Surgical technique of 3D computer-assisted navigated posterior fixation of the upper cervical spine: illustration of three cases
Highlight box
Key findings
• High cervical screws, including C1 lateral mass screws with the notching technique, C2 and C3 pars screws, and translaminar screws, can be safely placed using computer-assisted navigation (CAN) systems featuring automatic image registration with intraoperative computed tomography (iCT) and preoperative magnetic resonance imaging (MRI) in patients with variable pathologies.
What is conventional, and what is novel/modified?
• Intraoperative three-dimensional imaging techniques such as iCT have enabled CAN to integrate real-time imaging into navigation, leading to benefits such as enhanced screw accuracy, reduced perioperative risks, and postsurgical screw placement confirmation. Although CAN and imaging are increasingly used in lumbar and thoracic surgeries, their adoption in cervical spine procedures remains limited.
• The novelty of our presented cases is that posterior fixation of the high cervical spine can be accomplished safely and accurately using CAN systems featuring automatic image registration with iCT and preoperative MRI. This approach proves to be a safe and precise solution for cervical spine procedures, even when employing various surgical strategies such as lateral mass, translaminar, or pars screws to accommodate the variable anatomy of patients.
What is the implication, and what should change now?
• The utilization of CAN systems, incorporating automatic image registration with iCT and preoperative MRI, is both safe and feasible for high cervical surgery. Consequently, its implementation can enhance the precision of cervical screws and ensure safety.
Introduction
Surgical instrumentation of the high cervical spine can be challenging due to the highly mobile craniovertebral junction, the delicate bony structure, and the proximity of vertebral and internal carotid arteries and nerve roots (1). Further, a relatively high rate of non-unions up to 20% can be observed in such procedures, potentially as a result of higher mechanical stress at the graft-body interface (1,2). Consequently, numerous posterior fusion techniques have been developed, such as cervical pedicle screws, cervical pars screws, cervical lateral mass screws, and translaminar screws (3). Each technique represents a unique approach to address the anatomical variability observed in patients. Regardless of these diverse techniques, achieving the utmost accuracy is imperative to avoid potential catastrophic injuries because vascular injury manifests in 1–4% of cervical spinal procedures, with potentially disastrous consequences (1,4,5).
Therefore, reproducible anatomic precision is necessary. A noteworthy array of intraoperative three-dimensional (3D) imaging techniques has emerged from early uniplanar C-arm fluoroscopy, leading to the current generation of advanced multiplanar 3D tools, such as the mobile intraoperative computed tomography (iCT) system known as AIRO CT (Airo TruCT, Stryker, Kalamazoo, MI, USA) (6,7). Based on these 3D images, computer-assisted navigation (CAN; Brainlab, Munich, Germany) can augment the accuracy of screw placement and the incidence of substantial deviations from the preconceived surgical plan. CAN tool are designed as an intraoperative image-guided localization systems (8). Although navigation systems have become well-established in lumbar and thoracic surgeries, their utilization in the cervical spine has been relatively constrained. Therefore, limited literature exists regarding the utilization of CAN surgery in the context of cervical spinal instrumentation (9). This can be ascribed to the varied screw trajectories necessary for cervical spine procedures, coupled with potential anatomical variations such as a high-riding vertebral artery, increasing the degree of challenge during surgery. In the context of C1–2 transarticular screw fixation, Weidner et al. observed that image-guided surgery succeeded in reducing, though not eliminating, the risk of screw misplacement (10). In a study in 2003, navigation was demonstrated to be effective in 34 cases of C1–2 transarticular screw fixation, but the need for frameless image-guidance techniques to enhance the perioperative versatility of this technology was highlighted (11). A later investigation showed no significant misplacement of posterior cervical instrumentation after performing CAN using Brainlab surface matching (12). Nonetheless, surface matching has consistently yielded errors up to twice as large as point-pair matching with adhesive markers (13).
Hence, the evolution of matching techniques has progressed from manual matching to the implementation of automatic image-registration methods, which involve the simultaneous acquisition of images using iCT and their integration with preoperative imaging modalities, such as magnetic resonance imaging (MRI). To date, there is a paucity of literature describing modern techniques of upper cervical spine instrumentation employing automatic image registration and CAN, including postoperative validation using intraoperative AIRO CT imaging for various anatomical challenges and pathologies.
This study outlines a range of surgical techniques for posterior cervical fusion, tailored to anatomical variations of three different patients by employing CAN guided by preoperative MRI data and iCT scans. The described procedures involve classic cervical screws, including C1 lateral mass screws with the notching technique, C2 and C3 pars screws, and translaminar screws. Accuracy in screw placement is confirmed through iCT scans. We present this article in accordance with the SUPER reporting checklist (available at https://jss.amegroups.com/article/view/10.21037/jss-24-26/rc).
Preoperative preparations and requirements
Overall
For this technique, upper posterior cervical fusion procedures were performed utilizing CAN in conjunction with iCT imaging equipment.
To enhance practical understanding, the surgical technique is described based on three clinical cases. Patient-specific demographic characteristics and perioperative data are shown in Table 1.
Table 1
Patient parameters | Patient 1 | Patient 2 | Patient 3 |
---|---|---|---|
Demographic | |||
Sex (M/F) | F | F | M |
Age (years) | 61 | 64 | 75 |
BMI (kg/m2) | 29 | 32 | 30 |
ASA | 2 | 2 | 2 |
Smoker (yes/no) | No | No | Yes |
Previous cervical surgery | Yes | No | No |
Perioperative data | |||
Surgery time (min) | 178 | 219 | 260 |
Blood loss (mL) | 100 | 100 | 50 |
Length of stay (days) | 2 | 2 | 2 |
Follow-up (days) | 196 | 375 | 348 |
M, male; F, female; BMI, body mass index; ASA, American Society of Anesthesiologists.
Preoperative imaging
Before each surgery, routine preoperative imaging was conducted, encompassing X-ray, MRI, and CT scans. The MRI was loaded onto the CAN software, where the images were automatically matched with the iCT-acquired images at a later time point.
Surgical setup
Setup and patient positioning
After the general endotracheal anesthesia, the patients were positioned prone on a Jackson table. The setup for a cervical spine navigation procedure was initiated by applying radiolucent Mayfield tongs, ensuring that the pins were placed in accordance with established protocols. A rigid arm, equipped with jointed extensions and four reflective optical spheres, was aseptically affixed to the mid-portion of the Mayfield tongs using a customized attachment arm. This configuration allowed for unobstructed line-of-sight visualization of the optical array by the navigation camera, which was strategically positioned at the foot of the operating table to prevent interference from the surgeon’s hands or instruments (Figure 1).
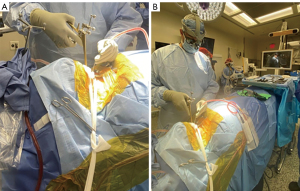
To ensure successful registration of navigated instrumentation through an iCT workflow, the surgical exposure was conducted prior to the registration process. This sequence was designed to prevent any potential disturbance to the optical array during surgical exposure. Subsequently, an iCT scan was acquired with the automated procedures in place (14).
Approach
A standard posterior cervical incision was performed, and electrocautery was employed to achieve dissection through the dermal layers, progressing down to the deep fascia in the midline avascular raphe through the ligamentum nuchae. Cobb and Penfield elevator subperiosteal elevation was utilized to expose the anatomical structures associated with the C1–2 region. This involved careful dissection along the posterior arch of C2, encompassing the lamina of C2, extending outward to the lateral aspect, and addressing the C1 arch on the undersurface of the lateral aspect, as well as in the midline.
iCT imaging
After the primary surgical approach, an iCT scan was performed. The iCT was equipped with reflective flat markers that allowed for automatic scanner calibration and image registration. Immediate image transfer to navigation and automatic registration of each scan was possible. In the context of navigation, conducting an iCT scan or verifying preoperative CT data using specific points identified within lateral and oblique fluoroscopy images is required. However, this alternative workflow involving the colocalization of preoperative CT scans with intraoperative fluoroscopic images remains relatively unexplored, particularly in the context of cervical spine procedures (14).
Registration for navigation
Concurrently with the acquisition of the iCT scan, the automatic image registration process was initiated, facilitating the seamless registration and automated transfer of the scans to the navigation system for the planned surgical procedure. Upon the successful completion of registration, the targeted screws were precisely positioned using the 3D navigation system.
CAN software
The navigation software consisted of an image fusion part and a spine planning application. It effectively colocalized a preoperative MRI dataset with an iCT scan. Despite changing from a supine position during MRI to a prone position for iCT, registration mismatches for the upper cervical spine. This is due to the fact that the atlantoaxial spine’s primary motion involves axial rotation rather than flexion-extension (15). This integrated approach not only facilitated navigation of the bone anatomy for screw instrumentation but also provided guidance for navigating associated soft-tissue structures. This capability proved particularly advantageous in minimally invasive procedures where the objective was to selectively decompress the pathological stenotic tissue while preserving the stability of the operative segment. It also allowed for precise navigation without the need for direct visualization and open exposure. The image fusion technique enabled the registration of a minimum of two image sets, whether they were of the same or different modalities (e.g., CT, MRI). The algorithm of the fusion process aligned the selected image sets by identifying common anatomical structures to achieve optimal fusion results. It was imperative that the two image sets shared a common anatomical area for successful fusion. Once the two image sets were fused, they could be simultaneously visualized, with all planned content, such as trajectories, defined in one image set being readily visible in any other fused image set.
The CAN Spine Planning application was used to plan the screw trajectories. Surgical planning could be conducted either preoperatively or intraoperatively. The software included the automatic labeling of vertebrae, precise measurements, and the generation of screw placement recommendations spanning from the cervical to the sacral region, encompassing sacroiliac and ilium screws (Figure 2).
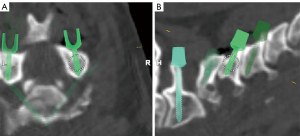
Step-by-step description
Step-by-step description of a nonunion of C2 fracture
All procedures performed in this study were in accordance with the ethical standards of the institutional and/or national research committee(s) and with the Helsinki Declaration (as revised in 2013). Written informed consent was obtained from the patient for publication of this article and accompanying images. A copy of the written consent is available for review by the editorial office of this journal.
Indication
A 61-year-old female patient initially presented with a C2 fracture. A thorough evaluation of potential treatment options ensued, including consideration of an anterior screw fixation procedure targeting the C2 vertebra. It became apparent during the assessment that the fracture bore a notable risk of a nonunion. To ascertain the healing status of the fracture, a CT scan was performed, revealing evidence of a nonunion. The presence of a nonunion, combined with the inherent instability of the fracture, underscored the need for an additional posterior fusion.
Preoperative imaging
In the preoperative CT scan of this patient, a C2 nonunion was observed, as evidenced by the conspicuous formation of a distinct radiolucent area surrounding the anteriorly positioned C2 screw (Figure 3).
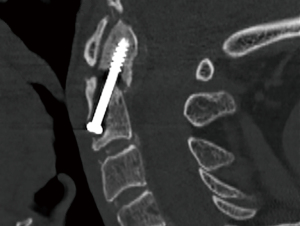
Specific challenges
The patient presented with three specific anatomic challenges. First, a previous surgery on the upper cervical spine had resulted in a remaining screw within the odontoid process. Second, the nonunion fracture could potentially alter the anatomical relationships when positioned on the operating table. Third, the patient presented with a high-riding vertebral artery. This was elucidated through radiographic assessments, both intraoperatively and preoperatively using CT scans.
Specific surgical concept customized for the patient’s anatomy
To rectify this patient’s instability, a posterior atlantoaxial fusion was proposed with lateral mass screws in C1 and preferably pedicle screws in C2. Due to the patient’s specific anatomical challenge, the high-riding vertebral arterial pedicle screw fixation was not feasible, and the decision was made to use laminar screws in C2.
C1 lateral mass screw
The lateral mass fixation technique for C1, initially described by Goel and popularized by Harms, involves navigating a surgical corridor characterized by the presence of the high cervical spinal cord medially, the vertebral artery laterally, the C2 nerve root caudally, and, often, the diminutive posterior arch of the atlas with an overlying vertebral artery cranially (16,17). Anterior to the C1 lateral mass, the internal carotid artery resides within a distance of 4.8±2.7 mm, with a minimum separation of 1.1 mm (11). This close anatomical proximity underscores the critical importance of precise screw depth in the C1 lateral mass, especially when considering the use of bicortical screws (11,18). Even a slight deviation of 1 or 2 mm in skive or screw placement could potentially lead to catastrophic clinical consequences. Given the high stakes and narrow margin for error in this region of the spine, the incorporation of intraoperative CAN may be beneficial due to its increased accuracy in comparison to conventional approaches (14,19,20).
Starting point
The starting point for these screws was at the base of the lateral mass, just below the posterior-lateral arch of the atlas (21). Notably, the starting point on the C1 lateral mass is surrounded by a rich venous plexus, and, therefore, subperiosteal dissection employing a Penfield elevator is recommended to expose the C1 lateral mass adequately.
Decortication
This step was followed by decortication of the surgical site. This can be accomplished using a small-angle curette or a high-speed burr. This step requires meticulous attention due to the proximity of critical anatomical structures, including the lateral position of the vertebral artery, the medial presence of the cervical cord, and the cranial location of the C2 nerve root. To determine the safe and feasible extent of decortication at the C1–2 articulation, a navigated probe or “pointer” was introduced into the joint, enabling 3D visualization of the articular surface.
Nerve root retraction
Once decortication had been successfully accomplished, the C2 nerve root was gently retracted in a caudal direction. The use of a computer-navigated pointer aided in visualizing the C1 lateral mass starting point; however, due to the depth of the lateral mass and the presence of the venous plexus, direct visualization could be challenging.
Navigated screw placement
The lateral mass was then tapped, probed, and determined to be entirely within bone. It is important to note that, accuracy of navigation may diminish following the use of the drill guide and its pressure. However, the correct entry point and trajectory can be determined using a reference tool without exerting pressure on the spine. Once the drill guide is positioned at the appropriate entry point and aligned with the desired trajectory, drilling commences. Navigation is then re-verified using referenced tools before screw placement. The determination of screw length and size was facilitated through intraoperative planning software, with a navigated drill guide employed to guide the drill into the lateral mass. An appropriately sized screw was inserted on each side.
Translaminar screw
In 2005, Wright introduced the C2 translaminar screw fixation technique (22). This approach involves an asymmetric placement strategy for the left and right C2 translaminar screws, characterized by distinct starting points along the cranial-caudal dimension. Given the trajectories that traverse the midline, this strategic arrangement is designed to prevent potential collisions with the contralateral screw. However, conventional open fixation methods pose two distinct challenges. First, achieving precise screw placement for both the left and right screws while avoiding interference with the contralateral screw remains critical. Second, ensuring the accurate freehand placement of the screw in terms of depth and trajectory is paramount to prevent bicortical fixation that might encroach upon the spinal canal. Neither of these technical challenges is significantly alleviated by biplanar intraoperative fluoroscopy. This limitation arises from the inherent difficulty in obtaining a clear screw axis trajectory along the lamina or obtaining a useful anteroposterior or lateral view during the procedure (14).
Starting point
First, 3D planning of the translaminar screw starting point using intraoperative software to optimize the placement of contralateral starting points was used. This strategic planning ensured that the screws could follow unobstructed crossing trajectories.
Drill
The CAN drill guide visualization, available in both sagittal and axial dimensions, enabled precise drilling of the lamina without the risk of bicortical breach, thereby enhancing surgical accuracy and safety.
Navigated screw placement
A pilot hole for translaminar fixation was created. Initially, the right lamina was drilled, probed, tapped, and then probed again, revealing that it was entirely contained within bone. Prior to screw insertion, to further ensure the precision of screw insertion, a navigated pointer, functioning similarly to a ball-tipped probe, was employed to confirm the planned trajectory (Figure 4). Subsequently, a screw was inserted, achieving fixation.
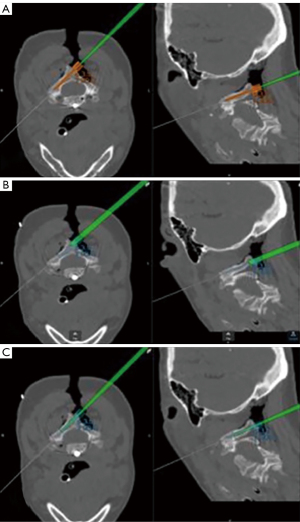
Rods
The subsequent step involved contouring rods to appropriately connect the C1 and C2 fixation points, followed by the placement of set screws.
Graft
The posterior lateral structures were decorticated to prepare a fusion bed surface. The intra-articular C1–2 joint was decorticated using a 3-mm steel bur, and a bone-morphogenetic protein allograft and crest autograft were meticulously placed within the C1–2 articulation.
Postoperative imaging
An iCT scan was conducted after implant positioning to assess the quality of the C1–2 fixation, which was found to be excellent (Figure 5).
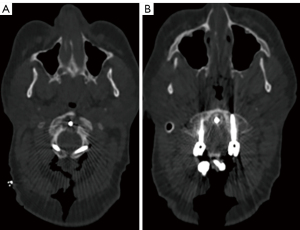
Follow-up
The patient exhibited no postoperative neurological complications and was discharged after a 2-day hospital stay. At the most recent follow-up, the patient had no more pain, and the postoperative radiograph showed evidence of successful atlantoaxial fusion, along with fusion of the previously nonunion site associated with the odontoid fracture (Figure 6).
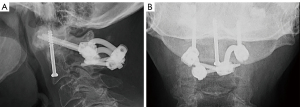
Step-by-step description of severe atlantoaxial arthropathy
Indication
A 64-year-old female patient sought medical attention due to progressively severe pain localized to the right posterior cervical and postauricular regions with radiation extending around the apex of her head, predominantly following the dermatomal distribution of the C2 spinal nerve. During clinical assessment, the patient’s cervical mobility was significantly impaired, particularly in terms of rotational motion, and the patient reporting numbness in her hands.
Preoperative imaging
Imaging findings on the patient’s CT scan confirmed the presence of C1–2 arthropathy. This pathology was characterized by a state of bone-on-bone articulation, including a severe right-sided spondylolytic degeneration of C1–2 (Figure 7).
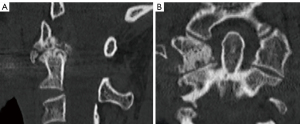
Specific challenges
The patient required an atlantoaxial fusion; however, the arch of the atlas was very narrow, which posed the risk of a spatial conflict during screw placement, potentially leading to irritation of the greater occipital nerve. To mitigate this concern, a notching technique on the undersurface of the arch was employed, effectively creating a recess that accommodated the screw head and alleviated the potential for nerve irritation. Further, the C2 pedicles appeared to be very thin, resulting in a high-riding vertebral artery.
Specific surgical concept customized for the patient’s anatomy
According to our clinical and radiological findings, our treatment strategy entailed the planning and execution of a navigated C1–2 posterior spinal fusion procedure involving a C1 lateral mass notch technique for stabilization of the atlas (C1) and the fixation of the axis (C2) vertebra through pars fixation, instead of pedicle screws with the ultimate goal of alleviating the patient’s debilitating symptoms and restoring cervical stability (23).
C1 lateral mass including notching technique
The initially prescribed starting point for atlas lateral mass screws at the base of the lateral mass has been linked to potential complications, including heightened bleeding and irritation of the greater occipital nerve. To mitigate these issues, a different practice is used of placing the screw at a more cranial position on the dorsal, posterior-lateral arch of the atlas when anatomically feasible. In instances where the arch is insufficiently spacious, a notching technique on the undersurface of the arch to create a recess for the screw can be applied (21).
Starting point
The starting point for these screws was selected at a more cranial position on the dorsal, posterior-lateral arch of the atlas. Subperiosteal dissection employing a Penfield elevator was performed to expose the C1 lateral mass adequately.
Decortication and nerve root retraction
Decortication was accomplished in the same manner as described earlier. Afterward, the C2 nerve root was gently retracted in a caudal direction. The computer-navigated pointer aided in visualizing the C1 lateral mass starting point.
Navigated screw placement
The screw was inserted partially through the posterior arch, and the lateral mass was then tapped, probed, and determined to be entirely within bone. The C1 lateral mass was drilled and probed and determined to be entirely within bone (Figure 8). It was then passed, and a 24-mm screw was inserted. A notch was made on the undersurface of C1 to accommodate the polyaxial head.
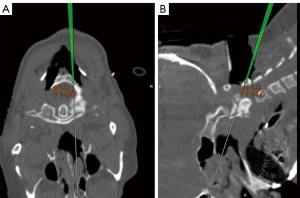
C2 pars screws
The distinctive structure of the second cervical vertebra presents significant obstacles during surgical procedures, increasing the risk of grave and possibly fatal adverse outcomes, such as damage to crucial arteries, the central nervous system, or individual nerve roots (24). However, recent technological progress in surgical instrumentation has provided essential enhancements in implant durability and versatility, as well as expanded options for screw positioning, thereby facilitating the treatment of pathologies affecting the cervical region of the spinal column (23). Compared with C2 lateral mass screws, C2 pars screws have better bone grip (16). Furthermore, they have become prevalent as an approach to decrease the risk of screw-related complications, especially injury of the vertebral artery during the perioperative period (25). Especially in the case of a high-riding or medially positioned vertebral artery, using 16-mm pars screws appears to be a better choice than pedicle screws (23).
Starting point
Pars screws were mapped out using navigation (Figure 9). The entry point for pars screw placement was strategically chosen approximately 4 mm lateral and cranial to the inferomedial border of the C2–3 facet joint. Subsequently, a pilot hole was created using a 3-mm steel burr.
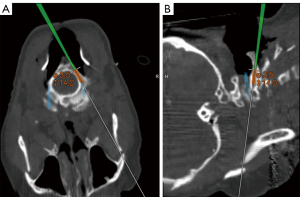
Navigated screw placement
The orientation of the screw trajectory was directed toward the junction of the pars and superior facet, guided by direct anatomical visualization. To ensure precision, a navigated drill guide and a power drill were carefully advanced along the intended trajectory, all while utilizing the image guidance system. Subsequently, a ball-tip probe was employed to inspect the area meticulously for any potential breaches.
Upon completing the tap, the screw was then inserted along the exact same trajectory, maintaining the meticulous approach to ensure optimal placement.
Rods
Rods of the appropriate length and alignment were selected and inserted in the pilot screw heads of C1 and C2.
Decortication and graft
The posterior arch and the structures of C2 underwent a delicate decortication process using a 3-mm steel burr. Subsequently, a combination of autograft and allograft materials was meticulously packed across the posterolateral fusion surfaces of C1–2.
Postoperative imaging
An iCT scan was conducted after implant positioning to assess the quality of the C1–2 fixation, which was found to be excellent (Figure 10).
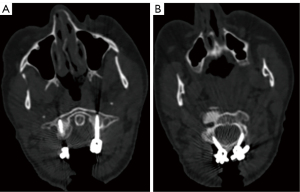
Follow-up
At 6- and 9-month postoperative follow-ups, radiographic imaging was obtained, and assessments revealed the integrity of the hardware and fixation. Additionally, the patient reported no pain or neurological deficits. A postoperative lateral X-ray obtained during the latest follow-up indicated successful posterior fusion (Figure 11).
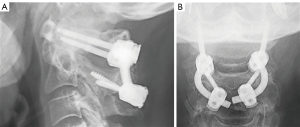
Step-by-step description of atlantoaxial arthropathy in combination with ankylosis
Indication
A 75-year-old male patient presented with severe pain and significant limitation in range of motion in the cervical spine. A comprehensive evaluation of his imaging studies was performed, and severe atlantoaxial arthropathy with ankylosis of C2–3 was shown (Figure 12).
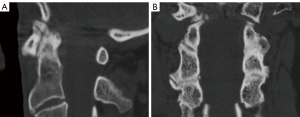
Specific challenges
Cervical ankylosis is defined by the reduced flexibility of the cervical spine resulting from partial fusion of certain segments. Notably, in cases of rheumatoid arthritis, an ankylosed spine can be accompanied by atlantoaxial instability. Although progressive facet joint destruction is commonly observed with upper cervical spine involvement, some patients may exhibit subluxation without detectable motion (26). For this patient, the preexisting fusion complicated the precise anatomical differentiation of each level, and surgery was difficult to plan due to potential underestimated instability.
Specific surgical concept customized for the patient’s anatomy
Given the potential combination of preexisting segmental fusion and instability, along with altered anatomical circumstances across multiple levels of disease, the need for highly precise intraoperative navigation becomes paramount. Precision is essential to distinguish the obscured anatomical structures and ensure the safe execution of surgery while mitigating the risk of harm to surrounding vascular and nervous structures, which could lead to catastrophic consequences.
C1 lateral mass
Starting point and nerve root retraction
The same starting point and technique for nerve root retraction was used as described earlier.
Navigated screw placement
The lateral mass was tapped, probed, and determined to be entirely within bone. The determination of screw length and size can be facilitated through intraoperative planning software, with a navigated drill guide employed to guide the drill into the lateral mass. An appropriately sized screw was inserted, first on the left and then on the right (Figure 13).
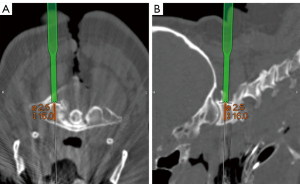
C2 and C3 pars screws
Starting point
Pars screws were mapped out using navigation (Figure 14). The entry point for pars screw placement was strategically chosen approximately 4 mm lateral and cranial to the inferomedial border of the C2–3 facet joint. Subsequently, a pilot hole was created using a 3-mm steel burr.
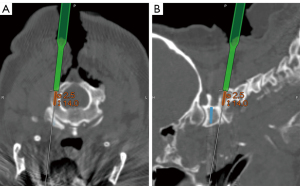
Navigated screw placement
The orientation of the screw trajectory was directed toward the junction of the pars and superior facet, guided by direct anatomical visualization. To ensure precision, a navigated drill guide and a power drill were carefully advanced along the intended trajectory, all while utilizing the image guidance system. Subsequently, a ball-tip probe was employed to inspect the area meticulously for any potential breaches.
Upon completing the tapping procedure, the screw was then inserted along the exact same trajectory, maintaining the meticulous approach to ensure optimal placement. In a parallel fashion, at the C3 level, within the lateral mass, fixation was achieved with an excellent outcome.
Rods
Subsequent to the instrumentation at the C1, C2, and C3 levels, the rods were skillfully contoured to achieve the requisite alignment and securely affixed onto the polyaxial screw heads.
Decortication and graft
The spinous process of T2 was selected as the source for local autograft, and the posterolateral structures at C1, C2, and C3 were carefully decorticated using a 3-mm steel burr. Subsequently, a combination autograft and allograft materials was meticulously packed into the fusion bed surfaces.
During the surgery, an intraoperative decision was made to incorporate bone morphogenetic protein as a supplement. This protein was strategically placed along the fusion bed surfaces spanning from C1 to C3.
Postoperative imaging
An iCT scan was conducted after implant positioning to assess the quality of the C1–3 fixation, which was found to be excellent (Figure 15).
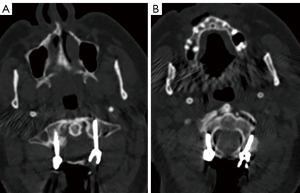
Follow-up
At 12 months postoperatively, radiographic assessments revealed the integrity of the hardware and complete posterior fusion, and the patient reported no pain or neurological deficits (Figure 16).
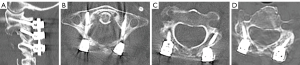
Postoperative considerations and tasks
Suture and postoperative tasks
After positioning all the required implants with navigation, when deemed necessary, a deep surgical drain was inserted. Subsequently, the fascial layer was closed in multiple layers, employing absorbable sutures. The deep dermal layer was sutured using 2-0 Vicryl material, followed by the closure of the skin layer with 3-0 Monocryl subcuticular sutures. Steri-Strips dressing was then applied to the incision site.
Postoperative imaging
Postoperative assessment involved evaluation via iCT control imaging for these three cases, illustrated in Figures 5,10,15. This assessment aimed to confirm the accuracy of screw placement. Afterward, the Mayfield tongs were removed, and the patient’s neurological and neuromonitoring status was diligently assessed.
Tips and pearls
High posterior cervical fusions, especially when utilizing CAN systems featuring automatic image registration with iCT and preoperative MRI, are technically demanding procedures and require adequate experience.
Logistics
The preoperative setup of the computer system, commencement of software initialization, and registration of the CAN may contribute to a notable extension of the duration of the surgical procedure when contrasted with the conventional freehand fluoroscopic technique.
Equipment failure or malfunction
The system may experience interruptions or crashes during an operation, attributed to hardware, software, or human factors, so it is paramount for any surgeon employing such a system to be proficient in executing the procedure independently.
Surgical technique
When exploring a new technology with different ranges of applications, a learning curve is expected. No data is available on this specific surgical technique. Therefore, as with any posterior cervical fusion procedure, a high level of experience is required. Further, a learning curve exists in robotic pedicle screw placement, but the number of cases needed to gain proficiency with this technique remains controversial (27).
Approach
A posterior approach encompasses higher risk of respiratory-, wound-, and procedure-related complications, as well as a higher incidence of symptomatic hematoma and transfusion requirements than when using an anterior approach to the cervical spine (28).
Patient-specific anatomy
Upper cervical spine instrumentation poses unique challenges due to its anatomical complexities, including the highly mobile craniovertebral junction, delicate bony structures, and the proximity of vertebral and internal carotid arteries, as well as nerve roots. The complexity is further heightened in cases, as demonstrated in this technical report, where patients exhibit altered anatomy resulting from previous surgeries, ankylosis, or a high-riding vertebral artery. Particularly in these instances, where individual variations add to the intricacy, it is crucial to rely on a surgical tool, such as CAN integrating automatic image registration with iCT and preoperative MRI, which can adapt to and incorporate these anatomical changes while ensuring high accuracy and safety.
Discussion
This surgical technique provides a systematic approach for the safe and accurate placement of axial and subaxial cervical screws, illustrated through three clinical examples, utilizing CAN systems that rely on automatic image registration with iCT and preoperative MRI. Postoperative confirmation of screw placement accuracy is achieved through intraoperative iCT scans.
Posterior upper cervical fusion is a crucial surgical procedure for treating a variety of spinal pathologies. Due to the potential risks associated with screw misplacement, such as damage to the vertebral arteries and neural structures, various techniques have been developed to assist surgeons and increase the accuracy of cervical screw placement (29). Notably, current reports still indicate a wide range of screw misplacement rates, spanning from 5% to 40%, when conventional techniques are utilized (30). The superior efficacy of 3D navigation placement when compared with freehanded techniques, enhanced by the precision of cervical screw insertion and, consequently, mitigating the complication rate, has been thoroughly scrutinized and substantiated across various research studies (30,31). The presented technique involves precise posterior upper cervical fusion techniques with CAN verified with intraoperative iCT scans.
C1 lateral mass screw fixation was developed to overcome the limitations of transarticular screw fixation (16). Bicortical fixation for C1 lateral mass screws has been shown to improve pullout strength significantly (32). Securing bicortical fixation through freehand techniques in proximity to the internal carotid artery and the C1 vertebral body is a technical challenge. Moreover, due to the inherent anatomical variations and the potential for human error, achieving the required length by precisely guiding the screw trajectory medially is a difficult task to replicate consistently with freehand methods. Prior endeavors to place C1 lateral mass screws using CAN faced limitations, as they could solely register C2 and not C1 where transarticular instrumentation for C1–2 with a CAN system could not be executed without the aid of an image intensifier, especially in the lateral perspective (12). Since then, further studies performed successful atlantoaxial fusion, as described by Goel and Harms, utilizing CAN (19). However, this study utilized Kirschner wires and cannulated screws, different from the here-described surgical technique (19).
Notching of a C1 arch for lateral mass screw insertion requires accurate navigation because the entry point for this screw deviates by only a few millimeters from a standard entry point. Therefore, a precise vision of the anatomy is required because it has been shown that the average thickness of the posterior lateral arch is 3.95 mm (21). This minimal but critical deviation posed no significant challenge when employing the 3D navigation system in our cases by securely positioning the C1 lateral mass screw using a notching technique with CAN. This observation underscores the system’s exceptional accuracy, even in highly intricate anatomical scenarios where minute differences can have substantial clinical implications. Importantly, no instances of malposition of any C1 screws were observed. Furthermore, we achieved bicortical fixation in C1 safely.
C2 translaminar screws eliminate the risk of vertebral artery injury associated with screw perforation. However, they necessitate precise placement due to the proximity of the spinal cord, which lies just ventral to the screw tract (33). To be more precise, to place translaminar screws safely, multiple considerations, such as a minimal thickness of the lamina, as well as the laminar inclination angle, must be taken into account to minimize the risk of spinal canal violation and laminar breach (34,35). The use of techniques such as CAN can be valuable in translaminar screw placement by helping to avoid dorsal cortical breaches that could compromise fixation, as well as ventral cortical breaches that pose a risk of neurological injury. A study conducted by Ma et al. unveiled a noteworthy incidence of misplaced screws, including 10 cortical breaches, within their examination of 68 translaminar screws (36). In this paper, we present a safe CAN technique for such screws, confirming their position in intraoperative iCT.
C2 pars screws are typically reserved for patients with anatomical characteristics less conducive to the use of pedicle screws. For example, when requiring a long C2 pedicle screw the risk facet-joint penetration is increased, potentially choosing a C2 pars screw in these cases would be beneficial (37). The use of C2 pars screws is less frequent in comparison with pedicle screws, and their documentation in the literature is relatively sparse. In a review of C2 pars screw placement guided by fluoroscopy, Elliott et al. noted that 3% to 8% of screws exhibited mispositioning. Conversely, an investigation employing the freehand technique, as reported in a single cadaveric study, encountered two critical deviations among 16 screws (38). This study concluded that the freehand approach exhibited less reliability when applied to pars screws in contrast to pedicle screws. Furthermore, in a study encompassing 219 pars screw placements, subsequent postoperative CT scans revealed breaches in 11% of the total screws (39). Among these, 2.3% constituted high-grade breaches, involving one medial breach and one superior breach (39). In our performed cases, immediate intraoperative confirmation with iCT confirmed no breach of any C2 pars screw. However, the number of cases presented in this technical report is limited.
Although the CAN system represents a valuable and intriguing tool in the realm of spine surgery, it is imperative that users acknowledge the potential limitations. Given that the system may experience interruptions or crashes during an operation, attributed to hardware, software, or human factors, it is paramount for any surgeon employing such a system to be proficient in executing the procedure independently.
To achieve successful CAN of surgical screws in the cervical region, the technological setup must be adapted to the constrained posterior cervical surgical field. The small anatomic structures and close proximity to an array increase the risk of array displacement, which can compromise the accuracy of the procedure. Moreover, cervical spinous processes are notably smaller compared with their lumbar counterparts, and even slight deviations can lead to severe complications.
Consequently, it is imperative to ensure the proper adaptation of both the registration and navigation tools, as well as the imaging modalities employed for preoperative and intraoperative screw planning.
The use of a reference clamp has previously been described as a potential source of error, being affixed to the vertebra upon which the surgeon intends to operate (12). Within the mid-cervical spine, the diminutive size of the spinous processes poses a challenge in establishing a stable fixation for the reference clamp. This challenge is exacerbated by the fact that many reference clamps were originally designed for use in the lumbar and thoracic spine (12). To address this issue, in our study, we used a radiolucent Mayfield clamp as an alternative fixation tool tailored to the unique anatomical characteristics of the cervical spine. We believe using this radiolucent Mayfield clamp overcomes the challenge of smaller spinous processes. It reduces the hardware needed in the limited space simultaneously, as well as reduces the chances of displacing the array by moving it out of the surgical field.
However, some practical challenges remain when positioning the reference array on the rigid Mayfield tongs. Although promising innovations are emerging that may potentially eliminate the requirement for Mayfield tong referencing, these advancements are not yet commercially available.
Furthermore, the combination of preoperative MRI and intraoperative iCT may be critical in achieving accurate screw positioning. This was shown in Hlubek et al.’s study involving patients with postoperative CT imaging; the freehand technique was significantly more accurate in comparison with using only CT-based navigation for C2 pedicle or pars screw placement (40). In our study, we used a preoperative MRI in combination with an intraoperative iCT, which led to excellent screw placement in every case without deviation of the trajectory and no postoperative neurological complications. It is important to note that the imaging prerequisites, including an isotropic MRI scan and precise colocalization with iCT scans, may not be universally applicable across various imaging and surgical centers at present.
The meticulous documentation of precision in cervical spine surgeries cannot be overstated, as it underpins the establishment of safety benchmarks for future applications. We believe that the combination of CAN and iCT scanning is poised to evolve into a quintessential component of state-of-the-art surgical spine care in the immediate future. Simultaneously, advancements in software are rapidly overcoming limitations, enabling CAN not only for assessing bony anatomy in instrumented cases but also for guiding decompression aspects of surgical procedures.
Conclusions
The surgical technique described in this paper demonstrates a detailed approach to posterior fixation strategies for variable conditions affecting the high cervical spine. It utilizes CAN systems with automatic image registration, combining iCT and preoperative MRI.
Acknowledgments
Funding: This study has been funded by a research grant by
Footnote
Reporting Checklist: The authors have completed the SUPER reporting checklist. Available at https://jss.amegroups.com/article/view/10.21037/jss-24-26/rc
Peer Review File: Available at https://jss.amegroups.com/article/view/10.21037/jss-24-26/prf
Conflicts of Interest: All authors have completed the ICMJE uniform disclosure form (available at https://jss.amegroups.com/article/view/10.21037/jss-24-26/coif). D.R.L. reports research support from Medtronic; royalties from NuVasive, Inc. and Stryker; consulting fee from Depuy Synthes and Vizeon, Inc.; private investments from HS2, LLC, Woven Orthopedic Technologies, Vestia Ventures MiRus Investiment LLC, ISPH, and LLC; and he is the member of scientific advisory board of Remedy Logic. The other authors have no conflicts of interest to declare.
Ethical Statement: The authors are accountable for all aspects of the work in ensuring that questions related to the accuracy or integrity of any part of the work are appropriately investigated and resolved. All procedures performed in this study were in accordance with the ethical standards of the institutional and/or national research committee(s) and with the Helsinki Declaration (as revised in 2013). Written informed consent was obtained from the patient for publication of this article and accompanying images. A copy of the written consent is available for review by the editorial office of this journal.
Open Access Statement: This is an Open Access article distributed in accordance with the Creative Commons Attribution-NonCommercial-NoDerivs 4.0 International License (CC BY-NC-ND 4.0), which permits the non-commercial replication and distribution of the article with the strict proviso that no changes or edits are made and the original work is properly cited (including links to both the formal publication through the relevant DOI and the license). See: https://creativecommons.org/licenses/by-nc-nd/4.0/.
References
- Badiee RK, Mayer R, Pennicooke B, et al. Complications following posterior cervical decompression and fusion: a review of incidence, risk factors, and prevention strategies. J Spine Surg 2020;6:323-33. [Crossref] [PubMed]
- Leven D, Cho SK. Pseudarthrosis of the Cervical Spine: Risk Factors, Diagnosis and Management. Asian Spine J 2016;10:776-86. [Crossref] [PubMed]
- Soliman MAR, Khan S, Ruggiero N, et al. Complications associated with subaxial placement of pedicle screws versus lateral mass screws in the cervical spine: systematic review and meta-analysis comprising 1768 patients and 8636 screws. Neurosurg Rev 2022;45:1941-50. [Crossref] [PubMed]
- Ghaith AK, Yolcu YU, Alvi MA, et al. Rate and Characteristics of Vertebral Artery Injury Following C1-C2 Posterior Cervical Fusion: A Systematic Review and Meta-Analysis. World Neurosurg 2021;148:118-26. [Crossref] [PubMed]
- Zeidman SM, Ducker TB, Raycroft J. Trends and complications in cervical spine surgery: 1989-1993. J Spinal Disord 1997;10:523-6. [Crossref] [PubMed]
- Scarone P, Vincenzo G, Distefano D, et al. Use of the Airo mobile intraoperative CT system versus the O-arm for transpedicular screw fixation in the thoracic and lumbar spine: a retrospective cohort study of 263 patients. J Neurosurg Spine 2018;29:397-406. [Crossref] [PubMed]
- Overley SC, Cho SK, Mehta AI, et al. Navigation and Robotics in Spinal Surgery: Where Are We Now? Neurosurgery 2017;80:S86-99. [Crossref] [PubMed]
- Farah K, Meyer M, Prost S, et al. Cirq® Robotic Assistance for Minimally Invasive C1-C2 Posterior Instrumentation: Report on Feasibility and Safety. Oper Neurosurg (Hagerstown) 2020;19:730-4. [Crossref] [PubMed]
- Vaishnav AS, Merrill RK, Sandhu H, et al. A Review of Techniques, Time Demand, Radiation Exposure, and Outcomes of Skin-anchored Intraoperative 3D Navigation in Minimally Invasive Lumbar Spinal Surgery. Spine (Phila Pa 1976) 2020;45:E465-76. [Crossref] [PubMed]
- Weidner A, Wähler M, Chiu ST, et al. Modification of C1-C2 transarticular screw fixation by image-guided surgery. Spine (Phila Pa 1976) 2000;25:2668-73; discussion 2674. [Crossref] [PubMed]
- Kotani Y, Abumi K, Ito M, et al. Improved accuracy of computer-assisted cervical pedicle screw insertion. J Neurosurg 2003;99:257-63. [PubMed]
- Richter M, Mattes T, Cakir B. Computer-assisted posterior instrumentation of the cervical and cervico-thoracic spine. Eur Spine J 2004;13:50-9. [Crossref] [PubMed]
- Mongen MA, Willems PWA. Current accuracy of surface matching compared to adhesive markers in patient-to-image registration. Acta Neurochir (Wien) 2019;161:865-70. [Crossref] [PubMed]
- Lebl DR, Avrumova F, Abjornson C, et al. Cervical Spine Navigation and Enabled Robotics: A New Frontier in Minimally Invasive Surgery. HSS J 2021;17:333-43. [Crossref] [PubMed]
- Lindenmann S, Tsagkaris C, Farshad M, et al. Kinematics of the Cervical Spine Under Healthy and Degenerative Conditions: A Systematic Review. Ann Biomed Eng 2022;50:1705-33. [Crossref] [PubMed]
- Goel A, Laheri V. Plate and screw fixation for atlanto-axial subluxation. Acta Neurochir (Wien) 1994;129:47-53. [Crossref] [PubMed]
- Harms J, Melcher RP. Posterior C1-C2 fusion with polyaxial screw and rod fixation. Spine (Phila Pa 1976) 2001;26:2467-71. [Crossref] [PubMed]
- Harel R, Kimchi G, Perry A, et al. C1 lateral mass screw insertion using cannulated, navigated screws: preliminary results of a novel technique. Br J Neurosurg 2023;37:1624-7. [Crossref] [PubMed]
- Bertram U, Schmidt TP, Clusmann H, et al. Intraoperative Computed Tomography-Assisted Spinal Navigation in Dorsal Cervical Instrumentation: A Prospective Study on Accuracy Regarding Different Pathologies and Screw Types. World Neurosurg 2021;149:e378-85. [Crossref] [PubMed]
- Gierse J, Mandelka E, Medrow A, et al. Comparison of iCT-based navigation and fluoroscopic-guidance for atlantoaxial screw placement in 78 patients with traumatic cervical spine injuries. Eur Spine J ;33:2304-13. [Crossref] [PubMed]
- Lee MJ, Cassinelli E, Riew KD. The feasibility of inserting atlas lateral mass screws via the posterior arch. Spine (Phila Pa 1976) 2006;31:2798-801. [Crossref] [PubMed]
- Wright NM. Translaminar rigid screw fixation of the axis. Technical note. J Neurosurg Spine 2005;3:409-14. [Crossref] [PubMed]
- Wang Z, Chang HR, Liu Z, et al. Clinical application of the C2 pars screw technique in the treatment of ossification of the posterior longitudinal ligament. BMC Musculoskelet Disord 2022;23:176. [Crossref] [PubMed]
- Hur JW, Kim JS, Ryu KS, et al. Accuracy and Safety in Screw Placement in the High Cervical Spine: Retrospective Analysis of O-arm-based Navigation-assisted C1 Lateral Mass and C2 Pedicle Screws. Clin Spine Surg 2019;32:E193-9. [Crossref] [PubMed]
- Gorek J, Acaroglu E, Berven S, et al. Constructs incorporating intralaminar C2 screws provide rigid stability for atlantoaxial fixation. Spine (Phila Pa 1976) 2005;30:1513-8. [Crossref] [PubMed]
- Iizuka H, Nishinome M, Sorimachi Y, et al. The characteristics of bony ankylosis of the facet joint of the upper cervical spine in rheumatoid arthritis patients. Eur Spine J 2009;18:1130-4. [Crossref] [PubMed]
- McKenzie DM, Westrup AM, O'Neal CM, et al. Robotics in spine surgery: A systematic review. J Clin Neurosci 2021;89:1-7. [Crossref] [PubMed]
- Shamji MF, Cook C, Pietrobon R, et al. Impact of surgical approach on complications and resource utilization of cervical spine fusion: a nationwide perspective to the surgical treatment of diffuse cervical spondylosis. Spine J 2009;9:31-8. [Crossref] [PubMed]
- Yukawa Y, Kato F, Ito K, et al. Anterior cervical pedicle screw and plate fixation using fluoroscope-assisted pedicle axis view imaging: a preliminary report of a new cervical reconstruction technique. Eur Spine J 2009;18:911-6. [Crossref] [PubMed]
- Bredow J, Oppermann J, Kraus B, et al. The accuracy of 3D fluoroscopy-navigated screw insertion in the upper and subaxial cervical spine. Eur Spine J 2015;24:2967-76. [Crossref] [PubMed]
- Luther N, Iorgulescu JB, Geannette C, et al. Comparison of navigated versus non-navigated pedicle screw placement in 260 patients and 1434 screws: screw accuracy, screw size, and the complexity of surgery. J Spinal Disord Tech 2015;28:E298-303. [Crossref] [PubMed]
- Ma XY, Yin QS, Wu ZH, et al. C1 pedicle screws versus C1 lateral mass screws: comparisons of pullout strengths and biomechanical stabilities. Spine (Phila Pa 1976) 2009;34:371-7. [Crossref] [PubMed]
- Hu Y, Yuan ZS, Spiker WR, et al. Deviation analysis of C2 translaminar screw placement assisted by a novel rapid prototyping drill template: a cadaveric study. Eur Spine J 2013;22:2770-6. [Crossref] [PubMed]
- Chan JJ, Shepard N, Cho W. Biomechanics and Clinical Application of Translaminar Screws Fixation in Spine: A Review of the Literature. Global Spine J 2019;9:210-8. [Crossref] [PubMed]
- Wang MY. Cervical crossing laminar screws: early clinical results and complications. Neurosurgery 2007;61:311-5; discussion 315-6. [PubMed]
- Ma W, Feng L, Xu R, et al. Clinical application of C2 laminar screw technique. Eur Spine J 2010;19:1312-7. [Crossref] [PubMed]
- Rosinski A, Balcescu C, Singleton I, et al. C1-C2 Facet Joint Penetration by C2 Pedicle Screws: Influence of Local Anatomy, Bone Mineral Density, and Screw Length. Int J Spine Surg 2023;17:76-85. [Crossref] [PubMed]
- Elliott RE, Tanweer O, Boah A, et al. Comparison of safety and stability of C-2 pars and pedicle screws for atlantoaxial fusion: meta-analysis and review of the literature. J Neurosurg Spine 2012;17:577-93. [Crossref] [PubMed]
- Punyarat P, Buchowski JM, Klawson BT, et al. Freehand technique for C2 pedicle and pars screw placement: is it safe? Spine J 2018;18:1197-203. [Crossref] [PubMed]
- Hlubek RJ, Bohl MA, Cole TS, et al. Safety and accuracy of freehand versus navigated C2 pars or pedicle screw placement. Spine J 2018;18:1374-81. [Crossref] [PubMed]