosis that could potentially contribute to the restoration of lumbar lordosis, as well as regional and global sagittal alignment.
We observed a higher subsidence rate (25%) compared to earlier studies on expandable TLIF cages, which typically ranged from 15% to 20% in a short-term follow-up (3,12,34). It is important to note that the methodology used to assess the subsidence significantly influences the reported rates. In general, subsidence was defined as a 2-mm loss in either disc height between postoperative and baseline measurements. Notably, we utilized CT scans for subsidence assessment in every patient, while many prior studies relied on standing lateral radiographs (12,34), and some studies used the cut-point of 4-mm loss of disc height as a definition (3), which are less sensitive in detecting subsidence. Furthermore, the expandable cages used in our study demonstrated the capability to increase both intervertebral height and disc lordosis. It is worth emphasizing that an overly aggressive attempt to maximize these parameters as much as possible may contribute to the higher rate of subsidence (35). Therefore, surgeon’s experience, learning curve, and strategies in using the expandable cage are essential to avoid subsidence while achieving optimal lordotic angle.
Following the initial 20 cases, we observed a significant reduction in the rate of subsidence. This improvement coincided with the surgeon’s increased proficiency in determining the optimal force required to tighten the cage expansion device, specifically aimed at increasing disc height and lordosis. These findings highlight the existence of a learning curve regarding optimal torque to be applied to prevent vertebral endplate injury from expandable cages. We have adopted a cautious approach to adjusting the degree of cage expansion. Rather than aiming for maximal expansion, we recommend using the “two-finger” technique, which simply involves using only two fingers to tighten the cage expander instrument for achieving optimal cage expansion. This technique serves as a precaution against excessive torque (36). We suggest applying this technique to reduce the risk of over-distraction force to the vertebral endplate to avoid iatrogenic endplate injury during the expansion of the cages. However, it is crucial to address the considerable variability in absolute torque values, percentage of stripping torque, and challenges in consistently reproducing specific torque levels following this technique (37,38). Therefore, it is important to note that this concept serves as a starting point, particularly crucial in osteoporotic bone, where surgeons may benefit from increased attention to tactile feedback from two fingers instead of palming the cage expander. To further improve precision and prevent overexpansion leading to endplate fractures and early subsidence, the consideration of employing a torque limiting handle attached to the cage inserter may be beneficial.
The 3D-printed Ti cages were used in Group HL, which may deliver an optimal environment for fusion and potentially exhibit significant osseous in-growth from vertebral endplates. Titanium benefits from additive manufacturing which offers the creation of porous implants crucial for spinal fusion. 3D printing has revolutionized the development of biomimetic, porous titanium implants, known for enhancing fusion through osseous integration within their porosity. Early interaction at the implant-bone interface with 3D-printed Ti cages may add stability and limit micromotion, promoting early fusion with stabilization (39). Laratta et al. also found the ingrowth and formation of cartilage and bone to be significantly greater in the 3D-printed Ti cages, compared to PEEK cages (40). The properties of bone biocompatibility of the HL group cage potentially influence long-term changes in vertebral endplates and may improve fusion rate. These long-term effects will be specifically focused in our long-term study.
In this study, short-term clinical outcomes were also reported. At three months post-surgery, leg pain significantly improved due to adequate neural decompression. Although back pain also partially improved, it might require more recovery time due to surgical wound pain at this follow-up period. For changes in ODI, a long-term follow-up may be necessary for assessing improvements in disability.
This study also had several limitations. The limited numbers of subjects in Group H were a result of a transition to utilize a new design of the expandable cage for MIS TLIF that expands both height and lordosis in Group HL. Unequal group sizes can potentially influence the apparent effect size and the precision of estimates, particularly if the smaller group exhibits a more extreme value. However, data in Group H exhibited a normal distribution, and the assumption of equal variances between the two groups was carefully tested. The decision not to equalize group sizes was made with the intention of avoiding the introduction of selection bias and ensuring the inclusion of all available data. This was a short-term study so the pain and disability in long-term follow up, maintenance of sagittal alignment over time, and fusion rates were not reported. In addition, long-term radiographic outcomes and late subsidence after three months were not investigated. Nevertheless, studies on immediate effect on sagittal alignment and short-term clinical outcomes are crucial for gaining a more comprehensive understanding of the mechanical impact of the novel expandable cages on radiographic parameters, unaffected by the influence of subsidence over time. Consequently, a future study involving a randomized trial, a larger sample size, and extended follow-up is necessary to corroborate our findings. Our ongoing efforts are directed towards a long-term study that will focus on the fusion rate, subsidence, the maintenance of the lordotic alignment, and the effect on the adjacent level.
Conclusions
This study suggested that the expandable cages with a design specifically aimed at increasing the lordotic angle has demonstrated favorable clinical outcomes following MIS TLIF and also confirmed the successful immediate effect on the better sagittal alignment, in comparison to conventional expandable cages that expand only height. However, regardless of the type of expandable cage used, it is crucial to avoid applying excessive force to achieve greater disc height or lordosis, as this may contribute to cage subsidence and a subsequent reduction in the restoration of lordotic alignment. Further study is required to investigate the long-term outcomes including the fusion and effect of subsidence over time.
Acknowledgments
Funding: None.
Footnote
Reporting Checklist: The authors have completed the STROBE reporting checklist. Available at https://jss.amegroups.com/article/view/10.21037/jss-23-106/rc
Data Sharing Statement: Available at https://jss.amegroups.com/article/view/10.21037/jss-23-106/dss
Peer Review File: Available at https://jss.amegroups.com/article/view/10.21037/jss-23-106/prf
Conflicts of Interest: All authors have completed the ICMJE uniform disclosure form (available at https://jss.amegroups.com/article/view/10.21037/jss-23-106/coif). The authors have no conflicts of interest to declare.
Ethical Statement:
Open Access Statement: This is an Open Access article distributed in accordance with the Creative Commons Attribution-NonCommercial-NoDerivs 4.0 International License (CC BY-NC-ND 4.0), which permits the non-commercial replication and distribution of the article with the strict proviso that no changes or edits are made and the original work is properly cited (including links to both the formal publication through the relevant DOI and the license). See: https://creativecommons.org/licenses/by-nc-nd/4.0/.
References
- Liu J, Duan P, Mummaneni PV, et al. Does transforaminal lumbar interbody fusion induce lordosis or kyphosis? Radiographic evaluation with a minimum 2-year follow-up. J Neurosurg Spine 2021;35:419-26. [Crossref] [PubMed]
- Cheng X, Zhang F, Zhang K, et al. Effect of Single-Level Transforaminal Lumbar Interbody Fusion on Segmental and Overall Lumbar Lordosis in Patients with Lumbar Degenerative Disease. World Neurosurg 2018;109:e244-51. [Crossref] [PubMed]
- Gelfand Y, Benton J, De la Garza-Ramos R, et al. Effect of Cage Type on Short-Term Radiographic Outcomes in Transforaminal Lumbar Interbody Fusion. World Neurosurg 2020;141:e953-8. [Crossref] [PubMed]
- Lener S, Wipplinger C, Hernandez RN, et al. Defining the MIS-TLIF: A Systematic Review of Techniques and Technologies Used by Surgeons Worldwide. Global Spine J 2020;10:151S-67S. [Crossref] [PubMed]
- Tan JH, Liu G, Ng R, et al. Is MIS-TLIF superior to open TLIF in obese patients?: A systematic review and meta-analysis. Eur Spine J 2018;27:1877-86. [Crossref] [PubMed]
- Dave BR, Marathe N, Mayi S, et al. Does Conventional Open TLIF cause more Muscle Injury when Compared to Minimally Invasive TLIF?-A Prospective Single Center Analysis. Global Spine J 2024;14:93-100. [Crossref] [PubMed]
- Kuhta M, Bošnjak K, Vengust R. Failure to maintain segmental lordosis during TLIF for one-level degenerative spondylolisthesis negatively affects clinical outcome 5 years postoperatively: a prospective cohort of 57 patients. Eur Spine J 2019;28:745-50. [Crossref] [PubMed]
- Wipplinger C, Melcher C, Hernandez RN, et al. "One and a half" minimally invasive transforaminal lumbar interbody fusion: single level transforaminal lumbar interbody fusion with adjacent segment unilateral laminotomy for bilateral decompression for spondylolisthesis with bisegmental stenosis. J Spine Surg 2018;4:780-6. [Crossref] [PubMed]
- Prabhu MC, Jacob KC, Patel MR, et al. History and Evolution of the Minimally Invasive Transforaminal Lumbar Interbody Fusion. Neurospine 2022;19:479-91. [Crossref] [PubMed]
- Alimi M, Shin B, Macielak M, et al. Expandable Polyaryl-Ether-Ether-Ketone Spacers for Interbody Distraction in the Lumbar Spine. Global Spine J 2015;5:169-78. [Crossref] [PubMed]
- Armocida D, Pesce A, Cimatti M, et al. Minimally Invasive Transforaminal Lumbar Interbody Fusion Using Expandable Cages: Increased Risk of Late Postoperative Subsidence Without a Real Improvement of Perioperative Outcomes: A Clinical Monocentric Study. World Neurosurg 2021;156:e57-63. [Crossref] [PubMed]
- Canseco JA, Karamian BA, DiMaria SL, et al. Static Versus Expandable Polyether Ether Ketone (PEEK) Interbody Cages: A Comparison of One-Year Clinical and Radiographic Outcomes for One-Level Transforaminal Lumbar Interbody Fusion. World Neurosurg 2021;152:e492-501. [Crossref] [PubMed]
- Basani V, Jitpakdee K, Härtl R. Complications of Minimally Invasive Transforaminal Lumbar Interbody Fusion. J Minim Invasive Spine Surg Tech 2023;8:S15-28.
- Chang CC, Chou D, Pennicooke B, et al. Long-term radiographic outcomes of expandable versus static cages in transforaminal lumbar interbody fusion. J Neurosurg Spine 2020;34:471-80. [Crossref] [PubMed]
- Tan LA, Rivera J, Tan XA, et al. Clinical and Radiographic Outcomes After Minimally Invasive Transforaminal Lumbar Interbody Fusion-Early Experience Using a Biplanar Expandable Cage for Lumbar Spondylolisthesis. Int J Spine Surg 2020;14:S39-44. [Crossref] [PubMed]
- Pisano AJ, Fredericks DR, Steelman T, et al. Lumbar disc height and vertebral Hounsfield units: association with interbody cage subsidence. Neurosurg Focus 2020;49:E9. [Crossref] [PubMed]
- Le Huec JC, Thompson W, Mohsinaly Y, et al. Sagittal balance of the spine. Eur Spine J 2019;28:1889-905. [Crossref] [PubMed]
- Kouyoumdjian P, Mansour J, Haignère V, et al. Hip-Spine Relationship between Sagittal Balance of the Lumbo-Pelvi-Femoral Complex and Hip Extension Capacity: An EOS Evaluation in a Healthy Caucasian Population. Global Spine J 2024;14:265-71. [Crossref] [PubMed]
- Yamasaki K, Hoshino M, Omori K, et al. Risk Factors of Adjacent Segment Disease After Transforaminal Inter-Body Fusion for Degenerative Lumbar Disease. Spine (Phila Pa 1976) 2017;42:E86-92. [Crossref] [PubMed]
- Sun J, Wang JJ, Zhang LW, Huang H, Fu NX. Sagittal Alignment as Predictor of Adjacent Segment Disease After Lumbar Transforaminal Interbody Fusion. World Neurosurg. 2018;110:e567-e71. [Crossref] [PubMed]
- Woodward J, Koro L, Richards D, et al. Expandable versus Static Transforaminal Lumbar Interbody Fusion Cages: 1-year Radiographic Parameters and Patient-Reported Outcomes. World Neurosurg 2022;159:e1-7. [Crossref] [PubMed]
- Li YM, Frisch RF, Huang Z, et al. Comparative Effectiveness of Expandable Versus Static Interbody Spacers via MIS LLIF: A 2-Year Radiographic and Clinical Outcomes Study. Global Spine J 2020;10:998-1005. [Crossref] [PubMed]
- Lian X, Navarro-Ramirez R, Berlin C, Jada A, Moriguchi Y, Zhang Q, et al. Total 3D Airo(R) Navigation for Minimally Invasive Transforaminal Lumbar Interbody Fusion. Biomed Res Int. 2016;2016:5027340. [Crossref] [PubMed]
- Torres J, James AR, Alimi M, et al. Screw placement accuracy for minimally invasive transforaminal lumbar interbody fusion surgery: a study on 3-d neuronavigation-guided surgery. Global Spine J 2012;2:143-52. [Crossref] [PubMed]
- Parajón A, Alimi M, Navarro-Ramirez R, et al. Minimally Invasive Transforaminal Lumbar Interbody Fusion: Meta-analysis of the Fusion Rates. What is the Optimal Graft Material? Neurosurgery 2017;81:958-71. [Crossref] [PubMed]
- Rabau O, Navarro-Ramirez R, Aziz M, et al. Lateral Lumbar Interbody Fusion (LLIF): An Update. Global Spine J 2020;10:17S-21S. [Crossref] [PubMed]
- Sinkov V, Lockey SD, Cunningham BW. Single Position Lateral Lumbar Interbody Fusion With Posterior Instrumentation Utilizing Computer Navigation and Robotic Assistance: Retrospective case review and surgical technique considerations. Global Spine J 2022;12:75S-81S. [Crossref] [PubMed]
- Yang H, Liu J, Hai Y, et al. What Are the Benefits of Lateral Lumbar Interbody Fusion on the Treatment of Adult Spinal Deformity: A Systematic Review and Meta-Analysis Deformity. Global Spine J 2023;13:172-87. [Crossref] [PubMed]
- Vaishnav AS, Saville P, McAnany S, et al. Retrospective Review of Immediate Restoration of Lordosis in Single-Level Minimally Invasive Transforaminal Lumbar Interbody Fusion: A Comparison of Static and Expandable Interbody Cages. Oper Neurosurg (Hagerstown) 2020;18:518-23. [Crossref] [PubMed]
- Yee TJ, Joseph JR, Terman SW, et al. Expandable vs Static Cages in Transforaminal Lumbar Interbody Fusion: Radiographic Comparison of Segmental and Lumbar Sagittal Angles. Neurosurgery 2017;81:69-74. [Crossref] [PubMed]
- Stein IC, Than KD, Chen KS, et al. Failure of a polyether-ether-ketone expandable interbody cage following transforaminal lumbar interbody fusion. Eur Spine J 2015;24:S555-9. [Crossref] [PubMed]
- Park DY, Heo DH. The Use of Dual Direction Expandable Titanium Cage With Biportal Endoscopic Transforaminal Lumbar Interbody Fusion: A Technical Consideration With Preliminary Results. Neurospine 2023;20:110-8. [Crossref] [PubMed]
- Dong S, Li J, Jia X, et al. Analysis of Risk Factors for Adjacent Segment Degeneration after Minimally Invasive Transforaminal Interbody Fusion at Lumbosacral Spine. Comput Intell Neurosci 2022;2022:4745534. [Crossref] [PubMed]
- Lawless MH, Claus CF, Tong D, et al. Radiographic and Patient-Reported Outcomes of Lordotic Versus Non-lordotic Static Interbody Devices in Minimally Invasive Transforaminal Lumbar Interbody Fusion: A Longitudinal Comparative Cohort Study. Cureus 2022;14:e21273. [Crossref] [PubMed]
- Stickley C, Philipp T, Wang E, et al. Expandable cages increase the risk of intraoperative subsidence but do not improve perioperative outcomes in single level transforaminal lumbar interbody fusion. Spine J 2021;21:37-44. [Crossref] [PubMed]
- Seng DW, Chou SM, Liang BZ, et al. Revising a loosened cancellous screw with a larger screw does not restore original pull-out strength - A biomechanical study. Clin Biomech (Bristol, Avon) 2021;89:105460. [Crossref] [PubMed]
- Acker WB 2nd, Tai BL, Belmont B, et al. Two-Finger Tightness: What Is It? Measuring Torque and Reproducibility in a Simulated Model. J Orthop Trauma 2016;30:273-7. [Crossref] [PubMed]
- Wilkofsky IG, Werner FW, Setter KJ. How repeatable is two-finger tightness when used to tighten bone screws? J Hand Surg Eur Vol 2014;39:1007-8. [Crossref] [PubMed]
- Wilcox B, Mobbs RJ, Wu AM, et al. Systematic review of 3D printing in spinal surgery: the current state of play. J Spine Surg 2017;3:433-43. [Crossref] [PubMed]
- Laratta JL, Vivace BJ, López-Peña M, et al. 3D-printed titanium cages without bone graft outperform PEEK cages with autograft in an animal model. Spine J 2022;22:1016-27. [Crossref] [PubMed]