Robotic-assisted spine surgery—a narrative review
Introduction
In recent years, data has continued to emerge supporting operative treatment of various spinal pathologies to improve patient pain and function (1-3). Pedicle screw instrumentation remains the mainstay of spinal fusion, providing rigid three-column fixation. Improper pedicle screw placement can lead to increased complications and the need for revision surgery. The success of spinal fusion depends on accurate pedicle screw placement to achieve adequate fixation and to avoid injury to surrounding structures (4).
The traditional freehand technique relies on anatomic landmarks and knowledge of pedicle trajectory to accurately place pedicle screws. However, this technique can become even more difficult with patients with significant deformity, revision surgery, and overall altered anatomy. Emerging technologies have increasingly been adopted in spine surgery in the attempt to increase precision and improve outcomes (5,6). Robotic assistance is an area of significant interest, with proposed benefits including increased accuracy of pedicle screw placement, decreased complication rates, and decreased radiation exposure (4-6).
As robotic assistance continues to become increasingly popular in spine surgery, it is critical for surgeons to understand the technology available and the associated outcomes to make informed decisions when considering which system to incorporate into their practice. The goal of this review is to provide an overview of the currently available robotic assistance systems and their associated outcomes and limitations. We present this article in accordance with the Narrative Review reporting checklist (available at https://jss.amegroups.com/article/view/10.21037/jss-23-40/rc).
Methods
A review of national databases (PubMed and Scopus) was performed using literature from 2014 to 2023. Keywords included terms “robotic”, “spine”, and “surgery”. Studies that aimed to describe the types of robotic devices, clinical and radiological outcomes, limitations, and future directions were included. Studies unavailable in English were excluded (Table 1).
Table 1
Items | Specification |
---|---|
Date of search | February 1, 2023–February 7, 2024 |
Databases and other sources searched | PubMed and Scopus |
Search terms used | “Robotic”, “spine”, and “surgery” |
Timeframe | 2014 to 2023 |
Inclusion criteria | English language; PubMed-indexed journal |
Selection process | Conducted by all authors independently; all sources reviewed and selected by senior author (H.M.) |
Types of robotic devices
Robotic-assisted spine surgery emerged with the first spine robot, the SpineAssist (Mazor Robotics Ltd., Caesarea, Israel), in 2004 (7,8). Since then, numerous technical advancements have produced fundamental changes in spine surgery practice (9). Existing surgical robots typically fall into three categories: supervisory-controlled, telesurgical, and shared-control. Supervisory-controlled robots allow the surgeon to plan the operation pre-operatively, then the robot performs the operation under close supervision. Telesurgical robots allow the surgeon to directly control the robot and its instruments during the operation, essentially acting as an extension of the surgeon’s own arms. Spine surgery robots fall under the third category, shared-control robots, which simultaneously allow both the surgeon and robot participate in the operation (7,8). Spine surgery has also benefited from computer-assisted navigation (CAN) (7,10). Stereotactic navigation systems using computed tomography (CT) or pulsed fluoroscopic images, obtained pre-operatively or intra-operatively, generate both two-dimensional (2D) and three-dimensional (3D) projections of the anatomy throughout the case (11). CAN is now widely used in many spinal procedures and can be integrated with currently available spine robot platforms.
There are four spine surgery robots that are most commonly utilized and well-studied. As mentioned, Mazor released the SpineAssist (Mazor Robotics Ltd.) in 2004, which became the first Food and Drug Administration (FDA)-approved spine robot and remains widely used. It is a shared-control robot that offers navigation superior to traditional intraoperative CAN (7). The SpineAssist automatically positions its arm along a pre-determined trajectory, then all subsequent drilling is performed by the surgeon (11). Mazor’s second-generation robot, the Renaissance, was released in 2011, which was similar to Mazor with some software and hardware improvements. However, both robots were criticized for incorrect screw trajectory secondary to skiving potential. To address this, a newer robot, the Mazor X, released in 2016, includes a camera that allows the robot to assess the work environment and self-detect its location intra-operatively. It also allows registration of each individual vertebral body to improve accuracy as well as preoperative planning (Figures 1,2). The ROSA Spine also became FDA-approved in 2016 and functions similarly to the Mazor X as it also has a stereoscopic camera (12). Both the Mazor X and ROSA have not been widely validated for use in pedicle instrumentation due to their relative novelty and lack of data. The third most commonly utilized robotic system is the Da Vinci Surgical System, which was initially released in 2000 for general laparoscopic procedures. Contrary to the Mazor and ROSA systems, the Da Vinci Surgical System follows the telesurgical model, meaning the surgeon controls the robot from a remote booth equipped with 3D vision during the operation (7). In spine surgery, the Da Vinci robot has been utilized in anterior lumbar interbody fusions (ALIFs) with limited but promising results. A few case series have demonstrated successful exposure and interbody placement without complications (13). However, its role in posterior based instrumentation has yet to considered. Finally, the Excelsius GPS (Globus Medical, Inc., Audubon, PA, USA) is a recent spine robot released in 2017. It features real-time intraoperative imaging, automatic adjustment for patient movement, and direct screw insertion without guidewires. The robot provides immediate feedback if the drill skives or reference frame moves (7,14). Further research pertaining to its intra-operative accuracy is required but early results suggest precise execution and accurate screw placement (15). The recent growth and widespread integration of Excelsius GPS suggests excellent consistency and may lead to expansion to other indications, including robotic interbody placement.
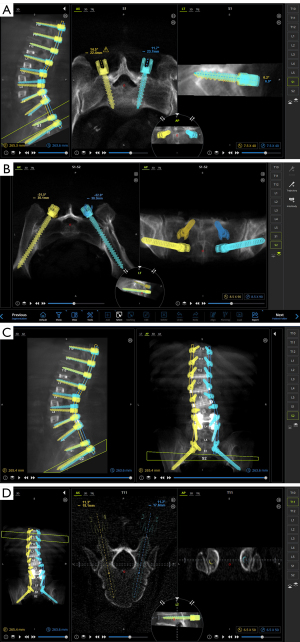
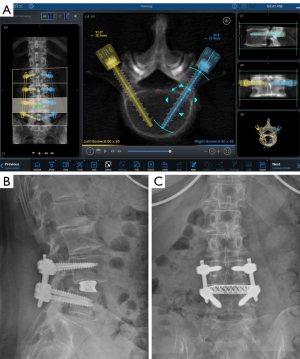
Outcomes
Outcomes important to spine procedures include pedicle screw accuracy, radiation exposure, patient-reported outcomes and complications. Pedicle screw accuracy is one of the major benefits of robotic surgery compared to free hand. In Gao et al.’s meta-analysis of randomized controlled studies, robotic assistance had superior accuracy and consistency of placement of screws and fewer proximal facet joint violations (16). This study found that when accuracy was expanded to include both Grade A and Grade B in the Gerztbein-Robbins classification (both considered to be satisfactory operative results), there was no significant difference between techniques (P=0.71, I2=0%). This difference is likely because robots lack the ability to make manual errors that may occur secondary to fatigue or oversight in repetitive tasks.
Additionally, robotic assistance may decrease radiation exposure in surgical candidates. In Gao et al.’s meta-analysis of randomized control trials, it was demonstrated that robotic assistance significantly reduced radiation time (mean difference of 12.4 seconds per screw) for the patient and surgical staff (16). Hyun et al. found in a prospective randomized controlled study that robotic assistance resulted in an almost 10 seconds difference of radiation per screw (3.5 vs. 13.3 seconds, P=0.015) (17). It was hypothesized that this difference was observed because placement of screws relied on the robotics’ utilization of the preoperative CT scan rather than the surgeon requiring multiple views to interpret placement intraoperatively.
To date, there appears to be no difference in patient-reported outcomes between freehand and robotic treatment. In a randomized control trial, Park et al. found that there was no difference in both visual analogue scale (VAS) and Oswestry disability index (ODI) after surgery at a minimum follow-up of 2 years (18). In a larger matched cohort study, similar results were found as there were no differences between robotic or free-hand patients that were able to achieve the minimal clinically important difference in VAS, ODI, and Short Form-12 Health Surveys at the 1-year follow-up (19).
Complications are relatively rare within both groups. The majority of studies demonstrate little to no complications using robotic technology (16,17). In a recent multicenter prospective study looking at minimally invasive procedures of 400 patients, the MIS ReFRESH Prospective Comparative Study found that the complication rate of free hand pedicle screw insertion was 5.8 times higher [95% confidence interval (CI): 3.5–9.6; P<0.001] and revision rate was 11.0 times higher (95% CI: 2.9–41.2; P<0.001) when compared to robot-guided placement (20). However, complications such as cannula skidding, skiving from soft tissue or technical issues such as registration issues resulting in robot abandonment have been observed and thus may bias results (21). Keric et al. reported complications included conversion to freehand secondary to registration failure (1.9%, n=6), dural tears (6.4%, n=26), screw misplacement (0.48%, n=9 screws), and infection requiring revision (4.9%, n=20) (22). Ultimately, however, it does not appear that these complications are significant, with some studies finding that robotic studies show shorter length of stay; Kantelhardt et al. found an average decrease of 3 days when compared with free hand technique (11.6 for open robotic vs. 14.6 days, P<0.05) (23).
Limitations
Robotic spine surgery is not without significant limitations, which include requiring a significant learning curve, possibly longer operative cases, and significant associated costs. Hu et al. found that the rate of successful pedicle screw placement (82% to >90%) increased while the frequency of conversion from robotic to manual screw placement (17% to 4%) decreased after the first 30 procedures performed by each surgeon (24). A recent systematic review found similar results (25). With this learning curve, operative times expected are longer and generally trend down as more cases are performed (26).
Independent of learning curves, the effect of utilizing robotic assistance has on operative times when compared to free hand technique is unclear. In a prospective single surgeon study, Lonjon et al. found that operative timing for robotic cases (336 minutes) was significantly longer than freehand (226 minutes; P<0.001) (27). Other studies have found no significant differences in duration of surgery when compared to free hand (23) or fluoroscopic assisted screws (28). A recent systematic review and meta-analysis of randomized controlled trials of robotic and freehand studies found that robotic cases were significantly longer (160.9 minutes; 95% CI: 32.37–289.50; P=0.014) (29). This is likely secondary to the intraoperative preparation required to register landmarks and position the assistant.
Finally, a significant barrier for adoption of robotic assistants are the costs associated with the technology. First, the fixed costs of a spinal robot ranges from $500,000 to $1,200,000, which does not include the yearly maintenance, navigation software, or disposable equipment required per case (7). There are conflicting reports of both the spinal robot’s cost-effectiveness (30,31) and lack thereof (32,33) when compared to freehand techniques. Menger et al. predicted savings from operating room (OR) time utilization and fewer costs associated with prolonged length of stay (infections, revisions), with an ultimate cost savings of roughly $608,546 over a 1 year period (30). Conversely, in a retrospective review, Ezeokoli et al. found 11% higher day-of-surgery and 16% higher total encounter costs for patients that underwent robotic surgery when compared to freehand procedures; the main factor that drove costs up was OR time ($1,521 vs. $880, P<0.001) and OR surgical supplies ($11,367 vs. $9,931, P=0.018) (33).
Future directions
There remains great potential for growth in robotic spine surgery. A current drawback to current robotic devices is the capability to reliably measure screw depth, unlike CAN, which provides improved visualization during screw placement; this drawback has limited the use of robots in the cervical spine. One study comparing the O-arm navigation system to robotic assistance demonstrated that the robot significantly reduced fluoroscopy and time per screw despite similar pedicle screw accuracy (34). The Mazor X Stealth Edition (Mazor Robotics Ltd.) has been developed and combines use of robotics with intraoperative navigation, but further biomechanical and clinical data is necessary prior to its safe implementation in the cervical spine.
There are possible applications of robotic assistance in addition to pedicle screw placement, including tumor resection and osteotomies for spinal deformity correction. The use of robots for burrs or saws may eventually enable safer decompression or osteotomy. Navigation using preoperative magnetic resonance imaging may eventually be useful in additional robotic spinal procedures including discectomy or mobilization of neural elements. Overall, robotic-assisted spine surgery is likely to continue to become more routinely used for a variety of expanding purposes, although further software development and higher-quality studies are necessary to forge growth and demonstrate its safety, effectiveness, and value.
Conclusions
Robotic-assisted spine surgery has seen increasing use in the attempt to increase precision and improve outcomes and has been associated with increased accuracy in pedicle screw placement and decreased complication rates. Barriers to its adoption include a significant learning curve, possibly longer operative cases, and significant associated costs. As robotic assistance continues to become increasingly popular in spine surgery, it is critical for surgeons to understand the technology available and the associated outcomes to make informed decisions when considering which system to incorporate into their practice.
Acknowledgments
Funding: None.
Footnote
Provenance and Peer Review: This article was commissioned by the Guest Editor (Cameron Kia) for the series “Minimally Invasive Techniques in Spine Surgery and Trend Toward Ambulatory Surgery” published in Journal of Spine Surgery. The article has undergone external peer review.
Reporting Checklist: The authors have completed the Narrative Review reporting checklist. Available at https://jss.amegroups.com/article/view/10.21037/jss-23-40/rc
Peer Review File: Available at https://jss.amegroups.com/article/view/10.21037/jss-23-40/prf
Conflicts of Interest: All authors have completed the ICMJE uniform disclosure form (available at https://jss.amegroups.com/article/view/10.21037/jss-23-40/coif). The series “Minimally Invasive Techniques in Spine Surgery and Trend Toward Ambulatory Surgery” was commissioned by the editorial office without any funding or sponsorship. The authors have no other conflicts of interest to declare.
Ethical Statement: The authors are accountable for all aspects of the work in ensuring that questions related to the accuracy or integrity of any part of the work are appropriately investigated and resolved. All clinical procedures described in this study were performed in accordance with the ethical standards of the institutional and/or national research committee(s) and with the Helsinki Declaration (as revised in 2013). Written informed consent was obtained from the patients for the publication of this article and accompanying images.
Open Access Statement: This is an Open Access article distributed in accordance with the Creative Commons Attribution-NonCommercial-NoDerivs 4.0 International License (CC BY-NC-ND 4.0), which permits the non-commercial replication and distribution of the article with the strict proviso that no changes or edits are made and the original work is properly cited (including links to both the formal publication through the relevant DOI and the license). See: https://creativecommons.org/licenses/by-nc-nd/4.0/.
References
- Abdu WA, Sacks OA, Tosteson ANA, et al. Long-Term Results of Surgery Compared With Nonoperative Treatment for Lumbar Degenerative Spondylolisthesis in the Spine Patient Outcomes Research Trial (SPORT). Spine (Phila Pa 1976) 2018;43:1619-30. [Crossref] [PubMed]
- Oster BA, Kikanloo SR, Levine NL, et al. Systematic Review of Outcomes Following 10-year Mark of Spine Patient Outcomes Research Trial (SPORT) for Spinal Stenosis. Spine (Phila Pa 1976) 2020;45:832-6. [Crossref] [PubMed]
- Oster BA, Kikanloo SR, Levine NL, et al. Systematic Review of Outcomes Following 10-Year Mark of Spine Patient Outcomes Research Trial (SPORT) for Degenerative Spondylolisthesis. Spine (Phila Pa 1976) 2020;45:820-4. [Crossref] [PubMed]
- Satin AM, Kisinde S, Lieberman IH. Can Robotic Spine Surgery Become the Standard of Care? Int J Spine Surg 2022;16:S44-9. [Crossref] [PubMed]
- Lee NJ, Leung E, Buchanan IA, et al. A multicenter study of the 5-year trends in robot-assisted spine surgery outcomes and complications. J Spine Surg 2022;8:9-20. [Crossref] [PubMed]
- Li HM, Zhang RJ, Shen CL. Accuracy of Pedicle Screw Placement and Clinical Outcomes of Robot-assisted Technique Versus Conventional Freehand Technique in Spine Surgery From Nine Randomized Controlled Trials: A Meta-analysis. Spine (Phila Pa 1976) 2020;45:E111-9. [Crossref] [PubMed]
- D'Souza M, Gendreau J, Feng A, et al. Robotic-Assisted Spine Surgery: History, Efficacy, Cost, And Future Trends. Robot Surg 2019;6:9-23. [Crossref] [PubMed]
- Kia C, Esmende S. Robotic-assisted Spine Surgery: A Review of its Development, Outcomes, and Economics on Practice. Tech Orthop 2021;36:272-6. [Crossref]
- Rasouli JJ, Shao J, Neifert S, et al. Artificial Intelligence and Robotics in Spine Surgery. Global Spine J 2021;11:556-64. [Crossref] [PubMed]
- Tjardes T, Shafizadeh S, Rixen D, et al. Image-guided spine surgery: state of the art and future directions. Eur Spine J 2010;19:25-45. [Crossref] [PubMed]
- Ringel F, Stüer C, Reinke A, et al. Accuracy of robot-assisted placement of lumbar and sacral pedicle screws: a prospective randomized comparison to conventional freehand screw implantation. Spine (Phila Pa 1976) 2012;37:E496-501. [Crossref] [PubMed]
- Overley SC, Cho SK, Mehta AI, et al. Navigation and Robotics in Spinal Surgery: Where Are We Now? Neurosurgery 2017;80:S86-99. [Crossref] [PubMed]
- Lee JY, Bhowmick DA, Eun DD, et al. Minimally invasive, robot-assisted, anterior lumbar interbody fusion: a technical note. J Neurol Surg A Cent Eur Neurosurg 2013;74:258-61. [Crossref] [PubMed]
- Zygourakis CC, Ahmed AK, Kalb S, et al. Technique: open lumbar decompression and fusion with the Excelsius GPS robot. Neurosurg Focus 2018;45:V6. [Crossref] [PubMed]
- Jiang B, Karim Ahmed A, Zygourakis CC, et al. Pedicle screw accuracy assessment in ExcelsiusGPS® robotic spine surgery: evaluation of deviation from pre-planned trajectory. Chin Neurosurg J 2018;4:23. [Crossref] [PubMed]
- Gao S, Lv Z, Fang H. Robot-assisted and conventional freehand pedicle screw placement: a systematic review and meta-analysis of randomized controlled trials. Eur Spine J 2018;27:921-30. [Crossref] [PubMed]
- Hyun SJ, Kim KJ, Jahng TA, et al. Minimally Invasive Robotic Versus Open Fluoroscopic-guided Spinal Instrumented Fusions: A Randomized Controlled Trial. Spine (Phila Pa 1976) 2017;42:353-8. [Crossref] [PubMed]
- Park SM, Kim HJ, Lee SY, et al. Radiographic and Clinical Outcomes of Robot-Assisted Posterior Pedicle Screw Fixation: Two-Year Results from a Randomized Controlled Trial. Yonsei Med J 2018;59:438-44. [Crossref] [PubMed]
- Karamian BA, DiMaria SL, Sawires AN, et al. Clinical Outcomes of Robotic Versus Freehand Pedicle Screw Placement After One-to Three-Level Lumbar Fusion. Global Spine J 2023;13:1871-7. [Crossref] [PubMed]
- Good CR, Orosz L, Schroerlucke SR, et al. Complications and Revision Rates in Minimally Invasive Robotic-Guided Versus Fluoroscopic-Guided Spinal Fusions: The MIS ReFRESH Prospective Comparative Study. Spine (Phila Pa 1976) 2021;46:1661-8. [Crossref] [PubMed]
- Hu X, Ohnmeiss DD, Lieberman IH. Robotic-assisted pedicle screw placement: lessons learned from the first 102 patients. Eur Spine J 2013;22:661-6. [Crossref] [PubMed]
- Keric N, Doenitz C, Haj A, et al. Evaluation of robot-guided minimally invasive implantation of 2067 pedicle screws. Neurosurg Focus 2017;42:E11. [Crossref] [PubMed]
- Kantelhardt SR, Martinez R, Baerwinkel S, et al. Perioperative course and accuracy of screw positioning in conventional, open robotic-guided and percutaneous robotic-guided, pedicle screw placement. Eur Spine J 2011;20:860-8. [Crossref] [PubMed]
- Hu X, Lieberman IH. What is the learning curve for robotic-assisted pedicle screw placement in spine surgery? Clin Orthop Relat Res 2014;472:1839-44. [Crossref] [PubMed]
- Pennington Z, Judy BF, Zakaria HM, et al. Learning curves in robot-assisted spine surgery: a systematic review and proposal of application to residency curricula. Neurosurg Focus 2022;52:E3. [Crossref] [PubMed]
- Urakov TM, Chang KH, Burks SS, et al. Initial academic experience and learning curve with robotic spine instrumentation. Neurosurg Focus 2017;42:E4. [Crossref] [PubMed]
- Lonjon N, Chan-Seng E, Costalat V, et al. Robot-assisted spine surgery: feasibility study through a prospective case-matched analysis. Eur Spine J 2016;25:947-55. [Crossref] [PubMed]
- Solomiichuk V, Fleischhammer J, Molliqaj G, et al. Robotic versus fluoroscopy-guided pedicle screw insertion for metastatic spinal disease: a matched-cohort comparison. Neurosurg Focus 2017;42:E13. [Crossref] [PubMed]
- Tarawneh AM, Salem KM. A Systematic Review and Meta-analysis of Randomized Controlled Trials Comparing the Accuracy and Clinical Outcome of Pedicle Screw Placement Using Robot-Assisted Technology and Conventional Freehand Technique. Global Spine J 2021;11:575-86. [Crossref] [PubMed]
- Menger RP, Savardekar AR, Farokhi F, et al. A Cost-Effectiveness Analysis of the Integration of Robotic Spine Technology in Spine Surgery. Neurospine 2018;15:216-24. [Crossref] [PubMed]
- Garcia D, Akinduro OO, De Biase G, et al. Robotic-Assisted vs Nonrobotic-Assisted Minimally Invasive Transforaminal Lumbar Interbody Fusion: A Cost-Utility Analysis. Neurosurgery 2022;90:192-8. [Crossref] [PubMed]
- Passias PG, Brown AE, Alas H, et al. A cost benefit analysis of increasing surgical technology in lumbar spine fusion. Spine J 2021;21:193-201. [Crossref] [PubMed]
- Ezeokoli EU, Pfennig M, John J, et al. Index Surgery Cost of Fluoroscopic Freehand Versus Robotic-Assisted Pedicle Screw Placement in Lumbar Instrumentation: An Age, Sex, and Approach-Matched Cohort Comparison. J Am Acad Orthop Surg Glob Res Rev 2022;6:e22.00137.
- Fan Y, Du J, Zhang J, et al. Comparison of Accuracy of Pedicle Screw Insertion Among 4 Guided Technologies in Spine Surgery. Med Sci Monit 2017;23:5960-8. [Crossref] [PubMed]