Efficacy of an allograft cellular bone matrix as an alternative to autograft in anterior cervical discectomy and fusion: radiological results & safety
Highlight box
Key findings
• Of the 53.4% of patients completing 12 months follow-up, the overall fusion rate was 97.4% (1-level: 100%, 2-level: 100%, 3-level: 92.3%, and 4-level: 100%). For the 12-month effective (completers and non-completers) fusion rate if we consider all lost to follow-up based on their 6-month status, the lower-bound fusion rate for the entire cohort was 71%.
• The incidence of surgery-related and device-related complications was low, with only 2 patients (2.7%) requiring secondary surgeries.
What is known and what is new?
• Autograft is the most used bone graft for spinal fusion surgeries due to its osteogenic, osteoconductive, and osteoinductive qualities. However, the use of autografts entails drawbacks such as prolonged procedure time, uncertain bone quality, and morbidity at the graft site.
• This study demonstrates that allograft cellular bone matrices (ACBMs) may serve as a safe, effective alternative for autograft as a fusion-promoting agent in anterior cervical discectomy and fusion (ACDF) procedures up to four levels.
What is the implication, and what should change now?
• ACBM should be considered as an alternative grafting option in single and multilevel ACDF procedures, especially with patients suspected to have poor bone quality.
• Future investigations are needed to prospectively compare fusion efficacy, clinical outcomes, and safety of ACBMs to autograft in spinal fusion surgeries.
Introduction
Spinal fusion is commonly used to treat a variety of degenerative, traumatic, and malignant spine pathologies. Over the last decade, the number of individuals diagnosed with a degenerative cervical spine condition in the United States increased by 42% from 3.2 to 6.2 million annually (1,2). Approximately 25% of individuals who are afflicted by degenerative cervical spine conditions elect to undergo surgical intervention, most commonly anterior cervical discectomy and fusion (ACDF), within the first 3 months of diagnosis (3,4). As a result, the utilization of ACDF procedures has escalated over recent decades, a trend that is forecasted to persist through 2040 (5-9).
During ACDF procedures, a variety of bone grafting materials can be employed to achieve successful fusion (10,11). Historically, autologous iliac crest bone graft (ICBG) or local autograft have been considered the gold standard for grafting material due to three distinct properties: (I) osteoinductive molecules that promote bone growth; (II) osteogenic cells to facilitate fusion; and (III) an osteoconductive matrix to serve as a medium for bone growth (12-14). Nevertheless, the use of autografts entails drawbacks such as prolonged procedure time, uncertain bone quality, and morbidity at the graft site (13-16). Heneghan and McCabe (14) reported that 38% of patients who underwent ICBG harvest experienced significant pain at the donor site and lower quality of life at 3 months post-ACDF, compared to the allograft group. Additionally, Sasso et al. (13) documented persistent pain at the donor site in 31% of patients 24 months following spinal fusion with ICBG. Recently, there have been advances in ICBG harvest by utilizing the trephine technique, which has demonstrated minimal post-operative pain and complications (15,16). Although promising, there is still concern that procedures which avoid donor site morbidity (local autograft & trephine technique) may result in insufficient volume of graft, especially for older patients with lower quality bone in need of multilevel procedures (17). Overall, there remains a need to offer additional grafting options for spinal fusion surgeries, particularly for patients with low-quality bone in need of multilevel procedures.
Numerous products have been investigated as alternatives to autograft for spinal fusion. Allograft materials like demineralized bone matrix (DBM) have been associated with increased infection rates and lower fusion rates (18-20). Recombinant human bone morphogenetic protein 2 (rhBMP-2) use has resulted in high fusion rates, however, the product is not commonly used in the cervical region due to significant risk for neck edema, dysphagia, radiculitis, vertebral osteolysis, and heterotopic ossification (21-25). To date, no graft or osteobiologic has consistently exemplified the efficacy and safety to replace ICBG or local autograft as the mainstay graft option for ACDF procedures (26-28).
To establish the ideal bone graft material which includes osteogenic, osteoinductive, and osteoconductive properties, while minimizing autograft associated complications, allograft cellular bone matrices (ACBMs) have been developed. These products are composed of high quality allogenic bone that contains live cellular components like mesenchymal stem cells (MSCs) and osteoprogenitor cells (OPCs) (29,30). Despite recent investigations into the use of ACBMs as fusion adjuncts in spinal fusion, this field of research is still in its nascency, particularly in ACDF procedures (12,31-34). Two systematic reviews concluded that ACBMs are viable graft options due to their high fusion rates and low rates of complications, yet additional non-industry funded, randomized trials are needed (20,35). Two prospective studies demonstrated high safety and efficacy of the Trinity Evolution® ACBM (Musculoskeletal Transplant Foundation, Edison, NJ, USA) in single and two-level ACDFs (31,32). This is the first reported study on the third-generation Trinity Elite® (TE) ACBM (Orthofix, MTF Biologics, Edison, NJ, USA) in ACDFs. TE manufacturing is believed to yield a twofold increase in verified adult stem cells, a hydrophilic scaffold for optimal bone growth, and better handling compared to previous versions (36). The aim of this study was to evaluate the radiological outcomes and safety profile of the TE ACBM in ACDF procedures, specifically exploring its efficacy in three and four-level ACDFs. We present this article in accordance with the STROBE reporting checklist (available at https://jss.amegroups.com/article/view/10.21037/jss-23-142/rc).
Methods
Study design & population
This retrospective, single-center, consecutive case series aimed to evaluate the safety and effectiveness of the TE ACBM used in ACDF procedures between 2019 and 2021. The study was conducted in accordance with the Declaration of Helsinki (as revised in 2013). The study was approved by the Hospital for Special Surgery IRB (No. 2023-1567). Participants did not have to give informed consent due to the retrospective nature of the study it was determined there was no risk to the patient.
Seventy-three patients with clinical and/or radiological evidence of symptomatic degenerative cervical spine disease who underwent ACDF with anterior plate fixation and TE at 1 to 4 contiguous levels were included. Patients were excluded if they had a prior attempt of fusion at the same level of the current procedure, autoimmune disease, active malignancy, or less than 6-month radiologic follow-up. Patient charts were reviewed to collect demographic information, medical comorbidities, and smoking status. Pertinent intra- and post-operative data, such as estimated blood loss (EBL), operative time, and post-operative length of stay, were also recorded. Post-operatively, all complications, epidural spinal injections, and secondary surgical procedures were collected to 15 months (12-month window limit). Complications were categorized as device or surgery related.
Allogenic cellular bone matrix
The TE ACBM is a flexible putty-like allograft that contains cancellous bone, viable MSCs and OPCs, and bone-promoting growth factors. The ACBM has a confirmed cell viability of 70% or greater with a minimum of 750,000 cells/cc of which 250,000 are MSCs or OPCs. The product is cryopreserved and stored in liquid nitrogen at −185 degrees Celsius. To prepare the ACBM for use during surgery, the product was placed unopened in a water bath containing warm sterile irrigant (saline or 5% Dextrose in Lactated Ringer’s Solution; 35 to 39 ℃; 95 to 102.2 ℉) to thaw. Once thawed (approximately 30 minutes), we decanted the cryopreservation solution and added 5% Dextrose in Lactated Ringer’s Solution to the indicated fill line. The product was mixed with all available morselized local bone packed within the interbody cage and implanted within 2 hours of thawing to ensure optimal cell viability. For single and two-level cases, the majority of cases (95%) utilized a small (1.2 cc) ACBM. For 3-level cases, 52% of cases utilized a small and 48% of cases used a medium (5.3 cc). In 4-level cases, all utilized a medium.
Surgical technique
All surgical procedures were conducted by one of two board-certified spine surgeons. The standard Smith-Robinson approach was employed. For the interbody fusion, a poly-ether-ketone-ketone (PEKK) interbody cage (Xtant, Belgrade, MT, USA) or a titanium truss interbody cage (4webMedical, Frisco, TX, USA) was utilized. These interbody cages were specifically utilized because of their hydrophilic properties. Additionally, both cages are three-dimensionally printed to have a surface topography conducive to bone on-growth. The interbody cages were meticulously packed with TE ACBM/local bone and placed within the intervertebral space. All procedures were supplemented with anterior plate fixation.
Radiographic assessment
The primary outcome measure was radiographic fusion rate at 6 and 12 months. Fusion rate was calculated as a percentage based on the number of patients with available imaging studies at that specific time. All radiographic measurements were performed using the institution’s PACS (Sectra-Workstation, Version 24.1) by a blinded, musculoskeletal-trained radiologist and a board-certified spinal surgeon reviewer. Any discrepancies were settled by a third, senior reviewer. To assess fusion, upright lateral flexion/extension radiographs and CT scans were utilized at the six-month and one-year follow-up intervals. Complete fusion was defined as: evidence of bridging bone across the disc space on CT, angular motion <3 degrees, and translational motion <2 mm on lateral radiographs. Fusion was only considered successful if all three of the criteria were achieved.
Segmental height was measured on plain radiographs as the distance from the most anterior and posterior points of the cephalad and caudal vertebral body, and the measurements were subsequently averaged. Subsidence was determined as a loss of segmental height >3 mm between the immediate and one-year post-operative follow up. Similarly, migration was defined as >3 mm displacement of the cage between the immediate and one-year post-operative follow-up. If subsidence and/or migration occurred, the segment could not be considered fused.
Safety assessment
All adverse events reported in the medical charts were evaluated for severity and causality (device-related or surgery-related) out to 15 months post-operatively. Occurrences of persistent neck and/or arm pain, paresthesia, weakness, and dysphagia were recorded. Device-related complications included migration, subsidence, or implant breakage. Secondary surgical procedures were classified as reoperation (procedure at the index level that does not involve modification of the device), revision (any procedure that adjusts, modifies, or removes part of the original implant configuration), removal (entire construct removed), or supplemental fixation [additional hardware is implanted at index level(s)].
Literature search
A literature search was conducted to establish a comparable historical control using PubMed with search terms for ACDF, anterior plate fixation, interbody cage, poly-ether-ether-ketone (PEEK), PEKK, titanium (Ti), and single-level, or multilevel cases. To be included, publications must have reported a single-level or multilevel ACDF procedure using a combination of PEEK, PEKK or Ti cage with supplemental anterior fixation. The evidence level, procedure type, graft material, study sample demographics, fusion assessment method, 12-month fusion rate, and complications (within 24 months) were recorded. Publications that were excluded were those that did not utilize anterior plate fixation, did not report fusion assessment method, and included ACDF procedures greater than four levels. The objective of this literature search was to provide context for our patient outcomes using ACBMs as the primary grafting option, in comparison to studies employing various alternative grafting options (autografts, allografts, BMP-2, and other ACBMs) in single- and multilevel ACDFs.
Statistical analysis
Demographic data, perioperative data, fusion rates, and surgical and device complications were quantified by the mean and standard deviation (SD). All statistical analyses, tables, and figures were generated using Excel (version 16.67, Microsoft Corporation).
Results
A total of 73 subjects (164 levels) were included in the study. The majority of cases were multilevel with 26% one-level, 32% two-level cases, 34% three-level cases, and 8% being four-level cases (Figure 1). At the 6-month follow-up, 69 individuals (94.5%) completed the radiological assessment. At 12 months, 39 (53.4%) individuals completed the radiological assessment. Regarding 12-month patient accountability, there were no reported deaths, two patients underwent supplemental fixation in the first 12 months post-operatively thereby excluding them from the 12-month fusion assessment, 13 patients were fused at 6 months and did not seek additional follow-up, and 19 did not return for follow-up.
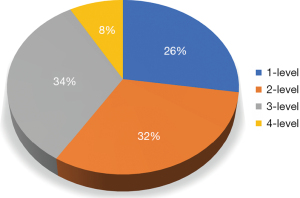
Demographic data
As depicted in Table 1, the mean age of the patient cohort was 54.6, with a range from 31 to 77 years of age. The cohort comprised of 50 (68.5%) males and 23 females (31.5%). The average body mass index (BMI) was 28.9 (±4.9) kg/m2. In terms of BMI classification, 13 (17.8%) patients were classified as normal weight, 33 (45.2%) patients were categorized as overweight, and the remaining 27 (37.0%) patients were obese. Among the cohort, 4 patients (5.5%) were current smokers, 22 subjects (30.1%) were former smokers, and 47 patients (64.4%) were nonsmokers. Perioperative characteristics included a median post-operative length of stay of 2 days, a median EBL of 40 mL, and a median operative time of 160 minutes (Table 1).
Table 1
Demographic/perioperative category | Amount (n=73) |
---|---|
Sex, n (%) | |
Male | 50 (68.5) |
Female | 23 (31.5) |
Age (years), mean (SD) [range] or n (%) | 54.6 (11.4) [31–77] |
≤50 | 25 (34.2) |
>50 to <65 | 37 (50.7) |
≥65 | 11 (15.1) |
BMI (kg/m2), mean (SD) [range] or n (%) | 28.9 (4.9) [19.1–42.1] |
Normal (18–24.9) | 13 (17.8) |
Overweight (25–29.9) | 33 (45.2) |
Obese (≥30) | 27 (37.0) |
Smoking status, n (%) | |
Current | 4 (5.5) |
Former | 22 (30.1) |
Never | 47 (64.4) |
Operated levels, mean (SD) | |
Vertebral levels | 2.25 (0.9) |
Perioperative data, median [IQR] | |
Estimated blood loss (mL) | 40 [25–50] |
Operative time (minutes) | 160 [130–180] |
Length of stay (days) | 2 [1–3] |
Graft volume (mL), mean | |
1-level | 1.6 |
2-level | 1.2 |
3-level | 3.2 |
4-level | 5.3 |
Total | 2.2 |
SD, standard deviation; BMI, body mass index; IQR, interquartile range.
Fusion rates
Of the 94.5% of patients completing 6 months follow up, the overall fusion rate was 45% (1-level: 50%, 2-level: 39.1%, 3-level: 54.5%, and 4-level: 16.7%, Figure 2). Of the 53.4% of patients completing 12 months follow-up, the overall fusion rate was 97.4% (1-level: 100%, 2-level: 100%, 3-level: 92.3%, and 4-level: 100%, Figure 3). For the 12-month effective (completers and non-completers) fusion rate, if we consider all lost to follow-up based on their 6-month status, the lower-bound fusion rate for the entire cohort was 71%. Only one case, a 3-level patient was assessed as not fused at 12 months. Conversely, the radiographic progression for a patient who underwent a successful 3-level fusion with symptomatic relief is shown in Figure 4A-4D.
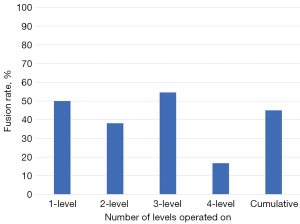
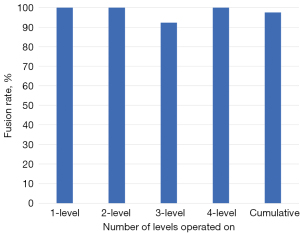
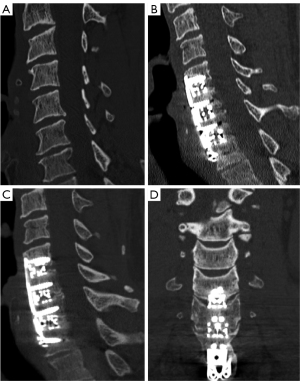
Surgical and device complications
There were no cases of migration or subsidence of the cages at any of the levels. The most common complaint following surgery was neck/arm pain with 18 (24.7%) patients at 6 months and 7 (9.6%) patients experiencing ongoing pain by 15 months post-operatively (Table 2). However, there was no record of intensity or frequency of the pain. Three patients (4.1%) had post-operative pain injections at an average of 12.1 months post-operatively. One patient had a single injection, the second patient had 3 injections concurrently, and the third patient had 6 injections over the 15-month period. None of the injection patients had secondary surgical intervention. At the latest documented follow-up, 9 (12.3%), 5 (6.8%), and 0 patients experienced, paresthesia, weakness, and dysphagia, respectively (Table 2).
Table 2
Complication | 6 months | 12 months | 15+ months |
---|---|---|---|
Surgery related, n (%) | |||
Unresolved neck/arm pain | 18 (24.7) | 12 (16.4) | 7 (9.6) |
Paresthesia | 15 (20.5) | 9 (12.3) | 9 (12.3) |
Weakness | 13 (17.8) | 7 (9.6) | 5 (6.8) |
Dysphagia | 4 (5.5) | 1 (1.4) | 0 |
Device related | |||
Subsidence | 0 | 0 | 0 |
Migration | 0 | 0 | 0 |
Secondary surgeries, n (%) | |||
Removal | 0 | 0 | 0 |
Reoperations | 0 | 0 | 0 |
Revisions | 0 | 0 | 0 |
Supplemental fixation | 2 (2.7) | 0 | 0 |
Secondary surgical interventions
There were no instances of reoperation, removal or revision to the original construct (Table 2). Two patients (2.7%) underwent a secondary surgical intervention within the first year post-operatively that required supplemental fixation (Table 2). The first case was a 54-year-old non-smoker who underwent a 4-level ACDF secondary to a fall. At 8 months post-operatively, she reported recurrent neck and upper extremity pain with a possible pseudoarthrosis at C6–C7 seen on imaging. At 11 months, posterior supplemental fixation with lateral mass screws was added at C6–C7. At 16 months (5 months post-operatively from second surgery), pain issues were resolved, and fusion was achieved (Figure 5A-5D). The second case was a 73-year-old male who underwent a C4–C7 fusion for degenerative changes resulting in radiculopathy and myelopathy. The patient had a severe fall at 5 months post-operative resulting in an unstable C2 vertebral fracture extending into a left side pars fracture. The patient then underwent a secondary surgery from C1–C6 involving additional posterior supplemental fixation. At the last follow-up, the patient was fused.
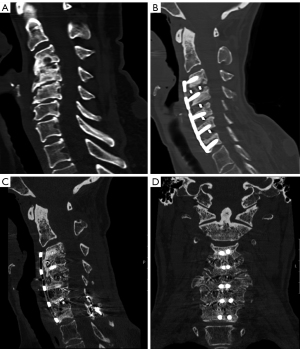
Discussion
In a real-world consecutive cohort of 73 patients undergoing an ACDF procedure treated with TE ACBM at up to 4 levels, 97.4% of patients with available imaging demonstrated fusion at 12 months post-operatively. There were no complications related to the graft material. Two patients required additional supplemental fixation and were excluded from radiographic analysis, although both went on to have satisfactory outcomes. Of the remaining population, the TE ACBM graft in conjunction with an osteoconductive interbody spacer demonstrated fusion rates of 100%, 100%, 92.3%, and 100% in one-, two-, three-, and four-level ACDFs, respectively. This study serves as preliminary evidence in support of the usage of ACBMs in cervical pathologies involving multiple spinal levels.
Given the multitude of bone graft materials available to surgeons, a literature review was conducted to compare our studies fusion outcomes to studies employing a comparable approach and instrumentation (Table 3).
Table 3
Study | Evidence level | Procedure | Graft | Study population† | Fusion assessment method | Fusion rate at 1 year | Graft/device-related complications, n (%) |
---|---|---|---|---|---|---|---|
Murrey et al. 2009 (37) | I | 1-level ACDFs with allograft bone spacers & anterior plate fixation | Local autograft | • N=106 in ACDF group | • Increased or maintained bone density at the site | 90.2% | • Secondary surgery: 9 (8.5%) |
• Age: 43.5±7.1 years | • No motion (<2 degrees) | o Revision: 5 (4.7%) | |||||
• 46.2% M | • >50% of trabecular bridging or bone mass maturation | o Removals: 0 | |||||
• 53.8% F | • No visible gaps in fusion mass | o Reoperations: 1 (0.9%) | |||||
• BMI: 27.3±5.5 kg/m2 | • No loss of disc height >3 mm | o Supplemental fixation: 3 (2.8%) | |||||
• 34.9% smokers | • Subsidence & migration: 2 (1.9%) | ||||||
Radcliff et al. 2017 (38) | I | 1 and 2-level ACDF with interbody cage and anterior fixation | Allograft | • 1-level: | • <2 degrees of segmental motion in flexion-extension | 1-level: 95.5%; 2-level: 90.9% | • 1-level: |
o N=81 | • Evidence of bridging bone across disc space | o Secondary surgery: 10 (12.3%) | |||||
o Age: 44±8.2 years | • Radiolucent lines at no more than 50% of the graft vertebral interfaces | □ Removal: 7 (8.6%) | |||||
o 44.4% M | □ Additional fixation 3: (3.7%) | ||||||
o 55.6% F | o Pseudoarthrosis: 5 (6.2%) | ||||||
o BMI: 27.4±4.2 kg/m2 | • 2-level: | ||||||
• 2-level: | o Secondary surgery: 17 (16.2%) | ||||||
o N=105 | □ Removal: 8 (7.5%) | ||||||
o Age: 46.2±8 years | □ Revision: 4 (3.74%) | ||||||
o 42.9% M | □ Additional fixation: 3 (2.80%) | ||||||
o 57.1% F | □ Reoperation: 2 (1.87%) | ||||||
o BMI: 28.1±4.2 kg/m2 | o Pseudoarthrosis: 9 (8.41%) | ||||||
Buttermann 2008 (39) | III | 1–3 level ACDF with cage and anterior fixation | Either ICBG or rh-BMP2 + allograft | • ICBG: | • Trabecularization across disc space | 92% | • ICBG: |
o N=36 | • <1-mm gapping of spinous processes on flexion/extension films | o Pseudoarthrosis: 2 (5.6%) | |||||
o Age: 48±9 years | o Delayed union: 1 (2.8%) | ||||||
o 33% M | o Graft site complications (infection & fracture): 2 (5.7%) | ||||||
o 66% F | • Rh-BMP2 + allograft: | ||||||
o 53% smokers | o Dysphagia: 15 (50%) | ||||||
• Rh-BMP2 + allograft: | o Readmission to ICU: 3 (10%) | ||||||
o N=30 | o Pseudoarthrosis: 1 (3.3%) | ||||||
o Age: 49±10 years | |||||||
o 50% M | |||||||
o 50% F | |||||||
o 37% smokers | |||||||
Fraser & Härtl 2007 (40) | III (meta-analysis) | 1–3 level ACDF with interbody cage and plate | Either allograft, local autograft, or ICBG | • N=2,682 | • Varied between studies | 1-level: 97.1%; 2-level: 94.6%; 3-level: 82.5% | • Pseudoarthrosis: |
• Mean age: 46.7 years | o 1-level: 10 (2.9%) | ||||||
• 53.4% M | o 2-level: 10 (5.4%) | ||||||
• 46.6% F | o 3-level: 7 (17.5%) | ||||||
Vanichkachorn et al. 2016 (32) | IV | 1-level ACDF with PEEK cages and anterior fixation | ACBM only | • N=31 | • Prescence of bridging bone across adjacent endplates on thin cut CT scans | 93.5% | • No additional surgeries in study period |
• Age: 48.9±8.1 years | • Less than 4 degrees of angular motion on flexion/extension X-ray | • 5 adverse events deemed possibly related to the ACBM | |||||
• 61% M | |||||||
• 39 % F | |||||||
• 16.1% smokers | |||||||
Peppers et al. 2017 (31) | IV | 2-level ACDF. With PEEK cages and anterior fixation | ACBM only | • N=40 | • Prescence of bridging bone across adjacent endplates on thin cut CT scans | 89.4% | • No complications or additional surgeries in study period |
• Age: 48.5±9 years | • Less than 4 degrees of angular motion on flexion/extension X-ray | ||||||
• 27.5% M | • Both levels needed fusion | ||||||
• 72.5% F | |||||||
• 45% smokers | |||||||
Laratta et al. 2018 (41) | IV | 3- or 4-level ACDF with PEEK or Ti cage and anterior fixation | Local autograft or allograft | • N=46 | • Not stated | 76% | • 24% nonunion rate |
• Age: 55.9±10.12 years | • 35% return to surgery | ||||||
• 46% M | |||||||
• 54% F | |||||||
• 22% smokers | |||||||
De la Garza-Ramos et al. 2016 (42) | IV | 3- or 4-level ACDF | Allograft or ICBG | • 3-level: | • Not stated | 3-level: 94.4%; 4-level: 84.6% | • 3-level: |
o N=71 | o Revision: 15 (21.1%) | ||||||
o Age: 52.3±9.7 years | o Pseudoarthrosis: 4 (5.6%) | ||||||
o 52.1% M | o Dysphagia: 9 (12.7%) | ||||||
o 47.9% F | o Neck Pain: 22 (31%) | ||||||
o 18.3% smokers | • 4-level: | ||||||
• 4-level: | o Revision: 5 (19.2%) | ||||||
o N=26 | o Pseudoarthrosis: 4 (15.4%) | ||||||
o Age: 57.2±8.8 years | o Dysphagia: 8 (30.8%) | ||||||
o 50% M | o Neck pain: 14 (53.8%) | ||||||
o 50% F | |||||||
o 11.5% smokers |
†, data of age and BMI are presented as mean ± standard deviation. ACDF, anterior cervical discectomy and fusion; M, male; F, female; BMI, body mass index; ICBG, iliac crest bone graft; rh-BMP2, recombinant human-bone morphogenetic protein 2; ICU, intensive care unit; PEEK, poly-ether-ether-ketone; ACBM, allograft cellular bone matrix; CT, computed tomography; Ti, titanium.
Murrey et al. (37) reported local autograft fusion rates for single level ACDF to be 90.2%, while Fraser & Hartl’s meta-analysis (40) illustrated fusion rates ranging from 97.1% for 1-level ACDF to 82.5% for 3-level ACDF with either ICBG or allograft supplemented with plate fixation. Moreover, Chau and Mobbs reported that autograft fusion rates for single level ACDF typically range from 83–99%, but this rate decreases significantly with increasing number of levels fused (43,44). This point is further supported by Laratta et al. (41) who reported a fusion rate of 76% for 3- or 4-level ACDF with autograft or allograft, while De la Garza-Ramos et al. (42) reported increased fusion rates of 94.4% and 84.6% for 3- and 4-level ACDF with autograft or allograft, respectively. Allograft alone has previously demonstrated pseudoarthrosis rates greater than 50% in 3- and 4-level ACDF patient cohorts (45). Our ACBMs results are comparable with reported autograft fusion rates and increased compared to allograft fusion rates (45). Furthermore, rh-BMP2 can serve as a highly effective grafting option for single- and multilevel ACDFs (39), but the U.S. Food and Drug Administration has issued a black box warning listing a life-threatening risk for edema related to use in the cervical region (46-48). Regarding other ACBMs, McAnany et al. (34) demonstrated a fusion rate of 87.7% for the Osteocel ACBM, compared to 94.7% in their control allograft group for ACDF procedures; however, this analysis exclusively consisted of single or two-level constructs. Previous investigations focusing on the Trinity Evolution established fusion rates of 94% for single-level ACDFs, and 89.4% for two-level ACDFs, respectively (31,32). Similarly, Eastlack et al. (12) reported an 87% fusion rate while investigating the utility of the Osteocel Plus ACBM in one or two level ACDF, while Kim et al. (33) reported a fusion rate of 88.1% for the ViBone ACBM in 1–3 level ACDFs. The results of this cohort are comparable to other reported ACBM literature. This study provides confidence for ACBM as a grafting option, particularly for patients undergoing multilevel ACDF.
As recent issues with bacterial contamination of other ACBM emerge (49), review of the complications to establish the safety profile for TE was vitally important. There were no device-related complications or unexpected adverse events related to the graft material. Further, this study demonstrated low rates of secondary procedures and post-operative dysphagia. All incidences of reported pain were noted but the majority were minor with 3 patients (4.1%) requiring injections. Notably, secondary procedures occurred in 2 patients (2.7% incidence). Based on our literature review, the secondary surgery rate for single-level ACDF with either autograft (local or ICBG) or allograft ranged from 2.9% to 12.3% (37-40). Comparatively, secondary procedure rates spanned from 5.4% to 35% for multilevel ACDF with autograft or allograft (38-42). It is important to note that two previous studies examining the 2nd generation, Trinity Evolution ACBM, in single- and two-level ACDF found no additional surgeries (31,32). Our secondary procedure rate compares favorably to autograft, and allografts included in our literature review but is increased compared to studies examining prior iterations of the Trinity ACBM (11,22,37). The incidence of dysphagia was 5.5% at 6 months post-operatively and 1.4% 12 months post-operatively, which aligns with the reported rates of post-operative dysphagia in 1.7–9.5% of ACDF patients (50). Several studies corroborate the heightened risk of dysphagia in multilevel procedures, with dysphagia rates as high as 30.8% in 4-level ACDFs (42,51,52). Our patient cohort experienced post-operative pain with 18 (24.7%), 12 (16.4%), and 7 (9.6%) patients experiencing ongoing pain at 6, 12, and 15 months post-operatively, respectively. These rates are higher than post-operative pain rates reported by Epstein (50) in a recent review on ACDF outcomes. However, studies examining outcomes in 3- and 4-level ACDFs have reported persistent post-operative neck pain in 11.6% to 49% of patients (53,54). Therefore, it is plausible that the high level of post-operative neck pain are in part due to the high amount of multilevel procedures in our cohort.
When considering bone graft alternatives for spinal fusions, especially in multilevel procedures, it’s crucial to evaluate the cost-effectiveness of ACBMs. The estimated cost of ACBMs is $525 per cm3, which is significantly more expensive than DBM, cancellous bone chips, and autograft, but less than rhBMP-2 which is widely used for lumbar fusions (55). Despite the greatest absolute cost, a cost-effectiveness study utilizing a Markov decision model demonstrated rhBMP-2 had the lowest cost per quality adjusted life year (QALY), likely due to decreased revision procedures (56), justifying its usage. No studies have directly examined the cost-effectiveness of ACBMs in spinal fusion procedures. Future investigations on this topic would be beneficial to determine if the increased cost of ACBMs is worthwhile. In the present study, only 2 patients (2.7%) required secondary surgery, demonstrating high durability and low rates of costly secondary procedures associated with the use of ACBMs in this cohort.
It is crucial to acknowledge several limitations inherent in this retrospective case series. Due to the retrospective design, it was not feasible to establish a control (autograft) group. To compensate for this limitation, a literature search was conducted to provide a contextual framework for our fusion rate and complication profile. Another limitation of this study was the unavailability of comprehensive patient-reported outcome measures (PROMs) which would have given more comprehensive context to reported pain. To address this limitation, we analyzed patient complications as reported in physician notes at multiple post-operative timepoints, thereby increasing our understanding of the safety profile associated with the investigated procedure and graft. Finally, it is important to acknowledge that our radiographic data was limited to one year post-operatively. Shriver et al.’s meta-analysis (57) revealed a variance in pseudarthrosis rates between randomized controlled trials (RCTs) (4.8%, 95% CI: 2.6–7.0%) and prospective cohort studies (0.2%, 95% CI: 0.1–0.5%), suggesting that the differences in fusion criteria and longer follow-ups of RCT better characterize pseudoarthrosis rates. Therefore, we utilized more stringent fusion criteria similar to RCTs that grade the progression of the fusion (bony bridging), the segmental motion, and the translation.
Conclusions
This study examined the fusion rate and safety profile associated with the TE ACBM in 1–4 level ACDF procedures. Fusion rates were comparable to the historical standard, autograft, at the 12-month time point. This study demonstrated low rates of secondary procedures (2.7%) and post-operative dysphagia (1.4%) at the 12-month follow-up. These findings provide evidence for the usage of ACBMs, as an alternative to autograft, in multilevel (up to 4-level) ACDF procedures. Future prospective, comparative studies between ACBMs and autograft are needed to prove safety, and efficacy of ACBMs in single and multilevel ACDF procedures.
Acknowledgments
We would like to acknowledge support from the Finn and Barbara Caspersen fellowship.
Funding: None.
Footnote
Reporting Checklist: The authors have completed the STROBE reporting checklist. Available at https://jss.amegroups.com/article/view/10.21037/jss-23-142/rc
Data Sharing Statement: Available at https://jss.amegroups.com/article/view/10.21037/jss-23-142/dss
Peer Review File: Available at https://jss.amegroups.com/article/view/10.21037/jss-23-142/prf
Conflicts of Interest: All authors have completed the ICMJE uniform disclosure form (available at https://jss.amegroups.com/article/view/10.21037/jss-23-142/coif). A.A.S. reports the royalties from Ortho Development Corp, consulting fees from Clariance Inc., Depuy Synthes Products Inc., Kuros Biosciences, and Ortho Development Inc.; the ownership interest with Centinel Spine, HSS ASC Development Network LLC, HS2 LLC, ISPH 3 Holdings LLC, ISPH II LLC, VBros Venture Partners X, Vestia Ventures MiRus Investment, LLC. He also participated in the Scientific Advisory Board of Clariance Inc., Depuy Synthes Products Inc., and Kuros Biosciences. FPC reports the royalties from Accelus-Consultant, consulting fees from 4Web Medical, Synexis LLC, NuVasive Inc., Spine Biopharma LLC; ownership interest with 4Web Medical, HealthPoint Capital Partners LP, Ivy Healthcare Capital Partners LLC, ISPH 3 Holdings LLC, ISPH II LLC, Orthobond Corp, Spine Biopharma, Tissue Differentiation Intelligence LLC, VBVP VI LLC, VBVP X LLC, Woven Orthopedic Technologies. He also participates in 4Web Medical, HealthPoint Capital Partners LP, Orthobond Corp., and Wove Orthopedic Technologies. CA reports consulting fees from Camber Spine Tech, and Centinel Spine Tech; ownership interest with Orthobond Corporation. She also participates in the advisory board of Camber Spine Tech and Orthobond Corporation. The other authors have no conflicts of interest to declare.
Ethical Statement: The authors are accountable for all aspects of the work in ensuring that questions related to the accuracy or integrity of any part of the work are appropriately investigated and resolved. Ethical approval was obtained from the Hospital for Special Surgery IRB (No. 2023-1567). Participants did not have to give informed consent due to the retrospective nature of the study it was determined there was no risk to the patient. The study was conducted in accordance with the Declaration of Helsinki (as revised in 2013).
Open Access Statement: This is an Open Access article distributed in accordance with the Creative Commons Attribution-NonCommercial-NoDerivs 4.0 International License (CC BY-NC-ND 4.0), which permits the non-commercial replication and distribution of the article with the strict proviso that no changes or edits are made and the original work is properly cited (including links to both the formal publication through the relevant DOI and the license). See: https://creativecommons.org/licenses/by-nc-nd/4.0/.
References
- Waheed MA, Hasan S, Tan LA, et al. Cervical spine pathology and treatment: a global overview. J Spine Surg 2020;6:340-50. [Crossref] [PubMed]
- Buser Z, Ortega B, D'Oro A, et al. Spine Degenerative Conditions and Their Treatments: National Trends in the United States of America. Global Spine J 2018;8:57-67. [Crossref] [PubMed]
- Luyao H, Xiaoxiao Y, Tianxiao F, et al. Management of Cervical Spondylotic Radiculopathy: A Systematic review. Global Spine J 2022;12:1912-24. [Crossref] [PubMed]
- Radhakrishnan K, Litchy WJ, O'Fallon WM, et al. Epidemiology of cervical radiculopathy. A population-based study from Rochester, Minnesota, 1976 through 1990. Brain 1994;117:325-35. [Crossref] [PubMed]
- Vonck CE, Tanenbaum JE, Smith GA, et al. National Trends in Demographics and Outcomes Following Cervical Fusion for Cervical Spondylotic Myelopathy. Global Spine J 2018;8:244-53. [Crossref] [PubMed]
- Marawar S, Girardi FP, Sama AA, et al. National trends in anterior cervical fusion procedures. Spine (Phila Pa 1976) 2010;35:1454-9. [Crossref] [PubMed]
- Lopez CD, Boddapati V, Lombardi JM, et al. Recent trends in medicare utilization and reimbursement for anterior cervical discectomy and fusion. Spine J 2020;20:1737-43. [Crossref] [PubMed]
- Weiss HK, Yamaguchi JT, Garcia RM, et al. Trends in National Use of Anterior Cervical Discectomy and Fusion from 2006 to 2016. World Neurosurg. 2020;138:e42-e51. [Crossref] [PubMed]
- Neifert SN, Martini ML, Yuk F, et al. Predicting Trends in Cervical Spinal Surgery in the United States from 2020 to 2040. World Neurosurg 2020;141:e175-81. [Crossref] [PubMed]
- Floyd T, Ohnmeiss D. A meta-analysis of autograft versus allograft in anterior cervical fusion. Eur Spine J 2000;9:398-403. [Crossref] [PubMed]
- Tuchman A, Brodke DS, Youssef JA, et al. Autograft versus Allograft for Cervical Spinal Fusion: A Systematic Review. Global Spine J 2017;7:59-70. [Crossref] [PubMed]
- Eastlack RK, Garfin SR, Brown CR, et al. Osteocel Plus cellular allograft in anterior cervical discectomy and fusion: evaluation of clinical and radiographic outcomes from a prospective multicenter study. Spine (Phila Pa 1976) 2014;39:E1331-7. [Crossref] [PubMed]
- Sasso RC, LeHuec JC, Shaffrey CSpine Interbody Research Group. Iliac crest bone graft donor site pain after anterior lumbar interbody fusion: a prospective patient satisfaction outcome assessment. J Spinal Disord Tech 2005;18:S77-S81. [Crossref] [PubMed]
- Heneghan HM, McCabe JP. Use of autologous bone graft in anterior cervical decompression: morbidity & quality of life analysis. BMC Musculoskelet Disord 2009;10:158. [Crossref] [PubMed]
- McLain RF, Techy F. Trephine Technique for Iliac Crest Bone Graft Harvest: Long-term Results. Spine (Phila Pa 1976) 2021;46:41-7. [Crossref] [PubMed]
- Fourman MS, Vaynrub M. Identifying the ideal trajectory for prone trephine iliac crest bone graft harvesting. Spine J 2023;23:1571-3. [Crossref] [PubMed]
- Viola A 3rd, Appiah J, Donnally CJ 3rd, et al. Bone Graft Options in Spinal Fusion: A Review of Current Options and the Use of Mesenchymal Cellular Bone Matrices. World Neurosurg 2022;158:182-8. [Crossref] [PubMed]
- Kerezoudis P, Alvi MA, Freedman BA, et al. Utilization Trends of Recombinant Human Bone Morphogenetic Protein in the United States. Spine (Phila Pa 1976) 2021;46:874-81. [Crossref] [PubMed]
- Roberts TT, Rosenbaum AJ. Bone grafts, bone substitutes and orthobiologics: the bridge between basic science and clinical advancements in fracture healing. Organogenesis 2012;8:114-24. [Crossref] [PubMed]
- Darveau SC, Leary OP, Persad-Paisley EM, et al. Existing clinical evidence on the use of cellular bone matrix grafts in spinal fusion: updated systematic review of the literature. Neurosurg Focus 2021;50:E12. [Crossref] [PubMed]
- Singh K, Ahmadinia K, Park DK, et al. Complications of spinal fusion with utilization of bone morphogenetic protein: a systematic review of the literature. Spine (Phila Pa 1976) 2014;39:91-101. [Crossref] [PubMed]
- Arnold PM, Anderson KK, Selim A, et al. Heterotopic ossification following single-level anterior cervical discectomy and fusion: results from the prospective, multicenter, historically controlled trial comparing allograft to an optimized dose of rhBMP-2. J Neurosurg Spine 2016;25:292-302. [Crossref] [PubMed]
- Tannoury CA, An HS. Complications with the use of bone morphogenetic protein 2 (BMP-2) in spine surgery. Spine J 2014;14:552-9. [Crossref] [PubMed]
- Balseiro S, Nottmeier EW. Vertebral osteolysis originating from subchondral cyst end plate defects in transforaminal lumbar interbody fusion using rhBMP-2. Report of two cases. Spine J 2010;10:e6-e10. [Crossref] [PubMed]
- Smoljanovic T, Cimic M, Bojanic I. Aggressive end plate decortication as a cause of osteolysis after rhBMP-2 use in cervical spine interbody fusion. Spine J 2010;10:187-8; author reply 188. [Crossref] [PubMed]
- Chau AM, Xu LL, Wong JH, et al. Current status of bone graft options for anterior interbody fusion of the cervical and lumbar spine. Neurosurg Rev 2014;37:23-37. [Crossref] [PubMed]
- Chen F, He W, Mahaney K, et al. Alternative grafts in anterior cervical fusion. Clin Neurol Neurosurg 2013;115:2049-55. [Crossref] [PubMed]
- Golubovsky JL, Ejikeme T, Winkelman R, et al. Osteobiologics. Oper Neurosurg (Hagerstown) 2021;21:S2-9. [Crossref] [PubMed]
- Kadam A, Millhouse PW, Kepler CK, et al. Bone substitutes and expanders in Spine Surgery: A review of their fusion efficacies. Int J Spine Surg 2016;10:33. [Crossref] [PubMed]
- Skovrlj B, Guzman JZ, Al Maaieh M, et al. Cellular bone matrices: viable stem cell-containing bone graft substitutes. Spine J 2014;14:2763-72. [Crossref] [PubMed]
- Peppers TA, Bullard DE, Vanichkachorn JS, et al. Prospective clinical and radiographic evaluation of an allogeneic bone matrix containing stem cells (Trinity Evolution® Viable Cellular Bone Matrix) in patients undergoing two-level anterior cervical discectomy and fusion. J Orthop Surg Res 2017;12:67. [Crossref] [PubMed]
- Vanichkachorn J, Peppers T, Bullard D, et al. A prospective clinical and radiographic 12-month outcome study of patients undergoing single-level anterior cervical discectomy and fusion for symptomatic cervical degenerative disc disease utilizing a novel viable allogeneic, cancellous, bone matrix (trinity evolution™) with a comparison to historical controls. Eur Spine J 2016;25:2233-8. [Crossref] [PubMed]
- Kim PD, Raiszadeh R, Bomback DA, et al. 12-Month clinical and radiographic outcomes of ViBone viable bone matrix in patients undergoing cervical and lumbar spinal fusion surgery. J Orthop Surg Res 2023;18:239. [Crossref] [PubMed]
- McAnany SJ, Ahn J, Elboghdady IM, et al. Mesenchymal stem cell allograft as a fusion adjunct in one- and two-level anterior cervical discectomy and fusion: a matched cohort analysis. Spine J 2016;16:163-7. [Crossref] [PubMed]
- Diaz RR, Savardekar AR, Brougham JR, et al. Investigating the efficacy of allograft cellular bone matrix for spinal fusion: a systematic review of the literature. Neurosurg Focus 2021;50:E11. [Crossref] [PubMed]
- Trinity Elite Allograft with Viable Cells - Orthofix . [cited 2024 Mar 11]. Available online: https://orthofix.com/products/spine-solutions/spine-procedures/anterior-cervical-fixation/trinity-elite-allograft-with-viable-cells/
- Murrey D, Janssen M, Delamarter R, et al. Results of the prospective, randomized, controlled multicenter Food and Drug Administration investigational device exemption study of the ProDisc-C total disc replacement versus anterior discectomy and fusion for the treatment of 1-level symptomatic cervical disc disease. Spine J 2009;9:275-86. [Crossref] [PubMed]
- Radcliff K, Davis RJ, Hisey MS, et al. Long-term Evaluation of Cervical Disc Arthroplasty with the Mobi-C© Cervical Disc: A Randomized, Prospective, Multicenter Clinical Trial with Seven-Year Follow-up. Int J Spine Surg 2017;11:31. [Crossref] [PubMed]
- Buttermann GR. Prospective nonrandomized comparison of an allograft with bone morphogenic protein versus an iliac-crest autograft in anterior cervical discectomy and fusion. Spine J 2008;8:426-35. [Crossref] [PubMed]
- Fraser JF, Härtl R. Anterior approaches to fusion of the cervical spine: a metaanalysis of fusion rates. J Neurosurg Spine 2007;6:298-303. [Crossref] [PubMed]
- Laratta JL, Reddy HP, Bratcher KR, et al. Outcomes and revision rates following multilevel anterior cervical discectomy and fusion. J Spine Surg 2018;4:496-500. [Crossref] [PubMed]
- De la Garza-Ramos R, Xu R, Ramhmdani S, et al. Long-term clinical outcomes following 3- and 4-level anterior cervical discectomy and fusion. J Neurosurg Spine 2016;24:885-91. [Crossref] [PubMed]
- Chau AM, Mobbs RJ. Bone graft substitutes in anterior cervical discectomy and fusion. Eur Spine J 2009;18:449-64. [Crossref] [PubMed]
- Fernyhough JC, White JI, LaRocca H. Fusion rates in multilevel cervical spondylosis comparing allograft fibula with autograft fibula in 126 patients. Spine (Phila Pa 1976) 1991;16:S561-4. [Crossref] [PubMed]
- Wewel JT, Kasliwal MK, Adogwa O, et al. Fusion rate following three- and four-level ACDF using allograft and segmental instrumentation: A radiographic study. J Clin Neurosci 2019;62:142-6. [Crossref] [PubMed]
- MEDCAC Meeting - On Label and Off-Label Use of Bone Morphogenetic Proteins (BMPs) (09/22/2010) . [cited 2023 Nov 30]. Available online: https://www.cms.gov/medicare-coverage-database/view/medcac-meeting.aspx?medcacid=55
- Lu DC, Tumialán LM, Chou D. Multilevel anterior cervical discectomy and fusion with and without rhBMP-2: a comparison of dysphagia rates and outcomes in 150 patients. J Neurosurg Spine 2013;18:43-9. [Crossref] [PubMed]
- James AW, LaChaud G, Shen J, et al. A Review of the Clinical Side Effects of Bone Morphogenetic Protein-2. Tissue Eng Part B Rev 2016;22:284-97. [Crossref] [PubMed]
- McVeigh LG, Zaazoue MA, Lane BC, et al. Management and outcomes of surgical site tuberculosis infection due to infected bone graft in spine surgery: a single-institution experience and 1-year postoperative follow-up. J Neurosurg Spine 2023;38:281-92. [Crossref] [PubMed]
- Epstein NE. A Review of Complication Rates for Anterior Cervical Diskectomy and Fusion (ACDF). Surg Neurol Int 2019;10:100. [Crossref] [PubMed]
- Fountas KN, Kapsalaki EZ, Nikolakakos LG, et al. Anterior cervical discectomy and fusion associated complications. Spine (Phila Pa 1976) 2007;32:2310-7. [Crossref] [PubMed]
- Yee TJ, Swong K, Park P. Complications of anterior cervical spine surgery: a systematic review of the literature. J Spine Surg 2020;6:302-22. [Crossref] [PubMed]
- Kim S, Alan N, Sansosti A, et al. Complications After 3- and 4-Level Anterior Cervical Diskectomy and Fusion. World Neurosurg 2019;130:e1105-10. [Crossref] [PubMed]
- Jack MM, Lundy P, Reeves AR, et al. Four-level Anterior Cervical Discectomy and Fusions: Results Following Multilevel Cervical Fusion With a Minimum 1-Year Follow-up. Clin Spine Surg 2021;34:E243-7. [Crossref] [PubMed]
- Lambrechts MJ, Issa TZ, Mazmudar A, et al. Cellular Bone Matrix in Spine Surgery - Are They Worth the Risk: A Systematic Review. Global Spine J 2024;14:1070-81. [Crossref] [PubMed]
- Virk S, Sandhu HS, Khan SN. Cost effectiveness analysis of graft options in spinal fusion surgery using a Markov model. J Spinal Disord Tech 2012;25:E204-10. [Crossref] [PubMed]
- Shriver MF, Lewis DJ, Kshettry VR, et al. Pseudoarthrosis rates in anterior cervical discectomy and fusion: a meta-analysis. Spine J 2015;15:2016-27. [Crossref] [PubMed]