Surgical treatment of post-traumatic vertebral osteonecrosis including intravertebral expansive implants—clinical, functional and imaging outcomes and a center experience over 7 years
Highlight box
Key findings
• Pioneering statistical demonstration of the clinical, functional and imaging advantages of early detection of post-traumatic vertebral osteonecrosis, so that there is still enough bone tissue in the vertebral body to allow for the stabilization and restoration of its anatomy through a minimally invasive interior reconstruction, with percutaneous access and quicker convalescence, that is to say, armed kyphoplasty.
What is known and what is new?
• The optimal treatment of post-traumatic vertebral osteonecrosis continues to be an under discussion and controversial subject.
• Armed kyphoplasty with expansive intravertebral implants is an emerging procedure, which, in theory, allows for a more effective maintenance of the restored vertebral height.
• We present a detailed description of the clinical-functional and imaging outcomes of a surgical algorithm including expansive intravertebral implants for the treatment of this condition, based on osteonecrosis stages according to vertebral body morphology (non-plana or plana) and mobility (mobile or immobile).
What is the implication, and what should change now?
• Currently, the majority of post-traumatic vertebral osteonecrosis cases can be anatomically restored by a posterior minimally invasive approach, armed kyphoplasty, with satisfactory clinical, functional e imaging outcomes.
• The surgeon must be alert for this condition early detection, avoiding bone necrosis and resorption progression, so that there is still enough bone tissue in the vertebral body to enable for the stabilization and restoration of its anatomy through a minimally invasive interior reconstruction, that is to say, armed kyphoplasty.
• Long-term prospective studies are needed so as to prove the effectiveness of intravertebral expansive implants and of the presented surgical algorithm.
Introduction
We first heard of post-traumatic osteonecrosis of the vertebral body in 1891, when Hermann Kummell initially described it as a vertebra collapse symptom emerging from weeks to months, following a minor trauma, and pinpointing nutritional insufficiency of the vertebral body as the etiological hypothesis (1,2). Firstly, it was regarded as an infrequent condition. Nevertheless, its diagnosis has become more frequent, seemingly because of population aging. In fact, it is more usual in older patients with osteoporosis and in the thoracolumbar transition (3-7). Presently, post-traumatic vertebral necrosis is considered to be underdiagnosed and regarded as having a significant incidence in the population. Effectively, reports point out prevalence from 7% to 37% of vertebral compression fractures, specifically involving cases with greater flattening and a more comminuted pattern than the ones located in not so vascularized regions of the vertebral body. Those are usual risk factors for the emergence of non-union in general. A kind of extrinsic interference, comprised of an overload on a weakened fractured vertebra with no sufficient stability to heal, has been described. In fact, the evolution of the vertebral fracture to non-union, with gradual osteonecrosis of the vertebral body and subsequent loss of its structural integrity, as well as neurological risk, poses one of the most unpredictable and concerning issues regarding spine traumatology. On this wise, patients presenting symptomatic vertebral necrosis, with or with no neurological compression symptoms, may be considered for surgical intervention, which can range from vertebroplasty, kyphoplasty and posterolateral arthrodesis to corporectomy and vertebral body total replacement (1,2,8-11). Atrophic type pseudarthrosis, present in fractures in general, is often treated resorting to: bone resection, mending bony ends aiming at restoring blood and growth factors for that particular area; fixation; and local application of bone graft, prompting bone healing. Nevertheless, regarding the spine, along with fixation methods, cementoplasty techniques (vertebroplasty and kyphoplasty) have been successfully applied in the treatment of this condition, instantly stabilizing the vertebral body with no wait for bone healing (5-9). Indications for each kind of surgical intervention are related to the type and stage of vertebral necrosis, the integrity of the vertebral body, spinal stability, degree of future demand of the spine for each patient and the latter’s previous functional condition. Nonetheless, the specific indications for each procedure are still not clearly established in the literature (1,2,8-11).
Considering the scant scientific literature at disposal, the authors came up with a classification for post-traumatic vertebral necrosis, which we followed as a treatment guide in this research. That classification lies on criteria straight connected to the surgical therapeutic approach chosen, such as mobility dynamics and morphology of the necrotic vertebra (1-10,12,13). This way, we can refer to two kinds of vertebral morphology: vertebra plana and vertebra non-plana. Furthermore, there are two different kinds of mobility: vertebrae with mobile deformity or pseudarthrosis, which has intrasomatic clefts corresponding to the mobile region; as well as vertebrae with immobile deformity, characterized by not having any intravertebral cleft. Those kinds of mobility and morphology can come together in four stages, as represented in Figure 1. The establishment of vertebral morphology and mobility in post-traumatic necrosis has to be carried out through the association of: radiographs, comprising dynamic radiographs in orthostatism and hyperextension; computed tomography (CT); and magnetic resonance imaging, also allowing for the evaluation of the amount of remaining bone tissue. The kind of vertebral morphology and of the mobility of the necrotic vertebra will set the tone for the surgical therapeutic choice, considering or not the possibility to maintain the vertebral body, whether or not performing kyphoplasty (1-10,12,13). Vertebra non-plana morphology consists of a vertebral body with an equal or greater height than one third of the height of the original body along its whole length. Vertebra non-plana is considered as a vertebral body which still have enough bone tissue, particularly with preserved bone cover (cortical ring plus endplates), which allows for kyphoplasty, enabling the reconstruction of the interior of the vertebral body (1-10,12,13). The favorable results in osteoporotic and traumatic vertebral fractures of armed kyphoplasty (kyphoplasty with the application of recent expansive intravertebral implants) allowed its indications to be extended to vertebral non-union situations, which demonstrated promising results (14,15). The objective of the present work is to evaluate the clinical, functional and imaging outcomes of the surgical treatment of situations of post-traumatic vertebral necrosis, following a therapeutic algorithm that includes armed kyphoplasty with intravertebral expansive implants and bridge pedicular stabilization, according to a predefined necrosis stage. We present this article in accordance with the STROBE reporting checklist (available at https://jss.amegroups.com/article/view/10.21037/jss-24-6/rc).
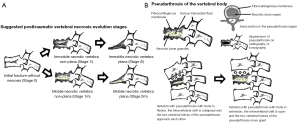
Methods
This investigation is a retrospective observational study in which we analyzed every patient diagnosed with post-traumatic vertebral necrosis who underwent surgical stabilization treatment in the last 7 years (between 2016 and 2023) in the same center. The post-traumatic vertebral necrosis diagnosis criteria were the following: patients who are still symptomatic from 6 weeks to 3 months after a vertebral fracture, namely maintaining axial pain at the level of the vertebral fracture with associated functional limitation, and who have imaging hints of vertebral necrosis on CT and magnetic resonance, with or with no gradual flattening and collapse, or the establishment of intrasomatic clefts (1,2,8-11). After the selection process (Figure 2), which only includes patients with a minimal follow-up time of 1 year after surgery, 39 operated patients were identified, of whom 2 had already passed away. Nevertheless, two patients were excluded as we were not able to contact them, leading to a final sample of 35 patients, that is to say 35 vertebrae that had undergone surgery. The treatment algorithm and the surgical techniques applied are described and illustrated in Figure 3 (1-10,12,13). In summary, armed kyphoplasty was performed on non-plana mobile and immobile vertebrae, and the decision whether or not to resort to adjacent pedicle fixation was taken according to each particular case. This additional stabilization measure was preferred in cases of location in the thoracolumbar transition, greater vertebral body bone loss and involvement of the posterior wall. In plana immobile vertebrae, bridge pedicle fixation of the adjacent levels was performed, given the presence of patients with contraindications for corporectomy and total vertebral body replacement (1-10,12,13). Clinical and functional evaluation was carried out retrospectively, taking into consideration clinical records concerning preoperative, immediate postoperative and current events. Every patient was clinically assessed applying the Visual Analogue Scale (VAS) for pain, the Oswestry Disability Index (ODI), and the Patient Global Impression of Change (PGIC) scale (16-18). The imaging evaluation comprised measuring the heights of the vertebral body in the sagittal plane (anterior, intermediate and posterior heights) and in the coronal plane (right, central and left heights). It also involved the calculation of the Beck index (anterior height/posterior height), considering the CT obtained preoperatively, in the immediate postoperative period and at the end of the follow-up period.
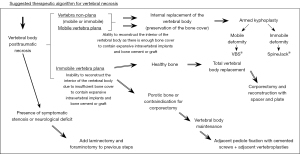
Statistical analysis
Statistical analysis was carried out resorting to the IBM SPSS Statistics for Windows, Version 28.0 (IBM Corp., Armonk, NY, USA) software. The Shapiro-Wilk normality test acknowledged asymmetric variable distributions. Moreover, we applied nonparametric statistical tests. P values <0.05 were found statistically significant.
Ethical approval
Our study took place according to the Declaration of Helsinki (as revised in 2013). The research project was approved by the Ethics Committee of Coimbra Hospital and University Centre (No. OBS_SF_169_2023). Every patient participating in this investigation signed an informed consent.
Results
Our sample included 35 patients between 56 and 87 years old, with a mean of 71.69±8.36 years at the initial fracture and corresponding to 35 non-union vertebras. The mean follow-up time was 3.17 years, with a minimum of 1 year and a maximum of 7.5 years. The most frequent mechanism (74.29%) of initial fractures was a fall from a standing height with trauma to the thoracolumbar spine, with only two cases of osteoporotic spontaneous fractures without trauma. Figure 4 demonstrates that the most affected vertebrae correspond to the thoracolumbar transition and that 82.86% of the sample presented a situation of vertebral pseudarthrosis (mobile), with the remaining necroses being immobile (Figure 1). In cases of mobile vertebrae, the anterosuperior location of the intravertebral cleft was the most common (54.3%). The percentage of vertebrae plana corresponded to 34.29% of the sample [stages 2m (mobile necrotic vertebra plana) and 2i (immobile necrotic vertebra plana)]. Regarding the type of initial fracture, the vast majority of the samples were incomplete burst fractures, that is, type A3 cephalic (82.9%) of the AOSpine classification, followed by A3 cephalic with B1 component (11.4%), that is, with fracture of the posterior elements (19). All fractures initially underwent conservative treatment with application of a thoracolumbar vest. The time from conservative treatment from the initial fracture to surgical intervention for vertebral necrosis had an average of 141.49 days and a maximum and minimum of 915 and 27 days, respectively. The most common type of surgical intervention performed was armed kyphoplasty (45.7%), followed by armed kyphoplasty associated with adjacent pedicle fixation (42.9%) and, finally, pedicle bridge fixation (11.4%), according to the algorithm previously presented (Figure 3). Several cases found in this series are illustrated in Figure 5. The existence of neurological deficits or previous radiculopathy associated to the necrotic vertebra was found in 4 patients (14.3%). In those patients, besides treating the necrotic vertebra, a laminectomy and/or foraminotomy was simultaneously carried out aiming at neurological decompression. However, there was no statistically significant difference in terms of clinical and imaging results compared to the remaining cases. The clinical and functional results in the 4 time periods analyzed are illustrated in Figure 6 (VAS), Figure 7 (ODI score) and Figure 8 (PGIC scale). A statistically significant difference was identified in VAS and ODI clinical parameters between the preoperative values (VAS 7.63±1.48, ODI 64.6±12.44) and the immediate postoperative values (VAS 4.37±1.5, ODI 40.09±10.14, P<0.001) and the end of follow-up time (VAS 3.23±1.63, ODI 31.71±12.41, P<0.001). Regarding the PGIC scale, there was a significant difference between the preoperative period (3.80±0.58) and the immediate postoperative period (5.34±0.64, P<0.001), as well as the end of follow-up period (5.94±0.80, P<0.001).
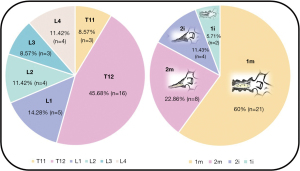
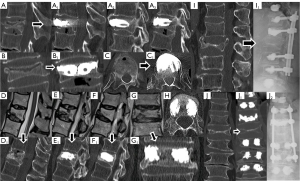
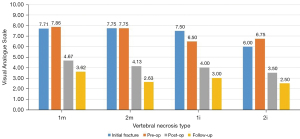
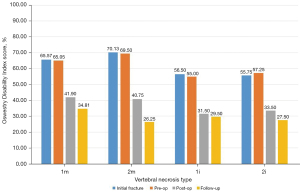
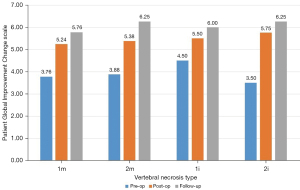
Analyzing the evolution of heights in the sagittal and coronal plane, as well as the Beck index, they all behaved in a similar way in the mobile vertebral necrosis types or pseudarthrosis [1m (mobile necrotic vertebra non-plana) and 2m] (Figures 9,10): they showed a statistically significant decrease between the initial fracture and the preoperative period (P<0.001), with a statistically significant increase between the preoperative period and the immediate postoperative period (P<0.001). In turn, in immobile vertebral necrosis [1i (immobile necrotic vertebra non-plana) and 2i] (Figure 11), there was neither a statistically significant decrease between the initial fracture and the preoperative period nor an increase between the immediate preoperative and the postoperative period. Despite not having a statistically significant difference, between pre- and postoperative periods, there was an increase in vertebral heights, even though less pronounced than in mobile necrotic vertebrae. In particular, in the immobile plana vertebrae (2i), the vertebral heights are maintained at various periods of time, as in these cases the therapeutic objective is to stabilize with a bridge fixation at adjacent levels and not to intervene in the no longer restorable morphology of the rigid vertebra plana. When comparing clinical-functional and imaging parameters between the various types of vertebral necrosis, only statistically significant differences were identified between non-plana vertebrae and the immobile plana vertebra stage (2i) in terms of anterior sagittal heights (P=0.02 between 1m and 2i; P=0.03 between 1i and 2i, both in relation to the immediate postoperative period; P=0.007 between 1i and 2i in relation to the end of follow-up period) and midsagittal (P=0.01 between 1m and 2i; P=0.045 between 1i and 2i, both in relation to the immediate postoperative period; P=0.01 between 1m and 2i; P=0.02 between 1i and 2i, both in relation to the end of the follow-up period). When statistically testing the degrees of improvement of each of the clinical-functional and imaging parameters in the various types of vertebral necrosis, only statistically significant differences were identified between all types of vertebral necrosis in comparison with the immobile vertebra plana stage (2i) between the preoperative and postoperative and final follow-up, regarding anterior and median sagittal heights. There was a statistically significant difference regarding the increase in anterior sagittal height between necrosis types 1m and 2i [P=0.005 preoperative-postoperative (preop-posop) and P=0.01 preoperative-follow-up (preop-fu)] and types 1i and 2i (P=0.024 preop-posop and P=0.02 preop-fu) between preoperative and postoperative periods (1m, 4.87±2.48; 1i, 8.15±4.74; 2i, 0.05±0.1) and between the preoperative time and the ending of the follow-up period (1m, 4.4±2.4; 1i, 8.15±4.74; 2i, 0.05±0.25). Likewise, there was a statistically significant difference regarding the increase in median sagittal height between necrosis types 1m and 2i (P=0.02 preop-posop and P=0.01 preop-fu) and types 1i and 2i (P=0.01 preop-posop and P=0.006 preop-fu) between preoperative and postoperative periods (1m, 5.09±2.38; 1i, 7.45±0.21; 2i, 0.025±0.05) and between the preoperative time and the ending of the follow-up period (1m, 4.76±2.29; 1i, 7.35±0.35; 2i, 0.05±0.17). In turn, regarding the degrees of improvement in posterior sagittal height and Beck index, there was only a significant difference between types 1m and 2i (and not between 1i and 2i) between preoperative and postoperative periods (sagittal height posterior with P=0.02 and Beck with P=0.048; 1m sag post, 2.23±1.83; 1m Beck, 0.15±0.12; 2i sag post, 0; 2i Beck, 0.002±0.005) and between the preoperative period and the ending of the follow-up period (posterior sagittal height with P=0.044 and Beck with P=0.045; 1m sag post, 1.74±2.5; 1m Beck, 0.15±0.15; 2i sag post, −0.5±1; 2i Beck, 0.005±0.009).
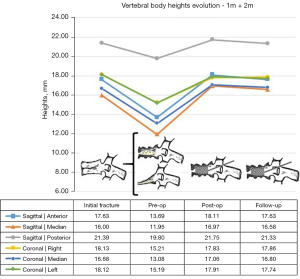
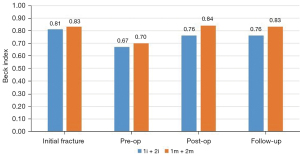
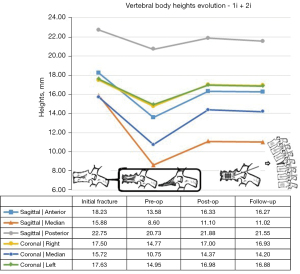
When grouping the necrosis into mobile (1m and 2m) and immobile (1i and 2i) vertebrae, the only statistically significant difference identified was the postoperative median sagittal height (P=0.005), corresponding to 16.97±0.52 mm in mobile and 11.1±2.83 mm in properties. In turn, grouping the necroses into vertebrae non-plana (1m and 1i) and vertebrae plana (2m and 2i), statistically significant differences in median sagittal height were observed postoperatively (P=0.024; 17.21±2.96 in non-plana and 13.56±5.49 in plana cases), in the anterior sagittal height at the end of the follow-up period (P=0.047; 18.33±2.49 in the non-plana cases and 15.62±4 in the plana cases) and in the median sagittal height at the end of the follow-up period (P=0.006; 16.9±2.64 in non-plana and 13.18±5.48 in plana cases). In the same way, analyzing non-plana versus plana vertebrae, but now comparing the degree of height improvement in these types of vertebral necrosis, statistically significant differences were identified for anterior sagittal height, posterior sagittal height and the Beck index between preoperative and postoperative periods (anterior sagittal height with P=0.003, being 5.15±2.74 mm in non-plana and 2.17±2.06 mm in plana; posterior sagittal height with P=0.002, being 2.33±1.8 mm in non-plana and 0.82±1.25 mm in plana cases; Beck index with P=0.034, being 0.16±0.12 in non-plana cases and 0.07±1.25 in plana cases) and between the pre-operative period and the ending of the follow-up period (anterior sagittal height with P<0.001, being 4.73±2.72 mm in non-plana 1.8±1.86 mm in plana; posterior sagittal height with P=0.007, being 1.89±2.46 mm in non-plana and 0.48±1.63 mm in plana; Beck index with P=0.007, being 0.16±0.15 in non-plana cases and 0.06±0.007 in plana cases). As for the clinical-functional parameters, there was only a statistical difference in terms of the ODI score between the pre-operative time and the ending of the follow-up period (−29.82±15.05 in non-plana cases; −38.75±13.41 in plana cases; P=0.048), corresponding the greatest improvement in the ODI for the plana vertebrae group.
Complications were observed in 9 patients (25.7%), and their occurrence did not demonstrate a statistically significant difference in terms of clinical and imaging results compared to patients without complications. These essentially consisted of bone cement leakage (77.78% of complications), the majority without clinical repercussions; however, one patient had radicular pain and other a partial deficit in ankle dorsiflexion. The other complications consisted of isolated cases, one of non-complete expansion of a stent, one of mild pullout of pedicle screws and another of fracture at an adjacent level, without clinical repercussion. There was no statistically significant difference in the occurrence of surgical complications between the various stages of vertebral necrosis, although there was a tendency for more complications to occur in stages 2 of classification (vertebrae planas), with stage 1m having 23.8% of complications, and stages 2m and 2i presenting 37.5% and 25%, respectively. However, comparing patients with complications and the group without complications, there was a significant difference between them in terms of improvement in posterior sagittal height between preoperative and postoperative periods (P=0.02) (0.63±1.4 mm in patients with complications and 2.22±1.73 mm in those without complications) and between the preoperative time and the ending of the follow-up period (P=0.046) (0.19±1.81 mm in patients with complications and 1.83±2.31 mm in those without complications). The existence of a correlation between the time elapsed between the initial fracture and surgical intervention after diagnosis of vertebral necrosis, and the clinical-functional and imaging parameters was investigated, and a significant correlation was found in the reverse direction, between this time and the anterior sagittal height in the immediate postoperative time (Rho =−0.50, P=0.002) and at the ending of the follow-up period (Rho =−0.53, P=0.001), and the Beck index in the immediate postoperative time (Rho =−0.53, P=0.001) and at the ending of the follow-up period (Rho =−0.53, P=0.001). Moreover, a significant direct correlation was identified between this elapsed time and the VAS at the end of the follow-up period (Rho =0.37, P=0.028), with no correlation being identified with the ODI. Furthermore, there was also a significant correlation in the opposite direction between this time and the PGIC scale at the ending of the follow-up time (Rho =−0.44, P=0.008). In addition, patients with surgical complications had a statistically significantly longer time (P=0.003) between the initial fracture and surgery (307.89 days), compared to patients without complications (83.88 days).
Discussion
The patients with post-traumatic vertebral necrosis included in this study were classified and treated according to the proposed vertebral necrosis stages initially described, which are based on the morphology and the mobility of the necrotic vertebra (Figures 1,3) (1-10,12,13). These two parameters will dictate the surgical therapeutic choice on the grounds of the likeliness or not to maintain the vertebral body and to reconstruct it internally, allowing or not a kyphoplasty or to contain the application of expandable intravertebral implants (armed kyphoplasty) (1-10,12,13). Expandable intravertebral implants are self-expanding devices inserted percutaneously inside the vertebral body by posterior transpedicular access. Their expansion and fill with bone cement will permit the restoration of vertebral body height, integrity, and stability. The application of expandable intravertebral implants, often named as “armed kyphoplasty”, not only allows for instant analgesia and stabilization advantages of vertebroplasty and kyphoplasty but also produces a vertebral body metallic endoskeleton that assures more effective resistance, strength and long-term preservation of the vertebral height which has been restored. That occurs as vertebral endplates, following reduction, are supported in a mechanic way by the expanded devices, decreasing or averting vertebral flattening following their expansion, at the same time diminishing the menace of post-traumatic local and segmental kyphosis. This situation also assures a very stable anterior support for the vertebral body (20-34). The progression of the indications for those devices has also displayed auspicious outcomes in vertebral fractures that become chronic and symptomatic non-union cases (14,15,24). Nonetheless, once there is lack of evidence in scientific literature about the application of expandable intravertebral implants and the surgical option that should be applied in accordance with each vertebral necrosis kinds, the authors propose—mainly considering the features of these devices and the current evidence of their role in treating acute fractures—a surgical therapeutic algorithm based on the vertebral morphology and mobility of the vertebral necrosis (Figure 3) (1-4,6,7,9,10,12,13,20-34). This algorithm cannot be validated since literature is still scarce on this topic. This way, we should regard it as a first proposal of the role played by expandable intravertebral implants in the treatment of vertebral necrosis. Thus, two kinds of vertebral morphology can be distinguished: cases of vertebra non-plana and vertebra plana; and two sorts of mobility [mobile vertebrae or pseudarthrosis and rigid immobile vertebrae (Figures 1,3)] (1-10,13). In mobile vertebrae (pseudarthrosis, which means with intravertebral clefts; Figure 1), independently of their non-plana or plana morphology, there is the possibility to restore at least a part of vertebral body height through the positioning of the spine in hyperextension, leading to the separation of the upper and lower halves of the pseudarthrosis and at the same time increasing the size of the intravertebral cleft (Figures 1,3). This way, in those cases, a vertebral body with enough bone tissue, particularly with maintained bone cover (cortical ring plus endplates), enables the use of expandable intravertebral implants, allowing for the reconstruction of the vertebral body interior instead of its total substitution. This way, whenever there is necrosis with this vertebral dynamics, we propose armed kyphoplasty (Figure 12), through which expandable intravertebral implants fill the empty cavity within the vertebral body surrounded by bone trabeculae impacted by the devices. The vertebral body is then filled with bone cement, which gives it inner stability and consistency. The introduction of cement aims at filling and stabilizing the interior of the vertebral body in an inert manner, solving bone regeneration inability without having to wait for bone healing. The complete filling of the intrasomatic cleft is crucial to stabilize the vertebral body, removing the pathological and symptomatic intravertebral mobility (1-13). Authors often opt for VBS® implants in vertebrae with mobile deformity. These stents are implants with capacity for space occupation, enabling the creation of large intrasomatic cavities with a cover composed of the metallic mesh of the devices and impacted bone trabeculae. This permits the application of a greater amount of bone cement, at the same time originating less pressure and more containment in order to decrease cement leakage (Figure 12) (20-25,34-36). The cement filling in the VBS® firstly follows a cavity pattern inside the stents. Furthermore, it presents a trabecular pattern because of peripheral interdigitation, which reaches an inner network of trabeculae—penetrating the holes of the mesh of the stent upon expansion—and with the stent metallic network itself. In vertebral necrosis, it is key that cement agglomerate is peripherally immobilized through the interdigitation in surrounding healthy bone trabeculae. This can only be reached whenever there is a suitable previous removal of the fibrocartilaginous membrane, of residues of pseudarthrosis and of the peripheral sclerosis, diminishing the menace of cement and implant migration (Figure 12) (1-10,21-26,37,38). Nevertheless, if we take into consideration the hydraulic pressure-dependent expansion mechanism of the VBS® and relevant sclerotic regions in the vertebral body of the immobile necrosis type, there is the risk that the resistance of the sclerotic bone is greater than the expandable capacity of those implants. Therefore, they might not expand, or not expand enough, thus not building the intrasomatic cavities of the suitable size. In addition, in necrotic vertebrae with immobile deformity (with no intravertebral cleft), vertebral expansion cannot occur by positioning the spine in hyperextension; this way, the generation of intrasomatic spaces completely depends on the action of intravertebral implants. Therefore, concerning immobile vertebrae non-plana, we propose SpineJack® implants (Figure 3). Although they are not space-occupying, they present a more powerful and mechanical expansion capacity, which is not straightly dependent on pressure. Those implants generate intrasomatic spaces even in vertebrae with immobile deformity, which are then filled with bone cement (14,20,27-34). The filling pattern of cement associated with SpineJack® implants is primarily trabecular, once the latter can only generate small cavities, which match their vertical expansion. Therefore, after filling those small spaces, the cement interlinks the neighboring trabecular area, usually connecting the implants horizontally. As stated before, SpineJack® implants, unlike VBS®, do not generate big intrasomatic cavities where the cement will be kept inside. This means that their application in vertebral necrosis should be based on a special strict intraoperative fluoroscopic control when inserting bone cement, so that its leakage is avoided (1,27-34). Technically speaking, we emphasize the likely difficulty in drilling and opening the interior of the vertebral body with immobile deformity, since it usually alternates fragile regions of necrotic bone with areas of very resistant sclerotic bone. Therefore, we should be careful regarding this process so as to prevent crossing cortical walls and inducing considerable vascular and neurological damage. Moreover, in vertebrae with immobile deformity, the main objective is to stabilize the vertebral body, so as to avoid its gradual flattening by necrosis and bone resorption, and not to gain weight. When the morphology of immobile necrosis is non-plana, armed kyphoplasty with SpineJack® implants is, as previously stated, recommended. On the other hand, if the morphology of immobile necrosis is plana, corpectomy and replacement, or a bridge pedicular fixation should be considered (Figure 3) (1,10,12,13). Placing expandable intravertebral implants in immobile vertebra plana is very risky and may lead to significant consequences since we do not have sufficient somatic bone cover to enable a stable implants containment within vertebral bone tissue. One of those risks is migration of the implants as they are not stable within bone tissue, with the danger of considerable neurological and vascular tissue injury, cement leakage or incapacity to introduce it inside the vertebral body. Therefore, in situations of patients presenting vertebra plana with immobile deformity, in the event of conditions and functional expectations that legitimize it, the vertebral body should be totally removed (interior and exterior) through corpectomy. Bearing in mind its replacement, a spacer (synthetic cage or structural bone allograft) with anterolateral plate fixation to adjacent vertebral bodies should be used, as well as pedicular instrumentation. Nonetheless, those patients are often elderly, presenting various comorbidities, as in our sample. The patient’s physiological condition might be a contraindication for the invasiveness of the anterior approaches to thoracic or abdominal cavities, or of an extensive posterior approach, which is necessary in the case of corpectomy. In addition, osteoporotic vertebrae increase not only the danger of adjacent vertebral fractures, but also loss of fixation in the intersomatic spacer following corpectomy. Thus, regarding those usual situations, we advise adjacent percutaneous pedicle fixation with cemented screws, two levels above and below the level of the vertebra plana (Figure 3), associated to prophylactic vertebroplasties at the two adjacent upper and lower levels to the instrumentation, aiming at minimizing its overload and at reducing junctional kyphosis as well as adjacent fractures (Figure 3) (1,10,12,13,39). Through a less invasive procedure than corpectomy, this treatment aims at providing elderly patients with a fast pain relief, at the same time enabling early rise and walking. In a few situations of severe kyphosis in osteoporotic patients with sagittal imbalance, Ponte osteotomies can be carried out at some levels, in order to reduce that deformity (37,40). In this work, a detailed analysis of the clinical-functional results of surgical treatment on a sample of 35 patients with various types of vertebral necrosis was carried out over four time periods: initial fracture; pre-operative or moment of vertebral necrosis diagnosis; immediate postoperative time; and at the ending of the follow-up period. It is worth noting the length of the time interval between the initial fracture submitted to conservative treatment and the diagnosis of vertebral necrosis (27 to 915 days) corresponds to the preoperative period and represents the time elapsed until the patients were referred to our department, thus determining a high variability in terms of the stages of evolution of the disease. In this sample, there was a higher prevalence of involvement of T12 (45.7%) and L1 (14.3%) vertebrae, which correspond to the thoracolumbar hinge. This is in line with the literature when referred to as a location where there is the risk of undesirable evolution of vertebral necrosis. Continuous excessive loads on the mobile thoracolumbar transition, due to the weakened fractured T12 or L1 vertebral bodies, with marked destruction of the anterior column, may give rise to insufficient stability to provide bone healing, which leads to necrosis and gradual bone reabsorption, with subsequent loss of its structural integrity and support function, following vertebral flattening and collapse with retropulsion of the posterior wall, as well as neurological risk (1,2,8-11). Regarding the type of initial fracture, the vast majority corresponded to incomplete cephalic burst fractures [AOSpine classification type A3 cephalic (19)], accounting for 82.9% of cases. This fact and the predominant presence of the intravertebral cleft in an anterosuperior location of the vertebral body (54.3%) is in agreement with the idea that it is a region of scarce intrasomatic bone vascularization, whose fracture injury can compromise the blood supply necessary for bone healing and initiate the cascade of nonunion and pseudarthrosis with typical location (1,2,8,11). Most of the clinical-functional and imaging parameters showed important improvements after surgical treatment, in the postoperative time and at the ending of the follow-up period, which is in agreement with the results of the few previous studies carried out with expansive implants in post-traumatic vertebral necrosis and demonstrates the effectiveness of the applied therapeutic algorithm (12,14,15,26). When comparing mobile and immobile vertebral necrosis, there are significant differences in the median sagittal height in the postoperative period. Furthermore, when comparing non-plana with plana ones, there are significant differences in the median sagittal height in the postoperative time and at the ending of follow-up, and in the anterior sagittal height at the end of follow-up. Also, various degrees of improvement of sagittal heights imply a significant difference between necrosis 1m and 1i with 2i. These data are in line with the greater vertebral anatomical restoration achieved in mobile necroses and demonstrate a stronger contribution of immobile plana 2i vertebrae, in which the heights of vertebrae do not improve, to the significant differences found when comparing plana to non-plana vertebrae, since the maintenance of their heights exceeds the increase in this parameter seen at 2m. When interpreting the graphs of sagittal vertebral heights and analyzing the mobile (Graph 8) and immobile vertebrae (Graph 9), it appears that the median sagittal height is the one that reaches the lowest values, and the posterior sagittal height is the most preserved height. Overall, while in mobile vertebrae the heights are all restored, in immobile vertebrae, in particular, the anterior sagittal, the median sagittal and median coronal heights improve only slightly and remain at very low values. These findings are likely due to the pronounced contribution of the immobile plana vertebrae (2i) in which there is no increase in height. Theoretically, the increase in anterior height of the immobile non-plana vertebrae (1i) would not be as expected as in the mobile vertebrae, once the former does not have intravertebral mobility. However, an average increase in preoperative anterior height was found, from 12.65 to 20.80 mm, which was maintained throughout the follow-up period. This data, which includes only two patients with 1i vertebrae, probably demonstrates the mechanical expansion strength of the SpineJack® expandable implants, which can increase the height of the vertebral body even in the absence of a pseudarthrosis cleft and any previous intravertebral mobility, which is favorable data for its use in this specific stage of immobile deformity of the disease (1i) (Figure 3). Moreover, when grouping necrosis into stages of non-plana vertebra (1m and 1i) and plana vertebra (2m and 2i), a statistically significant greater degree of functional improvement (ODI) was found in plana vertebrae. This probably demonstrates that necrotic plana vertebrae, particularly mobile ones (probably due to accentuated intravertebral mobility) are quite symptomatic and disabling, presenting at the beginning a higher ODI score (ODI-maximum preoperative occurs in 2m necrosis and corresponds to 69.50; Figure 7). Then, its surgical treatment, whether armed kyphoplasty in the 2m or bridge fixation in the 2i stages, allows for an important functional recovery (ODI improvement between preoperative and final follow-up: 2m =43.25 and 2i =29.75 versus 1m =30.24 and 1i =25.5; Figure 7), higher than that occurring in non-plana vertebrae. The occurrence of complications was not associated with differences in terms of clinical and imaging results, which could be explained by the majority of complications being minor polymethylmethacrylate (PMMA) extravasations with no or minimal clinical repercussions. We can perhaps justify the high rate of PMMA leakage for two main reasons, the first because we analyze the leakage through CT, which detects even the smallest cement amount outside cortical walls, and that vertebral necrosis is a pathology that often makes the vertebral body morphology unpredictable, many times with disrupted cortical walls and places that alternate hard sclerotic to soft necrotic bone. Nevertheless, a statistically significant greater improvement in posterior sagittal height was observed in patients without complications. This may mean that a lesser restoration of the posterior sagittal height implies a more advanced necrotic stage, which makes impossible a more effective anatomical restoration, indicating more impairment of the vertebral morphology and the integrity of the cortical ring, which may favor cement leakage. Significant indirect correlations were found between the time from the initial fracture to surgical intervention after diagnosis of vertebral necrosis and the anterior sagittal height in the immediate postoperative time and at the ending of the follow-up period. In addition, a significant direct correlation was identified between this time as well as the VAS at the end of the follow-up time and the PGIC scale at the same period. It was also found that patients with surgical complications had a statistically significantly longer time between the initial fracture and surgery for post-traumatic necrosis. These data provide the important statistical demonstration that the later the diagnosis and surgical intervention, the more advanced the vertebral necrosis will be and the more difficult it will be to restore the anatomy of the vertebral body (in particular, its anterior height), and the more complications are expected in relation to the surgical treatment. Furthermore, a longer period of waiting time was associated with higher pain rates at the end of the follow-up period and less improvement felt, according to the PGIC scale, which implies that there is a clinical repercussion of the intervention at a stage of more advanced vertebral necrosis, although not demonstrated in ODI functional parameters. These factors support the need for early diagnosis and rapid surgical treatment in order to obtain the best clinical and functional results at treating vertebral necrosis, preferably in the early stages, with minimally invasive and anatomical restoration techniques (kyphoplasty), preventing disease progression and greater risk of surgical complications.
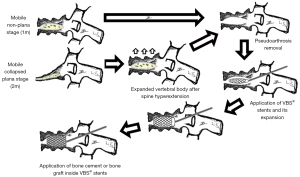
The primary limitations of this investigation are the following: its retrospective character and the reduced control for confounding factors with impact on clinical outcomes; the lack of a control group; a small number of patients at the sample, including few patients at each necrosis stage, which can compromise external validity and generalizability of the treatment algorithm outcomes; and some heterogeneity in the period until surgical intervention and in the follow-up.
Conclusions
We presented in detail the results of a surgical therapeutic algorithm that includes the use of recent expansive intravertebral implants at the entire spectrum and stages of post-traumatic vertebral necrosis, resulting in satisfactory clinical, functional and imaging results, including often an approximate anatomical restoration of the vertebral body obtained through minimally invasive access. The most important message seems to be the pioneering statistical demonstration of the clinical, functional and imaging importance of early detection (preferably in vertebrae non-plana stages 1i and 1m) of this underdiagnosed and progressive disease, so that there is still enough bone tissue in the vertebral body to permit the stabilization and restoration of its anatomy through a minimally invasive interior reconstruction, with percutaneous access and quicker convalescence, that is to say, armed kyphoplasty. At the same time, it allows for the progressive negative evolution of post-traumatic necrosis to be interrupted, avoiding vertebral collapse and the associated neurological risks. The surgeon must be alert to persistent axial pain in the context of a vertebral fracture, which, accompanied by imaging signs of vertebral non-union, allows for an early diagnosis and the subsequent treatment of the progressive disease. A late diagnosis or an unnecessary delay of surgery leads to bone necrosis and resorption to advance, giving rise to cases of vertebra plana (stage 2) and raising the risk of neurological injury, which demands more aggressive surgical solutions, with a higher risk of complications and inferior clinical-functional and imaging results. Nevertheless, we need a wider range of long-term prospective studies so as to prove the benefits and the effectiveness of these recent surgical options, such as comparative studies between armed kyphoplasty and traditional vertebroplasty or kyphoplasty, and of this therapeutic algorithm regarding an underdiagnosed, progressive, menacing and challenging disease.
Acknowledgments
Funding: None.
Footnote
Reporting Checklist: The authors have completed the STROBE reporting checklist. Available at: https://jss.amegroups.com/article/view/10.21037/jss-24-6/rc
Data Sharing Statement: Available at: https://jss.amegroups.com/article/view/10.21037/jss-24-6/dss
Peer Review File: Available at https://jss.amegroups.com/article/view/10.21037/jss-24-6/prf
Conflicts of Interest: All authors have completed the ICMJE uniform disclosure form (available at https://jss.amegroups.com/article/view/10.21037/jss-24-6/coif). The authors have no conflicts of interest to declare.
Ethical Statement: The authors are accountable for all aspects of the work in ensuring that questions related to the accuracy or integrity of any part of the work are appropriately investigated and resolved. The study took place in accordance with the Declaration of Helsinki (as revised in 2013). The research project was approved by the Ethics Committee of Coimbra Hospital and University Centre (No. OBS_SF_169_2023). Every patient participating in the study signed an informed consent.
Open Access Statement: This is an Open Access article distributed in accordance with the Creative Commons Attribution-NonCommercial-NoDerivs 4.0 International License (CC BY-NC-ND 4.0), which permits the non-commercial replication and distribution of the article with the strict proviso that no changes or edits are made and the original work is properly cited (including links to both the formal publication through the relevant DOI and the license). See: https://creativecommons.org/licenses/by-nc-nd/4.0/.
References
- Young WF, Brown D, Kendler A, et al. Delayed post-traumatic osteonecrosis of a vertebral body (Kummell's disease). Acta Orthop Belg 2002;68:13-9. [PubMed]
- Benedek TG, Nicholas JJ. Delayed traumatic vertebral body compression fracture; part II: pathologic features. Semin Arthritis Rheum 1981;10:271-7. [Crossref] [PubMed]
- Osterhouse MD, Kettner NW. Delayed posttraumatic vertebral collapse with intravertebral vacuum cleft. J Manipulative Physiol Ther 2002;25:270-5. [Crossref] [PubMed]
- Maldague BE, Noel HM, Malghem JJ. The intravertebral vacuum cleft: a sign of ischemic vertebral collapse. Radiology 1978;129:23-9. [Crossref] [PubMed]
- Lane JI, Maus TP, Wald JT, et al. Intravertebral clefts opacified during vertebroplasty: pathogenesis, technical implications, and prognostic significance. AJNR Am J Neuroradiol 2002;23:1642-6. [PubMed]
- Hasegawa K, Homma T, Uchiyama S, et al. Vertebral pseudarthrosis in the osteoporotic spine. Spine (Phila Pa 1976) 1998;23:2201-6. [Crossref] [PubMed]
- Nicholas JJ, Benedek TG, Reece GJ. Delayed traumatic vertebral body compression fracture; part I: clinical features. Semin Arthritis Rheum 1981;10:264-70. [Crossref] [PubMed]
- McKiernan F, Faciszewski T. Intravertebral clefts in osteoporotic vertebral compression fractures. Arthritis Rheum 2003;48:1414-9. [Crossref] [PubMed]
- Mirovsky Y, Anekstein Y, Shalmon E, et al. Vacuum clefts of the vertebral bodies. AJNR Am J Neuroradiol 2005;26:1634-40. [PubMed]
- Kim YC, Kim YH, Ha KY. Pathomechanism of intravertebral clefts in osteoporotic compression fractures of the spine. Spine J 2014;14:659-66. [Crossref] [PubMed]
- McCormack T, Karaikovic E, Gaines RW. The load sharing classification of spine fractures. Spine (Phila Pa 1976) 1994;19:1741-4. [Crossref] [PubMed]
- Moura DL, Gabriel JP. Expandable intravertebral implants in post-traumatic vertebral necrosis - new classification suggestion. Acta Ortop Bras 2023;31:e262943. [Crossref] [PubMed]
- McKiernan F, Jensen R, Faciszewski T. The dynamic mobility of vertebral compression fractures. J Bone Miner Res 2003;18:24-9. [Crossref] [PubMed]
- Premat K, Vande Perre S, Cormier É, et al. Vertebral augmentation with the SpineJack® in chronic vertebral compression fractures with major kyphosis. Eur Radiol 2018;28:4985-91. [Crossref] [PubMed]
- Cianfoni A, Distefano D, Isalberti M, et al. Stent-screw-assisted internal fixation: the SAIF technique to augment severe osteoporotic and neoplastic vertebral body fractures. J Neurointerv Surg 2019;11:603-9. [Crossref] [PubMed]
- Freyd M. The Graphic Rating Scale. Journal of Educational Psychology 1923;14:83-102.
- Fairbank JC, Pynsent PB. The Oswestry Disability Index. Spine (Phila Pa 1976) 2000;25:2940-52; discussion 2952. [Crossref] [PubMed]
- Dominges L, Cruz E. Adaptação Cultural e Contributo para a Validação da Escala Patient Global Impression of Change. Ifisionline 2011;2:31-7.
- Vaccaro AR, Oner C, Kepler CK, et al. AOSpine thoracolumbar spine injury classification system: fracture description, neurological status, and key modifiers. Spine (Phila Pa 1976) 2013;38:2028-37. [Crossref] [PubMed]
- Vanni D, Galzio R, Kazakova A, et al. Third-generation percutaneous vertebral augmentation systems. J Spine Surg 2016;2:13-20. [Crossref] [PubMed]
- Muto M, Greco B, Setola F, et al. Vertebral Body Stenting System for the Treatment of Osteoporotic Vertebral Compression Fracture: Follow-up at 12 Months in 20 Cases. Neuroradiol J 2011;24:610-9. [Crossref] [PubMed]
- Diel P, Röder C, Perler G, et al. Radiographic and safety details of vertebral body stenting: results from a multicenter chart review. BMC Musculoskelet Disord 2013;14:233. [Crossref] [PubMed]
- Hartmann F, Griese M, Dietz SO, et al. Two-year results of vertebral body stenting for the treatment of traumatic incomplete burst fractures. Minim Invasive Ther Allied Technol 2015;24:161-6. [Crossref] [PubMed]
- Schützenberger S, Schwarz SM, Greiner L, et al. Is vertebral body stenting in combination with CaP cement superior to kyphoplasty? Eur Spine J 2018;27:2602-8. [Crossref] [PubMed]
- Garnon J, Doré B, Auloge P, et al. Efficacy of the Vertebral Body Stenting System for the Restoration of Vertebral Height in Acute Traumatic Compression Fractures in a Non-osteoporotic Population. Cardiovasc Intervent Radiol 2019;42:1579-87. [Crossref] [PubMed]
- Distefano D, Scarone P, Isalberti M, et al. The 'armed concrete' approach: stent-screw-assisted internal fixation (SAIF) reconstructs and internally fixates the most severe osteoporotic vertebral fractures. J Neurointerv Surg 2021;13:63-8. [Crossref] [PubMed]
- Vanni D, Pantalone A, Bigossi F, et al. New perspective for third generation percutaneous vertebral augmentation procedures: Preliminary results at 12 months. J Craniovertebr Junction Spine 2012;3:47-51. [Crossref] [PubMed]
- Noriega DC, Rodrίguez-Monsalve F, Ramajo R, et al. Long-term safety and clinical performance of kyphoplasty and SpineJack® procedures in the treatment of osteoporotic vertebral compression fractures: a pilot, monocentric, investigator-initiated study. Osteoporos Int 2019;30:637-45. Erratum in: Osteoporos Int 2019;30:647. [Crossref] [PubMed]
- Noriega D, Maestretti G, Renaud C, et al. Clinical Performance and Safety of 108 SpineJack Implantations: 1-Year Results of a Prospective Multicentre Single-Arm Registry Study. Biomed Res Int 2015;2015:173872. [Crossref] [PubMed]
- Noriega D, Krüger A, Ardura F, et al. Clinical outcome after the use of a new craniocaudal expandable implant for vertebral compression fracture treatment: one year results from a prospective multicentric study. Biomed Res Int 2015;2015:927813. [Crossref] [PubMed]
- Baeesa SS, Krueger A, Aragón FA, et al. The efficacy of a percutaneous expandable titanium device in anatomical reduction of vertebral compression fractures of the thoracolumbar spine. Saudi Med J 2015;36:52-60. [Crossref] [PubMed]
- Muñoz Montoya JE, Torres C, et al. A Colombian experience involving SpineJack®, a consecutive series of patients experiencing spinal fractures, percutaneous approach and anatomical restoration 2016-2017. J Spine Surg 2018;4:624-29. [Crossref] [PubMed]
- Noriega D, Marcia S, Theumann N, et al. A prospective, international, randomized, noninferiority study comparing an implantable titanium vertebral augmentation device versus balloon kyphoplasty in the reduction of vertebral compression fractures (SAKOS study). Spine J 2019;19:1782-95. [Crossref] [PubMed]
- Kerschbaumer G, Gaulin B, Ruatti S, et al. Clinical and radiological outcomes in thoracolumbar fractures using the SpineJack device. A prospective study of seventy-four patients with a two point three year mean of follow-up. Int Orthop 2019;43:2773-9. [Crossref] [PubMed]
- Moura DL, Gabriel JP. Expandable Intravertebral Implants: A Narrative Review on the Concept, Biomechanics, and Outcomes in Traumatology. Cureus 2021;13:e17795. [Crossref] [PubMed]
- Moura DFL, Gabriel JP. Intravertebral expandable implants in thoracolumbar vertebral compression fractures. Acta Ortop Bras 2022;30:e245117. [Crossref] [PubMed]
- Kim KT, Suk KS, Kim JM, et al. Delayed vertebral collapse with neurological deficits secondary to osteoporosis. Int Orthop 2003;27:65-9. [Crossref] [PubMed]
- Hasegawa K, Homma T, Uchiyama S, et al. Osteosynthesis without instrumentation for vertebral pseudarthrosis in the osteoporotic spine. J Bone Joint Surg Br 1997;79:452-6. [Crossref] [PubMed]
- Jang JS, Kim DY, Lee SH. Efficacy of percutaneous vertebroplasty in the treatment of intravertebral pseudarthrosis associated with noninfected avascular necrosis of the vertebral body. Spine (Phila Pa 1976) 2003;28:1588-92. [Crossref] [PubMed]
- Cheung JPY. The importance of sagittal balance in adult scoliosis surgery. Ann Transl Med 2020;8:35. [Crossref] [PubMed]