Real-world clinical accuracy of long cortical bone trajectory screw placement using a patient-specific template guide
Highlight box
Key findings
• This research assessed the accuracy of inserting long cortical bone trajectory screws using a patient-specific template guide with 100 patients who had received surgery on their lumbar spine.
• Overall, 382 cortical bone trajectory (CBT) screws (92.7%) were fully inside the pedicle and the mean depth of the screw in the vertebral body was 60.9%±8.1%.
What is known and what is new?
• CBT screws must be inserted correctly anterior to the vertebral body, however, its trajectory is narrow and malpositioning of screw is not rare. This study showed that a patient-specific template guide was simple, highly accurate, and dependable for insertion of a long CBT screw.
What is the implication, and what should change now?
• Especially for those unfamiliar with CBT, the use of this template guide can be a solution to safely insert the CBT screw into the anterior part of the spine.
Introduction
Cortical bone trajectory (CBT) screws have recently gained popularity as an alternative to the traditional trajectory technique of lumbar pedicle screw (PS) insertion (1-6). Contrary to the traditional PSs which adopt a pathway converging along the pedicle’s anatomical axis, the insertion method for CBT screws begins at the pars interarticularis, extends along a trajectory moving upward and laterally through the pedicle, and achieve stability by contacting the highest density of cortical bone (7). Because cortical bone is less susceptible to osteoporosis than the trabecular bone, CBT provides strong screw fixation even in osteoporotic vertebrae, presenting a viable surgical approach for addressing spinal conditions in an aging society. A key benefit of the CBT method lies in its ability to conduct the full operation—encompassing decompression, interbody fixation, and screw insertion—through a singular, small midline cut, with many clinical studies highlighting its minimally invasive aspect (8-10). However, there is ongoing debate about the effectiveness of the traditional CBT method, which uses screws 25–30 mm in length, with some reports of low compression force on the anterior column of the vertebra (11) and low fusion rates (12,13). Therefore, as a preventive strategy, it has recently been advised to utilize longer CBT screws, ranging from 40 to 45 mm, which are oriented further towards the vertebral body’s front (2,7,14).
A longer CBT screw is anticipated to enhance cortical engagement and efficient load distribution across the vertebra, which in turn should encourage bone fusion and diminish the likelihood of screw loosening. In addition, recently, depth of the screw in the vertebral body (% depth) has been reported to affect the rate of bone fusion in the cases of using CBT screws (15,16). Thus, ensuring an ideally long cortical trajectory is challenging because of the narrow corridor, which necessitates high-level surgical skills. A patient-specific template guide for long CBT screw placement may be a promising solution to improve safety and accuracy. This three-dimensional (3D) printing technology has been effectively utilized in the CBT, with both a cadaveric study and a clinical investigation reporting positive results in the accuracy of screw placement (17,18).
The advantage of a patient-specific guide is that everyone can perform screw insertion with the same precision and safety. However, although the number of CBT users has increased in the past decade, unlike PS, not many people are familiar with inserting CBT screws in a freehand manner or using only intraoperative radiographic examinations. Therefore, surgeons who use patient-specific template guides to insert CBT screws include those with little or no experience in using CBT screws themselves. Therefore, assessing the precision of placing long CBT screws with the aid of a patient-specific template guide in a clinical context is crucial.
The aim of this study was to examine the clinical accuracy of long CBT screw placement with the use of a patient-specific template guide. We present this article in accordance with the STROBE reporting checklist (available at https://jss.amegroups.com/article/view/10.21037/jss-23-122/rc).
Methods
Subjects
This study was a retrospective clinical evaluation of prospectively enrolled patients using a patient-specific guide for CBT placement following approval from our institutional ethics committee. The subjects included 100 consecutively enrolled patients (46 men and 54 women; age, 68.5±9.9 years; range, 38–86 years). These patients received posterior lumbar spinal fusion with the assistance of guides, performed by three spine surgeons, which were not familiar to insert CBT screws by freehand or using only intraoperative radiographic examination, from January 2019 to January 2022.
The exclusion criteria were reoperative surgery, and vertebrae with a history of osteoporotic fracture or infection. All the patients exhibited symptoms of neural compression. Of the 100 patients, 70 patients had degenerative spondylolisthesis; 18 patients had lumbar canal stenosis; 11 patients had foraminal stenosis, and one patient had intervertebral instability. There were no cases of deformities or severe scoliosis. In total, 412 screws were positioned utilizing the MySpine MC® (Medacta International SA, Casted San Pietro, Switzerland) system, which is a patient-specific guide for CBT placement. The number of screws implanted at each level of the spine was as follows: L2 (4 screws), L3 (40 screws), L4 (188 screws), and L5 (180 screws). Although there were cases in this study for which S1 was also included in the fixed range, the screws inserted in S1 were excluded from the analysis because they could not be considered as CBT.
Preoperative planning
Using the MySpine® system (accessible via the MySpine web planner), 3D models of each vertebra were generated from pre-surgical computed tomography (CT) scans with a 1.0 mm slice thickness. Once the CT of the required part is sent to the company, only the necessary parts are trimmed and an stereolithography conversion is performed using Solidworks 2020 (Dassault Systemes, Paris, France). The surgeon then utilized the MySpine web planner to create a detailed surgical strategy for the accurate placement of CBT screws, specifying the screws’ length, diameter, and orientation in both sagittal and transverse planes (Figure 1). In accordance with a previous study, the planned trajectory (long CBT) was set to start from the pars interarticularis, pass through the inferior border of the pedicle (7), and end around the front of the vertebral endplate as nearly as possible. Once the surgical plan was established, the bone data were sent to the 3D modeling software (MySpine). A patient-specific guide and 3D bone models were constructed with medical polyamide by a 3D printing system using selective laser sintering (Figure 2A,2B).
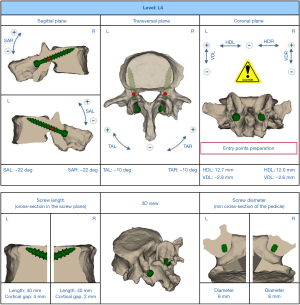
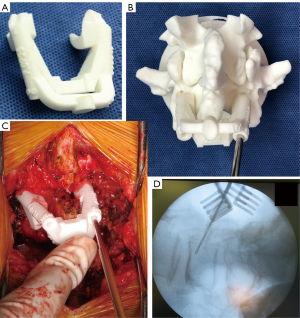
Surgical procedure
A midline incision was made above the targeted vertebrae, followed by the dissection of the paraspinal muscles to expose the lateral edges of the pars interarticularis. After thoroughly exposing the posterior bone surface, including both the central region and the caudal end of the lamina, which serve as the primary contact points for the guide (Figure S1), a surgical guide was positioned on top. At this point, it is essential to verify the stability and appearance of the guide placement on the actual lamina compared to the 3D model. This will help ensure there is no deviation in the axial direction. After inserting the drill into the guide, lateral fluoroscopic imaging was also employed to confirm both the planned entry point and trajectory. The initial penetration was carefully chosen to prevent disturbing any adjacent facet joint that was not fused, with the trajectory generally angled 20–25° upwards in the sagittal direction and 10° to the side in the axial direction, consistent with prior descriptions (7). Screw holes were then drilled to the specified depth on bilateral sides, and guide wires were inserted (Figure 2C,2D). Prior to inserting the screws, especially in the context of posterior lumbar interbody fusion, procedures such as posterior decompression, facetectomy, and the placement of two interbody cages were conducted to avoid interference from the medial placement of the screw heads. Finally, the path of the screw was tapped to the size of the planned screw to prevent cortical fissures from forming during screw insertion over the guidewire, followed by the insertion of the cancellous screw. The average screw sizes were 6.4±0.4 mm in diameter and 41.5 (range, 35–45) mm in length. In all cases, local and artificial bones were used for grafting.
Evaluation of screw position
The position of the CBT screws was independently assessed using conventional CT by two spine surgeons who had not performed the surgery. In this study, we analyzed CBT screws inserted from L2 to L5. Postoperative CT with a slice thickness of 1 mm was performed within 1 week of surgery. An axial image was obtained that included the full length of each screw. The screw deviations, encompassing both lateral and medial, were grouped into four distinct grades based on previously established criteria (19): no deviation, with the screw entirely within the pedicle; grade A signifies a deviation of less than 2 mm; grade B is assigned for deviations greater than 2 mm but less than 4 mm; and grade C is used for deviations exceeding 4 mm. The pre- and post-operative reconstructed vertebrae were then superimposed manually to analyze discrepancies between the planned and actual screw angles, screw positions and % depth using 3D image workstation software (Solidworks Software, Dassault Systèmes Company, Vélizy-Villacoublay, France). The deviations in the screw positions and angles were evaluated at the entry point in the axial and sagittal views, respectively (Figure 3). The deviation in the percent depth was evaluated using axial views (Figure 4). The deviations were compared at different levels of the lumbar spine.
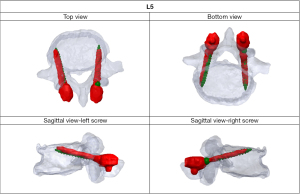
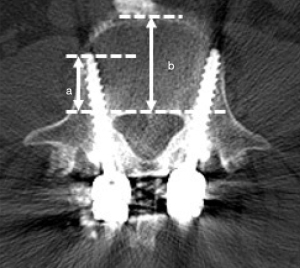
Statistical analysis
The data were analyzed using GraphPad Prism version 10 (GraphPad Software, San Diego, CA, USA). The normality of the data was tested using the Shapiro-Wilk test. The results were presented as the mean ± standard deviation. Differences in continuous variables among groups were assessed using the Mann-Whitney U test. To account for multiple testing in the four-group comparisons, a Bonferroni correction was performed to adjust the statistical significance to P<0.008 (i.e., 0.05/6).
Ethical considerations
The study was conducted in accordance with the Declaration of Helsinki (revised in 2013). Ethical approval was granted by Hokkaido Orthopedic Memorial Hospital (No. 106). The requirement for patient informed consent was waived, owing to the retrospective nature of the study and its deidentified data.
Results
Subjects
Out of 412 inserted screws, 382 (92.7%) were completely within the pedicle and the remaining 30 (7.2%) showed grade A inferomedial deviation. There were no grade B or C screw deviations (Table 1). The diameters of the screws inserted at each level are listed in Table 2. One hundred and eighty-five screws (44.9%) had a diameter of 7.0 mm, which is relatively thick compared to previous studies (15,20). The data for each measurement relating to the screw position, along with the corresponding values, are presented in Table 3. The average deviations between the planned and actual CBT positions at the entry point were measured at 0.88±0.73 mm vertically (sagittal plane) and 0.98±0.75 mm horizontally (axial plane). There were no significant differences in any deviation among the spinal levels. The mean angular deviations in the sagittal and axial planes were 2.30±1.87° and 2.66±2.02°, respectively. There was a significant difference in the axial angular deviation between L4 and L5 (P<0.001). The axial angular deviation tended to increase in the caudal vertebrae. Additionally, the direction of the actual screw trajectory’s deviation from the intended trajectory was analyzed. Sagittal angular deviation tended to be displaced cranially overall; however, among them, 82 (45.5%) CBT screws inserted into L5 deviated caudally compared to planned screws, which was greater than the other lumbar vertebrae. In terms of transverse angular deviation, there was a tendency for angular shifts to occur laterally in the lower lumbar vertebrae (L4 and L5) in comparison to the higher segments of the lumbar spine (Table 4).
Table 1
Level | No perforation | Grade A | Grade B | Grade C | Safety (% contain) |
---|---|---|---|---|---|
L2 | 4 | 0 | 0 | 0 | 4/4 (100.0) |
L3 | 38 | 2 | 0 | 0 | 38/40 (95.0) |
L4 | 177 | 11 | 0 | 0 | 177/188 (94.1) |
L5 | 163 | 17 | 0 | 0 | 163/180 (90.6) |
Total | 382 | 30 | 0 | 0 | 382/412 (92.7) |
Table 2
Screw diameter | L2 | L3 | L4 | L5 | Total |
---|---|---|---|---|---|
6.0 mm | 0 | 26 | 121 | 80 | 227 |
7.0 mm | 4 | 14 | 67 | 100 | 185 |
Total | 4 | 40 | 188 | 180 | 412 |
Table 3
Parameters | L2 | L3 | L4 | L5 | Total |
---|---|---|---|---|---|
∆Vertical deviation entry point (mm) | 0.21±0.19 | 0.78±0.79 | 0.82±0.56 | 1.02±0.88 | 0.88±0.73 |
∆Horizontal deviation entry point (mm) | 0.49±0.56 | 1.04±0.80 | 1.00±0.73 | 1.01±0.79 | 0.98±0.75 |
∆Sagittal angle (°) | 1.15±0.42 | 2.00±1.54 | 2.22±1.61 | 2.52±2.20 | 2.30±1.87 |
∆Axial angle (°) | 1.89±1.26 | 2.41±1.90 | 2.18±1.64 | 3.28±2.31* | 2.66±2.02 |
*, indicates the differences between the L4 and L5 in ∆ transversal angle (Mann-Whitney U test; P<0.001).
Table 4
Level | Total screws | Direction of angular deviation | |||
---|---|---|---|---|---|
Medial | Lateral | Cranial | Caudal | ||
L2 | 4 | 3 | 1 | 3 | 1 |
L3 | 40 | 22 | 18 | 24 | 16 |
L4 | 188 | 75 | 113 | 125 | 63 |
L5 | 180 | 38 | 142 | 98 | 82 |
Total | 412 | 138 | 274 | 250 | 162 |
The measurement of the % depth for each level is presented in Table 5. The mean % depth was 60.9%±8.1% and the mean % depth deviation was 9.6%±7.1% in total. In all vertebrae, the mean % depth was approximately 10% smaller for the actual screws than the planned screws. The mean % depth deviation of the L5 vertebra was smaller than that of the L4 vertebra (P=0.001). The patient showed no evidence of neurovascular complications related to the screws. An illustrative example is shown in Figure 5.
Table 5
Parameters | L2 | L3 | L4 | L5 | Total |
---|---|---|---|---|---|
Planned screw (%) | 78.5±4.5 | 69.4±6.8 | 68.6±11.9 | 67.4±9.3 | 68.2±10.4 |
Actual screw (%) | 69.8±6.2 | 59.9±8.7 | 60.4±8.3 | 61.6±7.6 | 60.9±8.1 |
Deviation (%) | 8.6±1.8 | 10.2±6.8 | 10.6±7.1 | 8.6±7.1* | 9.6±7.1 |
*, indicates the difference between L4 and L5 in the deviation of % depth (Mann-Whitney U test; P=0.001).
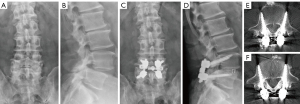
Discussion
This study investigated the real-world accuracy and safety of placing CBTs in the lumbar spine using a patient-specific guide. The result showed that mean % depth of the inserted screws was 60.9%±8.1%, which was approximately equivalent to the previous report (15). The findings indicated that 92.7% of the screws were precisely positioned within the pedicle. Earlier research (1,7,17,20-22) documented the incidence of screw perforation with CBT as follows: 20% using the freehand technique, 7.8% under fluoroscopic guidance, 7% with robotic assistance, and between 2.5% and 6.1% when utilizing a patient-specific template guide. Although the perforation rate was 7.3% in this study, no neurovascular injuries were observed and all deviated screws had grade A (<2 mm) inferomedial deviation. Moreover, this study used 185 screws (44.9%) with a 7 mm diameter, which is thicker than in previous studies; and this may be the reason for the slightly higher perforation of grade A. A navigation system also offers high puncture accuracy for CBT and are viable options. However, there are some differences between intraoperative navigation and patient-specific template guides. The patient-specific guide may have advantage of drilling error along with the change in vertebral rotation during the surgery due to the direct adjustment to the target vertebrae. In addition, navigation accuracy decreases as you move away from the reference frame, but patient-specific guides are designed for each vertebral level, so accuracy remains consistent. Another issue is cost. Intraoperative navigation is not available in all institutions and is expensive to implement. Therefore, patient-specific template guides serve as a convenient tool to perform CBT relatively safely in all hospitals. The use of this guide also provides a 3D model with holes drilled according to the created trajectory, making it easier to find a safe CBT trajectory during surgery by comparing it with the model, which is another one of its advantages. However, it should be noted that not every patient is suitable for this patient-specific template guide. For example, since this guide contacts the central part of the lamina and fits the inferior part of the lamina with hooks, if there are severe degenerative changes or bone union in the inferior part of the lamina, we must confirm the fit of the patient-specific guide before surgery by using the guide and the 3D model to determine whether the guide is reliable or not.
In the present report, a patient-specific template guide allowed for the deep insertion of the screw into the anterolateral side of the vertebral body. However, the mean % depth of the inserted screw compared to the planned screw was approximately 10% lesser regardless of the vertebral level. One reason for this is the characteristics of the planning software used to create the patient-specific template guide. The software is designed with headless screws at the planning stage, this is to ensure that the screw entry point is clearly visible. Skiving can occur intraoperatively if the screw entry is created in a steep area. However, because the actual CBT screw has a head and the trajectory of the CBT screw is inserted at an oblique angle to the bone, the head interferes with the bone when the CBT screw is inserted as deeply as planned, resulting in a smaller % depth than the planned screw. To prevent the actual screw depth from being shorter than planned, selecting a screw that is one size longer than the planned screw size and insert it shallowly will ensure that the screw head does not hit the lamina. The mean depth deviation of L4 was the largest and larger than that of L5 (P=0.001). One possible reason is that L4 was often a fixed cephalad vertebra and was placed shallowly to avoid violating the facet joints. Regarding the mean deviation between the planned and actual screw positions, L5 had the largest deviation in both the sagittal and axial angles compared with L2 and L4. This result is consistent with that of a previous report (17). Relative to the upper lumbar levels, the unique anatomical features of the lower lumbar levels, including the deeper surgical field, increased volume of paraspinal muscles, and more extensive degenerative alterations in the posterior elements, might have impacted the outcomes. In addition, 45.5% of the CBT screws inserted in L5 had a sagittal angular deviation directed caudally, which had the highest probability of deviating caudally of all. As L5 is often the most caudal part of the fixation, the skin and paraspinal muscles may also cause the screw to deviate caudally. It is important to ensure sufficient exposure to adequately fit patient-specific guides.
Finite element analysis has shown that a longer trajectory offers biomechanical advantages in the CBT screw. The long CBT, employing 40-mm screws, showed a 35% increase in pullout strength, compared to the original CBT with 25-mm screws (7). It has been reported that single level midline lumbar fusion, a novel minimally invasive surgery fusion technique through which interbody fusion can be achieved via limited posterior midline incision and posterior instrumentation using long CBT screws, resulted in a greater increase in the lumbar lordosis angle, compared to transforaminal lumbar interbody fusion, although not statistically significant (23). CBT screws have also demonstrated similar fusion rates as conventional PSs for short-segment lumbar fusion (24). Recently, CBT screws have been considered to have the potential to be a good anchor for a long segment fusion construct in terms of pull-out strength. Matsukawa et al. have reported that CBT screws have the potential to align harmoniously with sacral-alar-iliac screws due to their medial starting point, which allows for rapid rod insertion (25). However, caution should be exercised when using CBT in cases where severe deformity correction is required in addition to long segment spinal fusion. Regarding the biomechanical behavior of CBT screws within a fusion construct, Perez-Orribo et al. reported that a conventional PS was stiffer during axial rotation in intact discs compared to original CBT screws (26). Although the long CBT is different from the original CBT, we believe that further analysis with long term follow-up is needed for the use of CBT as a long segment fusion construct.
Notably, this study presented results involving three surgeons who were not experienced in the CBT technique before using a patient-specific template guide. The results of this study revealed that this patient-specific template guide is useful for safely inserting a long CBT screw into the anterolateral part of the vertebral body, even for surgeons who are not experienced in CBT. Dayani et al. reported a learning curve for CBT screw insertion using only intraoperative radiographs and found that infection, cerebrospinal fluid leakage, and outward deviation of the screw all occurred in early experience, although there were no significant differences (27). Dabbous et al. found that the CBT technique offers fixation strength similar to the conventional PS technique. Nonetheless, risks such as pedicle fracture and incorrect screw positioning were notable during the initial learning phase of this new approach (28). In this study, no serious neurovascular complications were observed from the beginning to the end of the study, and there was no significant difference in the number of perforated CBT screws between the first 50 cases and last 50 cases (15 vs. 15). The learning curve of this patient-specific template guide is relatively quick.
This study has several limitations. First, this study involved only three surgeons who were not experienced in the CBT technique and this study did not involve surgeons who did not use a patient-specific template guide. Thus, we did not compare the accuracy of CBT with and without the use of the template guide. Second, the study did not examine clinical data and outcomes such as operative time, fixation failure, intraoperative bleeding, and rates of successful fusion. Third, we did not estimate radiation exposure doses. In this study, only lateral fluoroscopy was used because all cases were performed using a patient-specific guide. Without a guide, frontal fluoroscopy is necessary, but with a patient-specific guide the horizontal deviation can be determined to some extent by the fitting of the guide, and if drilling is performed accurately, the operation can be performed without fluoroscopy afterward. Therefore, radiation exposure is likely to be lower when CBT is inserted using this patient-specific guide compared to the conventional method. A study involving multiple centers, with a greater patient cohort and extended follow-up, is necessary to confirm our results.
Conclusions
We focused on the clinical accuracy of a patient-specific template guide for CBT placement. This technique is simple, highly accurate, and reliable. The use of patient-specific template guide could offer a viable method for ensuring precise placement of long CBT screws and decreasing complications, potentially even for surgeons who are less experienced with the CBT technique.
Acknowledgments
We would like to thank Miss Reina Akiniwa of Hokkaido Memorial Hospital for assistance with the data collection.
Funding: None.
Footnote
Reporting Checklist: The authors have completed the STROBE reporting checklist. Available at https://jss.amegroups.com/article/view/10.21037/jss-23-122/rc
Data Sharing Statement: Available at https://jss.amegroups.com/article/view/10.21037/jss-23-122/dss
Peer Review File: Available at https://jss.amegroups.com/article/view/10.21037/jss-23-122/prf
Conflicts of Interest: All authors have completed the ICMJE uniform disclosure form (available at https://jss.amegroups.com/article/view/10.21037/jss-23-122/coif). I.O. received consulting fees and speaker honoraria from MEDACTA International. The other authors have no conflicts of interest to declare.
Ethical Statement: The authors are accountable for all aspects of the work in ensuring that questions related to the accuracy or integrity of any part of the work are appropriately investigated and resolved. The study was conducted in accordance with the Declaration of Helsinki (revised in 2013). Ethical approval was granted by Hokkaido Orthopedic Memorial Hospital (No. 106). The requirement for patient informed consent was waived, owing to the retrospective nature of the study and its deidentified data.
Open Access Statement: This is an Open Access article distributed in accordance with the Creative Commons Attribution-NonCommercial-NoDerivs 4.0 International License (CC BY-NC-ND 4.0), which permits the non-commercial replication and distribution of the article with the strict proviso that no changes or edits are made and the original work is properly cited (including links to both the formal publication through the relevant DOI and the license). See: https://creativecommons.org/licenses/by-nc-nd/4.0/.
References
- Santoni BG, Hynes RA, McGilvray KC, et al. Cortical bone trajectory for lumbar pedicle screws. Spine J 2009;9:366-73. [Crossref] [PubMed]
- Matsukawa K, Yato Y, Imabayashi H, et al. Biomechanical evaluation of fixation strength among different sizes of pedicle screws using the cortical bone trajectory: what is the ideal screw size for optimal fixation? Acta Neurochir (Wien) 2016;158:465-71. [Crossref] [PubMed]
- Calvert GC, Lawrence BD, Abtahi AM, et al. Cortical screws used to rescue failed lumbar pedicle screw construct: a biomechanical analysis. J Neurosurg Spine 2015;22:166-72. [Crossref] [PubMed]
- Mobbs RJ. The "medio-latero-superior trajectory technique": an alternative cortical trajectory for pedicle fixation. Orthop Surg 2013;5:56-9. [Crossref] [PubMed]
- Sansur CA, Caffes NM, Ibrahimi DM, et al. Biomechanical fixation properties of cortical versus transpedicular screws in the osteoporotic lumbar spine: an in vitro human cadaveric model. J Neurosurg Spine 2016;25:467-76. [Crossref] [PubMed]
- Ueno M, Sakai R, Tanaka K, et al. Should we use cortical bone screws for cortical bone trajectory? J Neurosurg Spine 2015;22:416-21. [Crossref] [PubMed]
- Matsukawa K, Taguchi E, Yato Y, et al. Evaluation of the Fixation Strength of Pedicle Screws Using Cortical Bone Trajectory: What Is the Ideal Trajectory for Optimal Fixation? Spine (Phila Pa 1976) 2015;40:E873-8. [Crossref] [PubMed]
- Lee GW, Son JH, Ahn MW, et al. The comparison of pedicle screw and cortical screw in posterior lumbar interbody fusion: a prospective randomized noninferiority trial. Spine J 2015;15:1519-26. [Crossref] [PubMed]
- Takenaka S, Mukai Y, Tateishi K, et al. Clinical Outcomes After Posterior Lumbar Interbody Fusion: Comparison of Cortical Bone Trajectory and Conventional Pedicle Screw Insertion. Clin Spine Surg 2017;30:E1411-8. [Crossref] [PubMed]
- Hung CW, Wu MF, Hong RT, et al. Comparison of multifidus muscle atrophy after posterior lumbar interbody fusion with conventional and cortical bone trajectory. Clin Neurol Neurosurg 2016;145:41-5. [Crossref] [PubMed]
- Fujiwara S, Ohnishi Y, Iwatsuki K, et al. Cortical bone trajectory fixation cause low compression force in anterior vertebral column. N Am Spine Soc J 2022;10:100113. [Crossref] [PubMed]
- Sakaura H, Miwa T, Yamashita T, et al. Posterior lumbar interbody fusion with cortical bone trajectory screw fixation versus posterior lumbar interbody fusion using traditional pedicle screw fixation for degenerative lumbar spondylolisthesis: a comparative study. J Neurosurg Spine 2016;25:591-5. [Crossref] [PubMed]
- Sakaura H, Miwa T, Yamashita T, et al. Cortical bone trajectory screw fixation versus traditional pedicle screw fixation for 2-level posterior lumbar interbody fusion: comparison of surgical outcomes for 2-level degenerative lumbar spondylolisthesis. J Neurosurg Spine 2018;28:57-62. [Crossref] [PubMed]
- McKinley TO, McLain RF, Yerby SA, et al. Characteristics of pedicle screw loading. Effect of surgical technique on intravertebral and intrapedicular bending moments. Spine (Phila Pa 1976) 1999;24:18-24, discussion 25. [Crossref] [PubMed]
- Matsukawa K, Yanai Y, Fujiyoshi K, et al. Depth of vertebral screw insertion using a cortical bone trajectory technique in lumbar spinal fusion: radiological significance of a long cortical bone trajectory. J Neurosurg Spine 2021;35:601-6. [Crossref] [PubMed]
- Yanai Y, Matsukawa K, Kato T, et al. Factors important in bone union after posterior lumbar interbody fusion using the cortical bone trajectory technique. J Spine Surg 2020;6:713-20. [Crossref] [PubMed]
- Matsukawa K, Kaito T, Abe Y. Accuracy of cortical bone trajectory screw placement using patient-specific template guide system. Neurosurg Rev 2020;43:1135-42. [Crossref] [PubMed]
- Kaito T, Matsukawa K, Abe Y, et al. Cortical pedicle screw placement in lumbar spinal surgery with a patient-matched targeting guide: A cadaveric study. J Orthop Sci 2018;23:865-9. [Crossref] [PubMed]
- Lamartina C, Cecchinato R, Fekete Z, et al. Pedicle screw placement accuracy in thoracic and lumbar spinal surgery with a patient-matched targeting guide: a cadaveric study. Eur Spine J 2015;24:937-41. [Crossref] [PubMed]
- Marengo N, Matsukawa K, Monticelli M, et al. Cortical Bone Trajectory Screw Placement Accuracy with a Patient-Matched 3-Dimensional Printed Guide in Lumbar Spinal Surgery: A Clinical Study. World Neurosurg 2019;130:e98-e104. [Crossref] [PubMed]
- Li Y, Chen L, Liu Y, et al. Accuracy and safety of robot-assisted cortical bone trajectory screw placement: a comparison of robot-assisted technique with fluoroscopy-assisted approach. BMC Musculoskelet Disord 2022;23:328. [Crossref] [PubMed]
- Petrone S, Marengo N, Ajello M, et al. Cortical bone trajectory technique's outcomes and procedures for posterior lumbar fusion: A retrospective study. J Clin Neurosci 2020;76:25-30. [Crossref] [PubMed]
- Elmekaty M, Kotani Y, Mehy EE, et al. Clinical and Radiological Comparison between Three Different Minimally Invasive Surgical Fusion Techniques for Single-Level Lumbar Isthmic and Degenerative Spondylolisthesis: Minimally Invasive Surgical Posterolateral Fusion versus Minimally Invasive Surgical Transforaminal Lumbar Interbody Fusion versus Midline Lumbar Fusion. Asian Spine J 2018;12:870-9. [Crossref] [PubMed]
- Wang J, He X, Sun T. Comparative clinical efficacy and safety of cortical bone trajectory screw fixation and traditional pedicle screw fixation in posterior lumbar fusion: a systematic review and meta-analysis. Eur Spine J 2019;28:1678-89. [Crossref] [PubMed]
- Matsukawa K, Kato T, Mobbs R, et al. Combination of sacral-alar-iliac screw and cortical bone trajectory screw techniques for lumbosacral fixation: technical note. J Neurosurg Spine 2020;33:186-91. [Crossref] [PubMed]
- Perez-Orribo L, Kalb S, Reyes PM, et al. Biomechanics of lumbar cortical screw-rod fixation versus pedicle screw-rod fixation with and without interbody support. Spine (Phila Pa 1976) 2013;38:635-41. [Crossref] [PubMed]
- Dayani F, Chen YR, Johnson E, et al. Minimally invasive lumbar pedicle screw fixation using cortical bone trajectory - Screw accuracy, complications, and learning curve in 100 screw placements. J Clin Neurosci 2019;61:106-11. [Crossref] [PubMed]
- Dabbous B, Brown D, Tsitlakidis A, et al. Clinical outcomes during the learning curve of MIDline Lumbar Fusion (MIDLF®) using the cortical bone trajectory. Acta Neurochir (Wien) 2016;158:1413-20. [Crossref] [PubMed]