A comparative cadaveric biomechanical study of bilateral FacetFuse® transfacet pedicle screws versus bilateral or unilateral pedicle screw-rod construct
Highlight box
Key findings
• Bilateral FacetFuse® transfacet pedicle screws (TFPS) demonstrated superior immediate stability compared to unilateral pedicle screw-rod (PSR).
• Bilateral TFPS was equivalent in immediate stability to bilateral PSR constructs at the L5–S1 disc level.
• Bilateral TFPS could potentially reduce the number of pedicle screws needed.
What is known and what is new?
• Traditional lumbar fusion surgeries use four pedicle screws to achieve stability at the L5–S1 level.
• Biomechanical stability of bilateral TFPS and its comparison with equivalent-sized unilateral or bilateral fully threaded PSR constructs, showed that TFPS may offer comparable immediate stability.
What is the implication, and what should change now?
• Bilateral TFPS was comparable to traditional bilateral PSR constructs in lumbar fusion surgeries due to improved stability and potential clinical benefits.
• Further clinical trials are needed to confirm these results and to determine the long-term outcomes and advantages of using TFPS.
Introduction
Anatomical structures such as pedicles, facets, laminae, and spinous processes have been utilized to enhance posterior stability in order to improve fusion rates (1-6). The transfacet technique was introduced by Dr. King in 1948 by placing short screws through the lateral articulations for internal fixation (7). Over time, the pedicle screw-rod (PSR) construct became the predominant method of posterior fixation in spine fusion, as it was believed to offer improved biomechanical stability by crossing all three columns of the spine (8). Facet screw fixation is used in minimally invasive and less exposure spine surgery (LESSTM) techniques that aim to preserve anatomical structures and minimize tissue disruption during lumbar surgeries.
Biomechanical studies have shown that facet screw fixation can provide comparable stability to pedicle screws (9-11). The transfacet pedicle screw (TFPS) technique, developed in 1959 and standardized by Boucher, allows for the use of longer screws for three-column fixation, potentially increasing stability compared to traditional PSR fixation (12). Panjabi et al. demonstrated stability in axial rotation (AR) but relatively low stability in other directions when comparing different pedicle fixation systems (13). Kretzer et al. found no statistically significant differences in stability between bilateral facet screws and bilateral pedicle screws at specific lumbar levels (14). Chin et al. showed that TFPS provides similar stability to PSR at the L1–2 and L2–3 levels, but PSR demonstrated less susceptibility to loosening versus TFPS (15).
While the middle to lower lumbar spine is often considered suitable for TFPS fixation, the lumbosacral junction (L5–S1) is not commonly considered due to the high stresses experienced at this level (16,17). Nevertheless, the large and flat facet joints, as well as the wide pedicles at L5 and S1, make it a surgically accessible target, warranting further study.
The objective of this study was to investigate the biomechanical stability of bilateral TFPS in comparison to equivalent-sized unilateral or bilateral PSR constructs at the L5–S1 disc level. The study evaluates the immediate stability achieved by these constructs for achieving fusion of the lumbar segment. We hypothesized that bilateral TFPS will yield immediate lumbar fixation that is comparable to unilateral or bilateral PSR constructs.
Methods
The study was conducted in accordance with the Declaration of Helsinki (as revised in 2013). Protocol for spine-related research on human subjects has been approved by Western Institutional Review Board (WIRB®) now known as WIRB-Copernicus Group (WCG® IRB) through protocol WIRB # 20181251. The study was conducted at the Barrow Neurological Institute in Phoenix Arizona and no ethical approval or informed consent were deemed necessary given the cadaveric nature of the study as referenced previously (18). Cadavers are systematically procured from non-transplant donation companies who as part of their procurement process obtained authorization by the individual or their next of kin (19).
Specimen preparation
Fourteen lumbar spine segments from T12 to the coccyx obtained from fresh human cadavers were included in the study. Dual-energy X-ray absorptiometry (DEXA) scans were performed on the L4 vertebra of each specimen to assess bone mineral density (BMD) and ensure non-osteoporotic conditions (Table 1). The specimens were meticulously cleaned of muscle tissue while preserving ligaments, joint capsules, and discs. Keeping the discs intact reduced variables and allowed for better assessment of fixation durability in the long term. For testing, the sacrum was reinforced with household wood screws, embedded in a block of polymethylmethacrylate or fast-curing resin (Smooth-Cast 300Q, Smooth-On, Inc., Easton, PA, USA), and connected to the base of the testing apparatus (Figure 1). Similarly, the T12 vertebra was embedded after inserting reinforcing screws into a cylindrical metal fixture for load application. The spines were initially tested in their intact condition, and then surgical procedures were performed at the L5–S1 level. The specimens were divided into two groups: the TFPS (n=7) group (FacetFuse®, LESSpine, Burlington, MA, USA), and the PSRs (n=7) group (PedFuse Return, LESSpine, Burlington, MA, USA). In both groups, the screw size was standardized at 5.0 mm × 40 mm to eliminate bias from screw length and diameter. The TFPS used in this study incorporated a swiveling head washer with a footprint diameter of 12 mm and a beveled face, allowing for even load distribution around the lamina and articular process (Figure 2). In the PSR group, the pedicle screws were fully threaded and top-loading with variable angle heads and secured using locking cap with a 55-mm interconnecting rod. Fluoroscopic guidance was used for screw placement and to confirm satisfactory position (Figure 3). The testing protocol resulted in three groups for analysis and comparisons: (I) bilateral TFPS with two screws; (II) bilateral pedicle screw-rod (BPSR) using 4 screws and 2 rods; and (III) unilateral pedicle screw-rod (UPSR) using 2 screws and 1 rod.
Table 1
Variables | TFPS group (N=7) | PSR group (N=7) |
---|---|---|
Age (years), mean [SD] | 46.71 [11.37] | 59.57 [6] |
Sex, n (%) | ||
Female | 4 (28.57) | 6 (42.86) |
Male | 3 (21.43) | 1 (7.14) |
DEXA (BMD g/cm2), mean (SD) | 0.83 (0.18) | 0.89 (0.19) |
BMD, bone mineral density; TFPS, transfacet pedicle screw; PSR, pedicle screw-rod; SD, standard deviation; DEXA, dual energy X-ray absorptiometry.
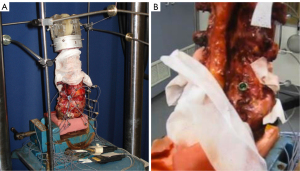
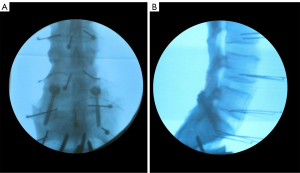
Biomechanical testing
In all tested conditions, specimens underwent standard pure moment flexibility tests to assess their motion. The motion data for the L5–S1 segment was evaluated. The tests were conducted using an apparatus that utilized a system of cables and pulleys to apply nondestructive and non-constraining torques. This apparatus was combined with a standard servohydraulic test system (MTS, Minneapolis, MN, USA) (20). The applied loading evenly distributed the loads to each motion segment, irrespective of the distance from the loading point (21). The loads were applied around the appropriate anatomical axes to induce three types of motion: flexion-extension (FE), right and left lateral bending (LB), and right and left AR. Prior to data collection, three preconditioning cycles were performed at 7.5 Nm for 60 seconds each. Following preconditioning, the specimens were allowed to rest at zero load for 60 seconds to account for creep (22-24). This preconditioning protocol ensured proper settling at the hardware-bone interface and improved the reproducibility of the results. During the data collection cycle, the load was applied quasi-statically in increments of 1.5 Nm. Each incremental load was held for 45 seconds, up to a maximum of 7.5 Nm. Range of motion (ROM) was measured as the angle achieved under maximum load (7.5 Nm) in each plane. The transition point from the lax zone (LZ) to the stiff zone (SZ), known as the “elbow” of the load-deformation response, was determined by extrapolating the quasi-static moment-angle data points at 4.5, 6.0, and 7.5 Nm to zero load using the method of least squares. Three parameters were derived from the angular quasi-static load-deformation data: ROM, LZ (representing ligamentous laxity), and SZ (representing ligamentous stretching) (25). To capture the three-dimensional motion of the specimens in response to the applied loads during flexibility tests, the Optotrak 3020 system (Northern Digital, Waterloo, Ontario, Canada) was utilized. This system used stereophotogrammetry to measure the three-dimensional displacement of infrared-emitting markers attached rigidly in a non-collinear arrangement to each vertebra. Custom software converted the marker coordinates into angles along each anatomical axis, based on the motion segment’s own coordinate system (20,26). Spinal angles were calculated using a vector technique specifically designed for describing 3D spinal motion (26).
Statistical analysis
From the collected raw data, three parameters were derived from the quasi-static load-deformation data: angular ROM, LZ, and SZ. The LZ and SZ are integral components of the ROM and represent the low-stiffness and high-stiffness sections of the biphasic load-deformation curve typically observed (25). To account for inter-specimen variability, the raw motion values for the instrumented conditions were normalized by dividing them with the motion observed in the respective intact conditions. The mean normalized values of LZ, SZ, and ROM were statistically analyzed for flexion, extension, LB (average of right and left), and AR (average of right and left). One-way analysis of variance (ANOVA) was conducted, followed by Holm-Sidak pairwise tests, to determine if there were any significant differences among the means obtained in the TFPS and PSR constructs. A P<0.05 was considered statistically significant.
In addition to the biomechanical assessments, the specimens were disarticulated and examined anatomically after completing the tests. This examination aimed to confirm if the TFPS trajectories crossed the facet joint planes, if the screw trajectories remained within the pedicles, and whether any inferior facet fractures occurred.
Results
The mean age of the fourteen specimens was 53.14±10.99 years, with 4 (28.57%) men and 10 (71.43%) women. There was a significant difference in mean age between the TFPS and PSR groups (46.71±11.37 and 59.57±6 respectively, P=0.02) however both groups exhibited similar bone mineral density (TFPS group 0.83±0.18 and PSR group 0.89±0.19, P=0.57) (Table 1). Comparisons between the instrumented conditions revealed that TFPS resulted in lower mean mobility compared to BPSR in all loading directions, with extension and AR showing significant differences (Table 2, P=0.048 and P=0.04 respectively). TFPS showed significantly restricted ROM compared to UPSR in all loading directions (Table 2, P<0.001). The difference between UPSR and BPSR was significant during LB and AR (Table 2, P=0.003 and P=0.002).
Table 2
Loading mode and comparison | P values | ||
---|---|---|---|
LZ | SZ | ROM | |
Flexion | |||
UPSR vs. BPSR | 0.14 | 0.06 | 0.08 |
UPSR vs. TFPS | 0.01* | <0.001* | 0.001* |
TFPS vs. BPSR | 0.16 | 0.050 | 0.06 |
Extension | |||
UPSR vs. BPSR | 0.15 | 0.13 | 0.054 |
UPSR vs. TFPS | 0.01* | 0.006* | <0.001* |
TFPS vs. BPSR | 0.16 | 0.11 | 0.048* |
Lateral bending | |||
UPSR vs. BPSR | 0.002* | 0.03* | 0.003* |
UPSR vs. TFPS | <0.001* | <0.001* | <0.001* |
TFPS vs. BPSR | 0.24 | 0.02* | 0.052 |
Axial rotation | |||
UPSR vs. BPSR | 0.02* | 0.002* | 0.002* |
UPSR vs. TFPS | 0.005* | <0.001* | <0.001* |
TFPS vs. BPSR | 0.44 | 0.003* | 0.04* |
*, P<0.05 is considered statistically significant. The authors own the copyright of this table (11). No permission file is required to reuse this table. ANOVA, analysis of variance; LZ, lax zone; SZ, stiff zone; ROM, range of movement; UPSR, unilateral pedicle screw-rod; BPSR, bilateral pedicle screw-rod; TFPS, transfacet pedicle screw.
Measurements using digital handheld calipers revealed that the mean distance from the caudal edge of each L5 facet articulation to the closest edge of the respective transfacet pedicle screw washer was 6.8±2.7 mm (range, 2.5 to 9.9 mm). The TFPS correctly engaged both right and left facet joints in all specimens except for one case where only one side was engaged. Out of fourteen TFPS placed, there were no inferior facet fractures, however there was a medial canal breach with one screw. None of standard pedicle screws breached the L5 or S1 pedicle walls.
Discussion
Our study showed that bilateral TFPS exhibited comparable immediate fixation to BPSR constructs at the L5–S1 disc level. Furthermore, TFPS demonstrated superior fixation when compared to UPSR constructs, suggesting a potential advantage for TFPS in spinal fusion stability. The facet joints play a crucial role in providing contact points between adjacent vertebrae for spinal stability (27). Techniques that promote facet fusion have shown success in fusion rates and clinical outcomes (28,29).
The study findings have important potential clinical relevance regarding the comparisons between the TFPS and PSR techniques. We expect greater caudal compression across the disc space by TFPS due to a downward screw angle directly across the facet joint with a lag screw in TFPS, with the potential benefit of improving lordosis and warrants future study. In contrast, PSR constructs indirectly fixate the facets and may pose a risk of reducing lordosis compared to TFPS. From a clinical standpoint, TFPS has the potential to improve sagittal balance which is beneficial in achieving optimal spinal alignment and addressing related deformities. Furthermore, the utilization of bilateral TFPS may reduce the number of pedicle screws required, while still achieving comparable stability in lumbar fusion procedures. This aspect not only has cost implications but also potentially decreases the risk of complications associated with a higher number of screws. The TFPS technique requires a single small midline incision to place multiple screws, which remains medial to the facets. In contrast, PSR sits within the multifidus muscle and can cause irritation, potentially leading to additional interventions. TFPS avoids direct contact with the adjacent facets, while PSR techniques may risk violating the adjacent facets especially in minimally invasive procedures (30). This is a critical consideration since such facet violations have been implicated in the onset of adjacent segment disease (ASD) (31,32). In clinical cases requiring intervention for ASD treatment, unlike PSR constructs, TFPS allows for extension of the fusion without the need for disrupting or modifying the intact hardware such as rod removal and replacement or placing a rod connector. This inherent flexibility of TFPS can be vital in preserving patient outcomes and minimizing additional procedure complexities.
This is the first study to biomechanically contrast TFPS and PSR fixation using equivalent-sized screws across the L5–S1 motion segment with an intact disc. A previous biomechanical study reported similar fixation performance in FE for TFPS compared to BPSR at the L4–L5 level but BPSR was stiffer in LB and AR (8). However, the study did not employ screws of equivalent size, which suggest that the comparative advantage of TFPS might be even more significant than previously reported. We postulate that LB was more challenging for TFPS compared to BPSR fixation due to the medial position of TFPS screws near the axis of rotation in the coronal plane. Pedicle screws, on the other hand, have screw heads placed farther laterally, providing a better lever arm against LB.
However, the exclusive use of 5.0 mm screws for both TFPS and PSR in this study could be seen as a limitation since it may not represent the larger sizes of pedicle screws available in clinical practice, potentially affecting the application of the results.
Conclusions
This study highlights the comparable immediate stability provided by bilateral TFPS and equivalent-sized bilateral PSR constructs at the L5–S1 level, and distinct advantage over unilateral PSR constructs. TFPS could have significant clinical implications in lumbar fusion surgeries as an alternative for achieving stability and reducing the risk of complications in PSR lumbar fusion surgeries. However, further clinical investigations are necessary to validate these findings and ascertain the long-term outcomes and advantages associated with the use of bilateral TFPS as a substitute construct. Continued research in this area will contribute to a better understanding of the clinical benefits and optimal application of TFPS, ultimately enhancing surgical outcomes in lumbar fusion surgeries.
Acknowledgments
Preliminary results of this work were presented at the University of the West Indies (UWI) Mona Medical Journal (WIMJ) as part of a spine seminar on less exposure surgery (LES) focus on spine for local educational purposes.
Funding: None.
Footnote
Data Sharing Statement: Available at https://jss.amegroups.com/article/view/10.21037/jss-24-10/dss
Peer Review File: Available at https://jss.amegroups.com/article/view/10.21037/jss-24-10/prf
Conflicts of Interest: All authors have completed the ICMJE uniform disclosure form (available at https://jss.amegroups.com/article/view/10.21037/jss-24-10/coif). K.R.C. reports that he is the cofounder and CEO of KIC (Kingsley Investment Company) Ventures and has ownership shares in the company. V.L. is an employee of LESSpine, a for-profit medical device company. The other authors have no conflicts of interest to declare.
Ethical Statement: The authors are accountable for all aspects of the work in ensuring that questions related to the accuracy or integrity of any part of the work are appropriately investigated and resolved. The study was conducted in accordance with the Declaration of Helsinki (as revised in 2013). Protocol for spine-related research on human subjects has been approved by Western Institutional Review Board (WIRB®) now known as WIRB-Copernicus Group (WCG® IRB) through protocol WIRB # 20181251. The study was conducted at the Barrow Neurological Institute in Phoenix Arizona and no ethical approval or informed consent was deemed necessary given the cadaveric nature of the study. Cadavers are systematically procured from non-transplant donation companies who as part of their procurement process obtained authorization by the individual or their next of kin.
Open Access Statement: This is an Open Access article distributed in accordance with the Creative Commons Attribution-NonCommercial-NoDerivs 4.0 International License (CC BY-NC-ND 4.0), which permits the non-commercial replication and distribution of the article with the strict proviso that no changes or edits are made and the original work is properly cited (including links to both the formal publication through the relevant DOI and the license). See: https://creativecommons.org/licenses/by-nc-nd/4.0/.
References
- Zindrick MR. The role of transpedicular fixation systems for stabilization of the lumbar spine. Orthop Clin North Am 1991;22:333-44.
- Highsmith JM, Tumialán LM, Rodts GE Jr. Flexible rods and the case for dynamic stabilization. Neurosurg Focus 2007;22:E11.
- Bono CM, Kadaba M, Vaccaro AR. Posterior pedicle fixation-based dynamic stabilization devices for the treatment of degenerative diseases of the lumbar spine. J Spinal Disord Tech 2009;22:376-83.
- Vaccaro AR, Garfin SR. Internal fixation (pedicle screw fixation) for fusions of the lumbar spine. Spine (Phila Pa 1976) 1995;20:157S-65S.
- El Masry MA, McAllen CJ, Weatherley CR. Lumbosacral fusion using the Boucher technique in combination with a posterolateral bone graft. Eur Spine J 2003;12:408-12.
- Hida S, Naito M, Arimizu J, et al. The transverse placement laminoplasty using titanium miniplates for the reconstruction of the laminae in thoracic and lumbar lesion. Eur Spine J 2006;15:1292-7.
- King D. Internal fixation for lumbosacral fusion. J Bone Joint Surg Am 1948;30A:560-5.
- Agarwala A, Bucklen B, Muzumdar A, et al. Do facet screws provide the required stability in lumbar fixation? A biomechanical comparison of the Boucher technique and pedicular fixation in primary and circumferential fusions. Clin Biomech (Bristol, Avon) 2012;27:64-70.
- Burton D, McIff T, Fox T, et al. Biomechanical analysis of posterior fixation techniques in a 360 degrees arthrodesis model. Spine (Phila Pa 1976) 2005;30:2765-71.
- Harris BM, Hilibrand AS, Savas PE, et al. Transforaminal lumbar interbody fusion: the effect of various instrumentation techniques on the flexibility of the lumbar spine. Spine (Phila Pa 1976) 2004;29:E65-70.
- Chin KR, Pencle FJR, Newcomb AGU, et al. Biomechanical Comparison of Same Size Transfacet Screws Versus Pedicle Screws Across the L5-S1 Native Disc. West Indian Med J 2017;66:416-23.
- Boucher HH. A method of spinal fusion. J Bone Joint Surg Br 1959;41-B:248-59.
- Panjabi MM, Yamamoto I, Oxland TR, et al. Biomechanical stability of five pedicle screw fixation systems in a human lumbar spine instability model. Clin Biomech (Bristol, Avon) 1991;6:197-205.
- Kretzer RM, Molina C, Hu N, et al. A Comparative Biomechanical Analysis of Stand Alone Versus Facet Screw and Pedicle Screw Augmented Lateral Interbody Arthrodesis: An In Vitro Human Cadaveric Model. Clin Spine Surg 2016;29:E336-43.
- Chin KR, Newcomb AG, Reis MT, et al. Biomechanics of posterior instrumentation in L1-L3 lateral interbody fusion: Pedicle screw rod construct vs. transfacet pedicle screws. Clin Biomech (Bristol, Avon) 2016;31:59-64.
- Rhee JW, Petteys RJ, Anaizi AN, et al. Prospective evaluation of 1-year outcomes in single-level percutaneous lumbar transfacet screw fixation in the lateral decubitus position following lateral transpsoas interbody fusion. Eur Spine J 2015;24:2546-54.
- Chin KR, Seale J, Cumming V. Mini-open or percutaneous bilateral lumbar transfacet pedicle screw fixation: a technical note. J Spinal Disord Tech 2015;28:61-5.
- Godzik J, Kalb S, Reis MT, et al. Biomechanical evaluation of interbody fixation with secondary augmentation: lateral lumbar interbody fusion versus posterior lumbar interbody fusion. J Spine Surg 2018;4:180-6.
- Meaningful Donation. Register 2023. Available online: https://meaningfuldonationcremation.com/register/
- Crawford NR, Dickman CA. Construction of local vertebral coordinate systems using a digitizing probe. Technical note. Spine (Phila Pa 1976) 1997;22:559-63.
- Panjabi MM. Biomechanical evaluation of spinal fixation devices: I. A conceptual framework. Spine (Phila Pa 1976) 1988;13:1129-34.
- Yücesoy K, Yüksel KZ, Baek S, et al. Biomechanics of unilateral compared with bilateral lumbar pedicle screw fixation for stabilization of unilateral vertebral disease. J Neurosurg Spine 2008;8:44-51.
- Karahalios DG, Kaibara T, Porter RW, et al. Biomechanics of a lumbar interspinous anchor with anterior lumbar interbody fusion. J Neurosurg Spine 2010;12:372-80.
- Perez-Orribo L, Kalb S, Reyes PM, et al. Biomechanics of lumbar cortical screw-rod fixation versus pedicle screw-rod fixation with and without interbody support. Spine (Phila Pa 1976) 2013;38:635-41.
- Crawford NR, Peles JD, Dickman CA. The spinal lax zone and neutral zone: measurement techniques and parameter comparisons. J Spinal Disord 1998;11:416-29.
- Crawford NR, Yamaguchi GT, Dickman CA. A new technique for determining 3-D joint angles: the tilt/twist method. Clin Biomech (Bristol, Avon) 1999;14:153-65.
- Berven S, Tay BB, Colman W, et al. The lumbar zygapophyseal (facet) joints: a role in the pathogenesis of spinal pain syndromes and degenerative spondylolisthesis. Semin Neurol 2002;22:187-96.
- Li Z, Li Z, Chen X, et al. Comparison between modified facet joint fusion and posterolateral fusion for the treatment of lumbar degenerative diseases: a retrospective study. BMC Surg 2022;22:29.
- Miyashita T, Kato K, Yunde A, et al. Comparison of facet fusion rates and clinical outcomes between cortical bone trajectory screw and percutaneous pedicle screw fixation for degenerative lumbar spondylolisthesis. Spine J 2024;24:1202-10.
- Herren C, Reijnen M, Pishnamaz M, et al. Incidence and Risk Factors for Facet Joint Violation in Open Versus Minimally Invasive Procedures During Pedicle Screw Placement in Patients with Trauma. World Neurosurg 2018;112:e711-8.
- Babu R, Park JG, Mehta AI, et al. Comparison of superior-level facet joint violations during open and percutaneous pedicle screw placement. Neurosurgery 2012;71:962-70.
- Wangsawatwong P, de Andrada Pereira B, Lehrman JN, et al. Biomechanical Effects of Facet Joint Violation After Single-Level Lumbar Fusion With Transpedicular Screw and Rod Instrumentation. Spine (Phila Pa 1976) 2023;48:1033-40.