Minimally-invasive trans-facet lumbar interbody fusion using a dual-dimension expandable cage: preliminary results of a multi-institutional retrospective study
Highlight box
Key findings
• Minimally invasive trans-facet lumbar interbody fusion (LIF) with dual-dimension expandable cages demonstrates a substantial capacity for spondylolisthesis reduction and disc height restoration, and provides good short-term clinical outcomes.
What is known and what is new?
• The standard minimally invasive transforaminal lumbar interbody fusion (MI-TLIF) technique provides favorable clinical outcomes. However, it carries potential risks of radiculitis and incidental durotomy.
• The trans-facet modification of the standard MI-TLIF reduces the risk of neural injury by preserving the bones and ligamentum flavum as protective barriers. It also allows for thorough decompression, leading to good clinical outcomes.
What is the implication, and what should change now?
• The minimally invasive trans-facet LIF demonstrates good clinical and radiographic outcomes in the short term. This approach may be the most appropriate for deploying a large cage as it allows for a safe, large, unobstructed pathway to the disc.
Introduction
Minimally invasive transforaminal lumbar interbody fusion (MI-TLIF) has grown in prominence, with an extensive body of literature showing its favorable clinical outcomes over open surgeries (1-3). However, the technique is not devoid of challenges. There are potential risks of radiculitis (ranging from 2.8% to 57.1%), screw malposition (0.3–12.7%), and incidental durotomy (0.3–8.6%) (4,5).
The trans-facet lumbar interbody fusion (trans-facet LIF) was introduced recently to maintain good patient outcomes while reducing surgical risks (6). The technique involves engaging the disc via drilling through both the superior and inferior articular processes. By leaving the lateral edge of the superior articular process, spinal lamina, pars interarticularis, and ligamentum flavum unremoved as protective barriers, the annulotomy site is safer from exiting and traversing nerve roots. Thus, the interbody fusion procedure can be safely completed. Additionally, there is an opportunity for direct decompression after discectomy and interbody fusion, depending on individual clinical conditions. Khalifeh et al. (7) reported their case series comprising 68 patients. They observed improved patient-reported and radiographic outcomes, including spondylolisthesis reduction and increased disc height, foraminal height, and segmental lordosis.
Restoring proper lumbar lordosis according to individual pelvic incidence (PI) is crucial, as it is associated with good clinical outcomes (8-10). Compared to traditional static cages, expandable cages lead to a greater and more sustained increase in disc height and segmental lordosis, which is associated with improved patient outcomes (11). Further, a significant increase in lumbar lordosis has been reported when applying expandable cages in two-level MI-TLIF (12). Most of these cages expand in the superior-inferior planes (one dimension). Due to the small size of the safe triangle and the limits of neural retraction, the mediolateral dimensions of these cages are small (8–12 mm). Thus, these centrally located small cages put pressure against the relatively weak part of the endplate and increase the risk of cage subsidence (13,14). This results in less favorable clinical and radiological outcomes (15). Recently, a novel dual-dimension expandable cage has been introduced (DualX, Amplify Surgical, Irvine, CA, USA). Expanding medial-laterally increases the footprint and pushes the cage-bone contact area toward the periphery of the endplate; expanding cranial-caudally restores disc height and sagittal alignment with properly selected cage lordosis.
In this case series, we report our clinical and surgical outcomes combining these two important advancements in minimally-invasive spine surgery: (I) the use of the trans-facet trajectory to allow for a larger footprint for interbody fusion and (II) the use of a dual-dimension expandable cage. We present this article in accordance with the STROBE reporting checklist (available at https://jss.amegroups.com/article/view/10.21037/jss-24-29/rc).
Methods
Study design and participants
We respectively reviewed the medical records and radiographs for consecutive patients older than 18 years who underwent minimally-invasive trans-facet LIF with dual-dimension expandable cages. The patients were sourced from Duke University Hospital and Vail-Summit Orthopaedics and Neurosurgery and were treated between February 1, 2022 and July 15, 2023. Inclusion criteria were as follows: (I) spondylolisthesis within Meyerding grade II with segmental instability; (II) disc degeneration disease with segmental instability; (III) significant neural element compression requiring extensive decompression, with anticipated iatrogenic instability post-decompression. Patients with pronounced spinal deformities leading to sagittal imbalance or scoliosis, tumors, spine infections, trauma, or those who were lost to follow-up were excluded.
Finally, 20 patients were enrolled in this study. Nine were males and 11 were females, with a mean age of 61.2±14.3 years. All patients received subjective outcome measures, followed by standing plain radiography of the whole spine and lumbosacral spine. The clinical outcomes and radiographic measurements were compared between pre- and post-operation at three months.
The study was conducted in accordance with the Declaration of Helsinki (as revised in 2013). The study was approved by the Institutional Review Board of Duke University Hospital (approval number: Pro00100250) and individual consent for this retrospective analysis was waived. Vail-Summit Orthopaedics and Neurosurgery was also informed and agreed the study.
Clinical and radiographic outcome measures
We recorded patient demographics and surgical details such as operative time, estimated blood loss, length of hospital stay, and perioperative complications. All patients were instructed to indicate their pain in the back using a 10-point visual analogue scale (VAS). The Oswestry Disability Index (ODI) was adopted for a more comprehensive survey of clinical symptoms and physical function. Scores in each category were calculated separately and then converted to percentile. The patient-reported outcomes were collected before the operation, at a three-month postoperative follow-up, and during a phone interview one year after the operation. The minimum clinically important differences (MCID) were set at 1.2 points for back pain and 12.8 points for ODI, based on the study of Copay et al. (16).
Radiographic measurements, taken before the surgery and three months post-operation, included anterior and posterior disc space heights, segmental lordosis, and the extent of spondylolisthesis. These were measured from standing anterior-posterior (AP) and lateral views of the lumbosacral spine. Additionally, spinopelvic parameters, including lumbar lordosis, PI, and pelvic tilt (PT), were gauged from full-length standing radiographs. Cage subsidence, defined as a cage protruding more than 2 mm into the vertebral body on standing lateral radiographs, was classified into mild (2–4 mm) or severe (>4 mm) (17). Subsidence appearing in image studies taken during postoperative admission was termed “early”, while that discovered in follow-up radiographs post six-week operation was termed “late” (18). All radiographic parameters were measured by an experienced spine surgeon, and the mean of three repeated measurements was reported.
Surgical procedure
Under general or awake spinal anesthesia, patients were positioned prone on a Jackson table, arms abducted to less than 90 degrees. Electromyography (EMG) monitoring electrodes were placed on both lower extremities. A paramedian skin incision was made bilaterally, spanning between the pedicles of the target levels along the lateral pedicle line. Using the Wiltse approach, the plane between the multifidus and longissimus muscles was exposed, revealing the facet joint’s lateral aspect. Percutaneous trans-pedicle screws were placed under the guidance of the TrackX fluoroscopy-based real-time 2D instrument tracking system (TrackX Technology, Hillsborough, NC, USA) or 3D neuronavigation (StealthStation S8 Surgical Navigation System, Minneapolis, MN, USA). Then, a guide pin was inserted to the targeted facet joint with the docking point and convergence angle planned preoperatively. Serial soft tissue dilators were introduced, followed by a tubular retractor. An operative microscope aided in bony drilling with a high-speed burr. Specific bony portions were removed or retained to protect critical elements. Medially, part of the inferior articular process of the cranial vertebra was removed, leaving the spinal lamina and ligamentum flavum to protect the dura sac and transversing nerve root; laterally, the lateral part of the superior articular process of the caudal vertebra was marked and preserved as the safe barrier to the exiting nerve root. The cranial and caudal edge of the bony resection was determined by lateral fluoroscopy. The bony drilling was deepened to expose the annulus, after which the disc materials were removed. A DaulX dual-expanding cage (Amplify Surgical, Irvine, CA, USA) was placed into the disc space and then expanded to the aimed height and width (Figure 1). The height and lordosis were determined by both the preoperative imaging to achieve proper spinopelvic alignment and the intraoperative soft tissue tension. The final height could be as large as 17 mm at various degrees of lordosis, and the cage also expended laterally to maximize the footprint, with the maximum mediolateral expansion being 21 mm. Using a specially designed bone grafting delivery device, we filled the space inside and surrounding the cage with autogenous bone graft and DBX demineralized bone matrix (DePuy Synthes, Warsaw, IN, USA).
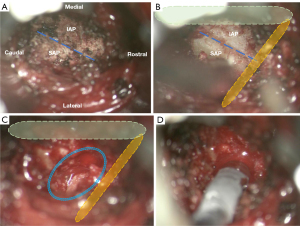
Then, the tubular retractor was moved backward and turned medially toward the spinal lamina. Decompression for the spinal canal and/or lateral recess was performed if there was evident neural compression in the preoperative imaging and the patient had corresponding symptoms. After a final EMG check and surgical wound irrigation, meticulous hemostasis was performed and the wound was closed in layers (Figure 2).
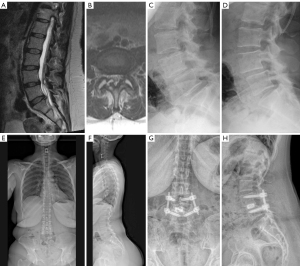
Statistical analysis
Statistical analyses were carried out using IBM SPSS Statistics for Windows, version 19.0 (IBM Corp., Armonk, NY, USA). The chi-square test was employed for categorical variables. For continuous variables, the data’s normality was checked using the Kolmogorov-Smirnov test. The Student’s t-test and Mann-Whitney U test were applied for parametric and nonparametric analyses between baseline and postoperative radiographic parameters, respectively. Repeated-measures one-way analysis of variance (ANOVA) with Bonferroni post-hoc test was performed to analyze the change in surgical outcomes. The bivariate Pearson correlation analysis followed by a multiple regression analysis was conducted to determine the factors associated with the increase in segmental lordosis post-surgery. A P value of less than 0.05 was considered significant.
Results
Patient demographics
Twenty patients, consisting of 9 males and 11 females, were included in the study. The mean age was 61.2±14.3 years, and the mean body mass index was 26.5±4.9 (Table 1). Seventeen patients (85.0%) had spondylolisthesis, with 15 patients having grade I spondylolisthesis and the remaining two patients having grade II spondylolisthesis. Two patients underwent a two-level fusion surgery, while the rest had a single-level surgery (Table 1). Therefore, a total of 22 levels were included in the analysis. L4/5 was the most frequently involved level, accounting for 68.2% of all cases, followed by L5/S1 (22.7%) and L3/4 (9.1%, Table 2). All patients underwent unilateral laminotomy for ipsilateral or bilateral decompression due to either a facet cyst or spinal canal stenosis after the trans-facet LIF procedure.
Table 1
Patient # | Age (years) | Sex | BMI (kg/m2) | Anesthesia | Pathology level | Diagnosis |
---|---|---|---|---|---|---|
1 | 70 | M | 22.4 | General | L4/5 | Recurrent left L4/5 facet cyst and spinal stenosis |
2 | 44 | M | 29.0 | General | L4/5 and L5/S1 | Grade I spondylolisthesis, L4/5; degenerated disc disease, L5/S1 |
3 | 87 | M | 31.8 | Awake | L4/5 | Grade I spondylolisthesis, L4/5; spinal stenosis, L2–L5 |
4 | 55 | F | 36.8 | Awake | L4/5 | Grade I spondylolisthesis, L4/5; facet cyst, right L4/5 |
5 | 79 | F | 37.5 | Awake | L3/4 | Grade I spondylolisthesis, L3/4; facet cyst, left L3/4 |
6 | 70 | F | 24.2 | General | L4/5 | Grade I spondylolisthesis, L4/5; spinal stenosis |
7 | 36 | M | 27.8 | General | L4/5 | Grade II spondylolisthesis, L4/5; spinal stenosis |
8 | 44 | F | 28.8 | Awake | L5/S1 | Recurrent HIVD, L5/S1 |
9 | 67 | M | 24.8 | General | L3/4 and L4/5 | Postlaminectomy kyphosis, retrolisthesis, L3/4; grade I spondylolisthesis, L4/5 |
10 | 54 | F | 19.2 | Awake | L4/5 | Grade I spondylolisthesis, L4/5; spinal stenosis |
11 | 46 | F | 26.6 | Awake | L4/5 | Grade I spondylolisthesis, L4/5; facet cyst, left L4/5 |
12 | 68 | F | 23.5 | Awake | L4/5 | Grade I spondylolisthesis, L4/5; spinal stenosis |
13 | 81 | M | 20.8 | Awake | L4/5 | Grade I spondylolisthesis, L4/5; facet cyst, right L4/5 |
14 | 62 | F | 25.0 | General | L4/5 | Grade I spondylolisthesis, L4/5; spinal stenosis |
15 | 72 | F | 24.0 | General | L4/5 | Grade II spondylolisthesis, L4/5; spinal stenosis |
16 | 65 | M | 27.6 | Awake | L4/5 | Grade I spondylolisthesis, L4/5; spinal stenosis |
17 | 56 | M | 24.4 | Awake | L5/S1 | Grade I spondylolisthesis, L5/S1; spinal stenosis |
18 | 66 | M | 29.4 | Awake | L4/5 | Grade I spondylolisthesis, L4/5; spinal stenosis |
19 | 63 | F | 25.0 | General | L5/S1 | Grade I spondylolisthesis, L5/S1; spinal stenosis |
20 | 38 | F | 20.4 | Awake | L5/S1 | Spondylolytic spondylolisthesis, L5/S1, with instability |
Total, mean (SD) | 61.2 (14.3) | – | 26.5 (4.9) | – | – | – |
BMI, body mass index; F, female; HIVD, herniated intervertebral disc; M, male; SD, standard deviation.
Table 2
Variables | Values |
---|---|
Patient | 20 (100.0) |
Age (years) | 61.2±14.3 |
Sex | |
Male | 9 |
Female | 11 |
BMI (kg/m2) | 26.5±4.9 |
Operative level | |
L3/L4 | 2 (9.1) |
L4/L5 | 15 (68.2) |
L5/S1 | 5 (22.7) |
Data are expressed as n, n (%) or mean ± standard deviation. BMI, body mass index.
Operative data
Twelve patients (60.0%) underwent awake surgery, with a mean operative time of 164.5±36.1 minutes and an estimated blood loss of 64.0±39.5 mL. The mean length of hospital stay for all patients was 1.75±1.2 days (Table 3). Two complications (10%) were recorded: an incidental durotomy occurred while trying to release the dura from adhesive scar tissue in a patient who had undergone decompression surgery twice. This happened after the placement of the cage and thus was not a complication due to cage placement. We repaired the durotomy site, and the patient experienced no neurological sequelae. One pedicle screw misplacement was identified in another patient, leading to leg pain. The screw was revised in a subsequent surgery, and the leg pain resolved. There were no instances of neural element damage, postoperative hematoma, or infection.
Table 3
Variables | Values |
---|---|
Patient | 20 (100.0) |
Awake surgery | 12 (60.0) |
Operative time† (min) | 164.5±36.1 |
Estimated blood loss† (mL) | 64.0±39.5 |
Cage lordotic angle (4/8/12 degrees) | 7 (31.8)/14 (63.6)/1 (4.5) |
Length of hospital stay (days) | 1.75±1.2 |
Complication | |
Incidental durotomy | 1 (5.0) |
Pedicle screw misplacement | 1 (5.0) |
Cage subsidence | |
Early/late subsidence, mild | 2 (10.0)/2 (10.0) |
Data are expressed as n (%) or mean ± standard deviation. †, for one-level surgery.
Four patients (20%) had cage subsidence ranging from 2 to 4 mm, categorized as mild. Radiographs taken on postoperative day 1 revealed cage subsidence in two of these patients, presumably related to intraoperative endplate injury. The other two patients showed cage subsidence at the 6-week postoperative mark, with no further sinking observed at the final follow-up (Table 3). None of the patients required a revision surgery.
Clinical outcomes and radiographic parameters
The mean back pain VAS score before surgery was 7.3±2.7, which significantly decreased to 2.9±2.0 (P<0.001) and 2.6±1.6 (P=0.02) at postoperative three months and one year, respectively. The ODI also showed significant improvement, decreasing from 48.7±22.9 pre-operation to 17.8±10.0 three months post-operation (P<0.001) and 16.4±11.1 one-year post-operation (P=0.03; Table 4). One year after surgery, 80% of patients reached the MCID in the VAS score, and 83.3% passed the MCID in the ODI score. The radiographic parameters indicated that the median spondylolisthesis slippage was initially 5.9 mm with an interquartile range (IQR) of 5.0 to 10.3 mm, and significantly reduced to 0 mm (IQR, 0–3 mm) post-surgery (P<0.001). The anterior and posterior disc heights were initially 9.8±4.7 and 4.9±3.3 mm, respectively, and they significantly increased to 15.1±2.6 and 10.5±2.2 mm post-surgery (P<0.001 for both anterior and posterior disc heights; Table 5, Figure 3).
Table 4
Surgical outcomes (n=20) | Baseline | Post-op 3m | Post-op 1y | P value† |
---|---|---|---|---|
Visual analogue scale | 7.3±2.7 | 2.9±2.0 | 2.6±1.6 | 0.01 |
Oswestry disability index | 48.7±22.9 | 17.8±10.0 | 16.4±11.1 | 0.01 |
Data are expressed as mean ± standard deviation. †, repeated-measures one-way analysis of variance. Post-op, postoperative; 3m, 3 months; 1y, 1 year.
Table 5
Radiologic parameters (n=20) | Baseline | Post-op 3m | P value |
---|---|---|---|
Spondylolisthesis (mm) | 5.9 (5.0–10.3) | 0 (0–3) | <0.001 |
Anterior disc height (mm) | 9.8±4.7 | 15.1±2.6 | <0.001 |
Posterior disc height (mm) | 4.9±3.3 | 10.5±2.2 | <0.001 |
Pelvic incidence (degree) | 57.8±9.1 | 59.7±11.6 | 0.13 |
Lumbar lordosis (degree) | 53.8±13.9 | 53.5±14.1 | 0.87 |
Pelvic tilt (degree) | 22.7±5.9 | 24.5±6.8 | 0.11 |
PI minus LL (degree) | 1.75 (−1.2 to 12.5) | 6.8 (5–12.1) | 0.14 |
Data are expressed as mean ± standard deviation or median (interquartile range). LL, lumbar lordosis; PI, pelvic incidence; Post-op, postoperative; 3m, 3 months.
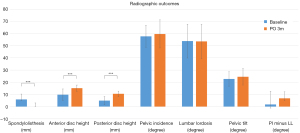
Regarding spinopelvic parameters, there were no significant changes in PI, lumbar lordosis, and PT three months post-surgery (P>0.05). Additionally, the median value of PI minus lumbar lordosis was initially 1.75 degrees (IQR, −1.2 to 12.5 degrees) and changed to 6.8 degrees (IQR, 5–12.1 degrees) three months post-surgery, showing no significant changes (P=0.14; Table 5, Figure 3).
The segmental lordosis increased by 2.9 degrees after the surgery. A bivariate correlation analysis was first applied to correlate preoperative radiographic factors and implant characteristics to the increase in segmental lordosis. Significant correlations were found between the increase of segmental lordosis and both cage height and cage lordotic angle, with correlation coefficients of 0.53 (P=0.01) and 0.51 (P=0.02), respectively (Table 6). However, a further multiple regression analysis identified only cage height as the independent factor influencing the increase of segmental lordosis, with a β coefficient of 0.53 (P=0.03; Table 6).
Table 6
Increase in segmental lordosis | Correlation coefficient† | P value† | β coefficient‡ | P value‡ |
---|---|---|---|---|
Cage height | 0.53 | 0.01 | 0.53 | 0.03 |
Cage lordotic angle | 0.51 | 0.02 | 0.31 | 0.27 |
†, bivariate Pearson correlation; ‡, multiple regression analysis.
Discussion
Key findings
In this multi-institutional study, we reported our preliminary results of minimally-invasive trans-facet LIF using a novel dual-dimension expandable cage. The combination of these two advancements was associated with good short-term clinical outcomes and a significant capacity for spondylolisthesis reduction, disc height restoration, and increase in segmental lordosis. There were no complications regarding nerve root injury or incidental durotomy associated with the trans-facet approach. The procedure provided a safe and sufficiently large corridor to the intervertebral disc and thus facilitated deploying large cages.
Strengths and limitations
The primary advantages of the trans-facet approach include minimizing neural injury risks by preserving the natural anatomical barriers and providing a significantly larger safe zone for accessing the intervertebral disc compared to the traditional TLIF and trans-Kambin approach. These features render the trans-facet TLIF the most suitable approach to implementing the newly introduced dual-dimension expandable cage, which has a width of 12 mm before medial-lateral expansion. The medial-lateral expansion mechanism enlarges the cage footprint and optimizes the ability to restore disc height safely. Nevertheless, as both the trans-facet approach and the dual-dimension expandable cages are recent advancements, and it may be risky to deploy this large cage with traditional TLIF or trans-Kambin approach, our study is limited by its relatively small sample size and the absence of a control group for surgical techniques or implant choices. This poses challenges in distinguishing the individual contributions of the trans-facet approach and the innovative implants to the favorable clinical outcomes.
Our study, which highlighted several beneficial outcomes of minimally invasive trans-facet LIF with dual-expandable cages, has limitations. First, this retrospective study originated from two experienced minimally-invasive spine surgeons, so one should exercise caution when generalizing the results. Outcomes might vary depending on a surgeon’s experience with minimally-invasive surgery, clinical judgment, and implant choices. Second, the follow-up period was relatively short, with clinical outcomes evaluated at one year and radiographic parameters measured at three months, making it challenging to determine the fusion rate. Third, compared to static cages, the expandable cages may provide greater improvement in radiographic measurements, such as anterior disc height, posterior disc height, and segmental lordosis. However, these improved radiographic outcomes do not necessarily translate to better patient-reported outcomes (19,20). Therefore, despite our report showing good clinical and radiographic outcomes, it remains inconclusive whether the added costs of dual-dimension expandable cages compared to static or single-dimension expandable cages are justified and provide sufficient benefit. Future large-scale studies with well-defined comparative groups and extended follow-ups may be necessary to thoroughly explore the benefits of the trans-facet approach and the novel dual-dimension expandable cage.
Explanations of findings
Awake spine surgery utilizes spinal, epidural, or combined anesthesia techniques, circumventing complications from general anesthesia. Numerous benefits of awake spine surgery have been established, including enhanced intraoperative hemodynamic stability, reduced intraoperative blood loss, diminished early postoperative nausea and vomiting, decreased postoperative urinary retention, lower early pain scores, and shorter hospital stays (21-24). Sixty percent of our patients underwent minimally-invasive trans-facet LIF with spinal anesthesia and erector spinae plane block. None experienced complications related to general anesthesia, such as postoperative nausea, vomiting, or cognitive dysfunction.
Complications like incidental durotomy and radiculitis are not uncommon with MI-TLIF (4,5,25). To reduce the risk of neural injury, we minimized the duration that the neural structure is exposed by using trans-facet LIF. By preserving the spinal lamina and ligamentum flavum, exposure of the dural sac and traversing nerve root is avoided during the interbody fusion procedure, which reduces the risk of injury. Additionally, the preservation of the lateral edge of the superior articular process and pars interarticularis safeguards the exiting nerve root with bone structures. Using these natural anatomical barriers, trans-facet LIF offers decreased risks of neural injury (7). In our series, no neural structure damage occurred during the fusion procedure. The only durotomy occurred during decompression after a smooth interbody fusion, arising as we attempted to separate the dura from adhesive scar tissue from a prior facet cyst resection, and thus was not considered as a complication related to cage placement. Restate, trans-facet LIF is a procedure with minimal risks of neural injury.
A sufficiently large safe corridor is pivotal for cage deployment. With the TLIF technique, the safe zone was reported to measure 1.15 cm2 at L1/L2 to 1.26 cm2 at L5/S1 (26). This space is restrictive for introducing a large cage. Efforts to enlarge this space often necessitate retracting the dural sac and traversing the root medially, potentially risking traction injury to the neural structure. Furthermore, cage introduction might lead to incidental durotomy due to proximity to medial neural structures. Some surgeons use the trans-Kambin approach to access the disc space (27). However, this anatomic corridor might be even smaller, measuring approximately 53.81 mm2 at L1/L2 to 115.84 mm2 at L4/L5 (28). Even with foraminoplasty to secure a larger safe zone, nerve root injury remains a concern due to its proximity to the exiting nerve root (29). Furthermore, pathologies like spondylolisthesis can further reduce the safe area (30). The trans-facet approach accesses the disc space through a corridor between traditional TLIF and the trans-Kambin approach, and a recent study showed that both the safe area and the maximum permissible cannula diameter for the trans-facet approach were significantly larger than the trans-Kambin approach and traditional safe triangles (31). Despite the inherent advantage, comprehensive imaging studies, meticulous preoperative planning on trajectory, and even nerve segmentation in magnetic resonance images are vital for safely performing trans-facet LIF (30).
Although there was no significant change in overall lumbar lordosis and spinopelvic parameters at the one-year follow-up, we observed a 2.9-degree increase in segmental lordosis, with 77.3% of patients having a more lordotic segmental angle postoperatively. Liu et al. documented an overall increase in segmental lordosis by 1.88 degrees with lordosing TLIFs in approximately 57% of their patients, using a mix of static and expandable cages (32). Ledesma et al. reported a more significant increase in segmental lordosis of 2.45 degrees with single-dimension expandable cages, compared to a 0.86-degree increase with static ones at the one-year follow-up (19). Meta-analysis presents varied perspectives on whether expandable cages result in a more significant increase in segmental lordosis. Alvi et al. found a significant correlation (33), while Lin et al. did not (34). Several factors, such as expandable cages, cage position, and cage lordotic angle, have been proposed to be associated with an increase in segmental lordosis (19,35,36), and in our study, we found that the increase in segmental lordosis was associated with the final height of the cage rather than its lordotic angle. Despite debates on the contributing factors of an increased segmental lordosis, the restored segmental lordosis correlates with favorable clinical outcomes (11) and is reported as a protective factor against adjacent segment degeneration (37).
While expandable cages have shown promising results, cage subsidence remains a potential complication. Introducing and expanding the cage at the center of the endplate places increased pressure on it, which might lead to endplate injury and subsequent cage subsidence. The rate of cage subsidence ranges from 5.4% to 25% with single-dimension expandable cages (19,38,39), compared to 6% to 22.4% with static cages (19,38,40). Alvi et al. performed a meta-analysis assessing the difference between single-dimension expandable and static cages and found no significant difference in subsidence rate (33). To increase the footprint and reduce the risk of cage subsidence, novel dual-dimension expandable cages were recently introduced. With medial-lateral expansion, it moves the cage-endplate interface toward a stronger periphery of the endplate (14,41,42). These cages mimic the biomechanics of anterior or lateral approaches and can be deployed with TLIF, eliminating the need for position shifts and risks associated with anterior/lateral approaches, such as injuries to visceral organs, vessels, or the lumbosacral plexus. With cranial-caudal expansion and selected lordotic angle, disc height and segmental lordosis can be restored, further providing indirect decompression for the neural elements and good spinal alignment. Although dual-dimension expandable cages potentially decrease the risk of subsidence, there are currently no reports directly comparing these cages to static or single-dimension expandable cages. Park and Heo reported no cage subsidence events in their series using the same dual-dimension expandable cage with a biportal endoscopic TLIF technique (43). Our study recorded mild early cage subsidence in two patients (10%), possibly linked to intraoperative endplate injuries. Another two patients (10%) exhibited mild/late cage subsidence. However, no progressive subsidence was noted, and overall good outcomes were reported. Careful preoperative planning and meticulous intraoperative techniques, combined with the larger footprint and optimal lordotic angle from the novel dual-dimension expandable cage, result in positive clinical outcomes.
Conclusions
Minimally-invasive trans-facet LIF with dual-dimension expandable cages demonstrates a substantial capacity for spondylolisthesis reduction and disc height restoration, and provides good short-term clinical outcomes. It may be the most appropriate for deploying this large cage as it allows for a large, unobstructed pathway to the disc. However, future studies are needed to determine the long-term outcomes, including the arthrodesis rate.
Acknowledgments
Funding: None.
Footnote
Reporting Checklist: The authors have completed the STROBE reporting checklist. Available at https://jss.amegroups.com/article/view/10.21037/jss-24-29/rc
Data Sharing Statement: Available at https://jss.amegroups.com/article/view/10.21037/jss-24-29/dss
Peer Review File: Available at https://jss.amegroups.com/article/view/10.21037/jss-24-29/prf
Conflicts of Interest: All authors have completed the ICMJE uniform disclosure form (available at https://jss.amegroups.com/article/view/10.21037/jss-24-29/coif). M.M.A.E.B. serves as a consultant for Amplify Surgical. However, the authors declared that Amplify Surgical was not involved in the study’s design, data collection, analysis, manuscript preparation, or decision to publish the findings. The company did not have access to the manuscript or the data at any time. The other authors have no conflicts of interest to declare.
Ethical Statement: The authors are accountable for all aspects of the work in ensuring that questions related to the accuracy or integrity of any part of the work are appropriately investigated and resolved. The study was conducted in accordance with the Declaration of Helsinki (as revised in 2013). The study was approved by the Institutional Review Board of Duke University Hospital (approval number: Pro00100250) and individual consent for this retrospective analysis was waived. Vail-Summit Orthopaedics and Neurosurgery was also informed and agreed the study.
Open Access Statement: This is an Open Access article distributed in accordance with the Creative Commons Attribution-NonCommercial-NoDerivs 4.0 International License (CC BY-NC-ND 4.0), which permits the non-commercial replication and distribution of the article with the strict proviso that no changes or edits are made and the original work is properly cited (including links to both the formal publication through the relevant DOI and the license). See: https://creativecommons.org/licenses/by-nc-nd/4.0/.
References
- Khan NR, Clark AJ, Lee SL, et al. Surgical Outcomes for Minimally Invasive vs Open Transforaminal Lumbar Interbody Fusion: An Updated Systematic Review and Meta-analysis. Neurosurgery 2015;77:847-74; discussion 874. [Crossref] [PubMed]
- Lu VM, Kerezoudis P, Gilder HE, et al. Minimally Invasive Surgery Versus Open Surgery Spinal Fusion for Spondylolisthesis: A Systematic Review and Meta-analysis. Spine (Phila Pa 1976) 2017;42:E177-85. [Crossref] [PubMed]
- Mooney J, Michalopoulos GD, Alvi MA, et al. Minimally invasive versus open lumbar spinal fusion: a matched study investigating patient-reported and surgical outcomes. J Neurosurg Spine 2022;36:753-66. [Crossref] [PubMed]
- Weiss H, Garcia RM, Hopkins B, et al. A Systematic Review of Complications Following Minimally Invasive Spine Surgery Including Transforaminal Lumbar Interbody Fusion. Curr Rev Musculoskelet Med 2019;12:328-39. [Crossref] [PubMed]
- Wong AP, Smith ZA, Nixon AT, et al. Intraoperative and perioperative complications in minimally invasive transforaminal lumbar interbody fusion: a review of 513 patients. J Neurosurg Spine 2015;22:487-95. [Crossref] [PubMed]
- Khalifeh JM, Dibble CF, Stecher P, et al. Transfacet Minimally Invasive Transforaminal Lumbar Interbody Fusion With an Expandable Interbody Device-Part I: 2-Dimensional Operative Video and Technical Report. Oper Neurosurg (Hagerstown) 2020;19:E473-9. [Crossref] [PubMed]
- Khalifeh JM, Dibble CF, Stecher P, et al. Transfacet Minimally Invasive Transforaminal Lumbar Interbody Fusion With an Expandable Interbody Device-Part II: Consecutive Case Series. Oper Neurosurg (Hagerstown) 2020;19:518-29. [Crossref] [PubMed]
- Hioki A, Miyamoto K, Kodama H, et al. Two-level posterior lumbar interbody fusion for degenerative disc disease: improved clinical outcome with restoration of lumbar lordosis. Spine J 2005;5:600-7. [Crossref] [PubMed]
- Schwab F, Patel A, Ungar B, et al. Adult spinal deformity-postoperative standing imbalance: how much can you tolerate? An overview of key parameters in assessing alignment and planning corrective surgery. Spine (Phila Pa 1976) 2010;35:2224-31. [Crossref] [PubMed]
- Tchachoua Jiembou G, Nda HA, Konan ML. Evaluation of lordosis recovery after lumbar arthrodesis and its clinical impact. Chin Neurosurg J 2023;9:18. [Crossref] [PubMed]
- Hawasli AH, Khalifeh JM, Chatrath A, et al. Minimally invasive transforaminal lumbar interbody fusion with expandable versus static interbody devices: radiographic assessment of sagittal segmental and pelvic parameters. Neurosurg Focus 2017;43:E10. [Crossref] [PubMed]
- McMordie JH, Schmidt KP, Gard AP, et al. Clinical and Short-Term Radiographic Outcomes of Minimally Invasive Transforaminal Lumbar Interbody Fusion With Expandable Lordotic Devices. Neurosurgery 2020;86:E147-55. [Crossref] [PubMed]
- Lowe TG, Hashim S, Wilson LA, et al. A biomechanical study of regional endplate strength and cage morphology as it relates to structural interbody support. Spine (Phila Pa 1976) 2004;29:2389-94. [Crossref] [PubMed]
- Cadman J, Sutterlin C 3rd, Dabirrahmani D, et al. The importance of loading the periphery of the vertebral endplate. J Spine Surg 2016;2:178-84. [Crossref] [PubMed]
- Yao YC, Chou PH, Lin HH, et al. Risk Factors of Cage Subsidence in Patients Received Minimally Invasive Transforaminal Lumbar Interbody Fusion. Spine (Phila Pa 1976) 2020;45:E1279-85. [Crossref] [PubMed]
- Copay AG, Glassman SD, Subach BR, et al. Minimum clinically important difference in lumbar spine surgery patients: a choice of methods using the Oswestry Disability Index, Medical Outcomes Study questionnaire Short Form 36, and pain scales. Spine J 2008;8:968-74. [Crossref] [PubMed]
- Zhao L, Xie T, Wang X, et al. Clinical and radiological evaluation of cage subsidence following oblique lumbar interbody fusion combined with anterolateral fixation. BMC Musculoskelet Disord 2022;23:214. [Crossref] [PubMed]
- Malham GM, Parker RM, Blecher CM, et al. Assessment and classification of subsidence after lateral interbody fusion using serial computed tomography. J Neurosurg Spine 2015;23:589-97. [Crossref] [PubMed]
- Ledesma JA, Lambrechts MJ, Dees A, et al. Static versus Expandable Interbody Fusion Devices: A Comparison of 1-Year Clinical and Radiographic Outcomes in Minimally Invasive Transforaminal Lumbar Interbody Fusion. Asian Spine J 2023;17:61-74. [Crossref] [PubMed]
- Calvachi-Prieto P, McAvoy MB, Cerecedo-Lopez CD, et al. Expandable Versus Static Cages in Minimally Invasive Lumbar Interbody Fusion: A Systematic Review and Meta-Analysis. World Neurosurg 2021;151:e607-14. [Crossref] [PubMed]
- De Cassai A, Geraldini F, Boscolo A, et al. General Anesthesia Compared to Spinal Anesthesia for Patients Undergoing Lumbar Vertebral Surgery: A Meta-Analysis of Randomized Controlled Trials. J Clin Med 2020;10:102. [Crossref] [PubMed]
- Meng T, Zhong Z, Meng L. Impact of spinal anaesthesia vs. general anaesthesia on peri-operative outcome in lumbar spine surgery: a systematic review and meta-analysis of randomised, controlled trials. Anaesthesia 2017;72:391-401. [Crossref] [PubMed]
- Zorrilla-Vaca A, Healy RJ, Mirski MA. A Comparison of Regional Versus General Anesthesia for Lumbar Spine Surgery: A Meta-Analysis of Randomized Studies. J Neurosurg Anesthesiol 2017;29:415-25. [Crossref] [PubMed]
- Sykes DAW, Tabarestani TQ, Chaudhry NS, et al. Awake Spinal Fusion Is Associated with Reduced Length of Stay, Opioid Use, and Time to Ambulation Compared to General Anesthesia: A Matched Cohort Study. World Neurosurg 2023;176:e91-e100. [Crossref] [PubMed]
- Epstein NE. More nerve root injuries occur with minimally invasive lumbar surgery, especially extreme lateral interbody fusion: A review. Surg Neurol Int 2016;7:S83-95. [Crossref] [PubMed]
- Hardenbrook M, Lombardo S, Wilson MC, et al. The anatomic rationale for transforaminal endoscopic interbody fusion: a cadaveric analysis. Neurosurg Focus 2016;40:E12. [Crossref] [PubMed]
- Ishihama Y, Morimoto M, Tezuka F, et al. Full-Endoscopic Trans-Kambin Triangle Lumbar Interbody Fusion: Surgical Technique and Nomenclature. J Neurol Surg A Cent Eur Neurosurg 2022;83:308-13. [Crossref] [PubMed]
- Kumari C, Gupta T, Gupta R, et al. Cadaveric anatomy of the lumbar triangular safe zone of Kambin's in North West Indian population. Anat Cell Biol 2021;54:35-41. [Crossref] [PubMed]
- Morimoto M, Wada K, Tamaki S, et al. Clinical Outcome of Full Endoscopic Trans Kambin's Triangle Lumbar Interbody Fusion: A Systematic Review. World Neurosurg 2023;178:317-29. [Crossref] [PubMed]
- Tabarestani TQ, Sykes DAW, Kouam RW, et al. Novel Approach to Percutaneous Lumbar Surgeries via Kambin's Triangle-Radiographic and Surgical Planning Analysis with Nerve Segmentation Technology. World Neurosurg 2023; Epub ahead of print. [Crossref] [PubMed]
- Tabarestani TQ, Salven DS, Sykes DAW, et al. Using Novel Segmentation Technology to Define Safe Corridors for Minimally Invasive Posterior Lumbar Interbody Fusion. Oper Neurosurg (Hagerstown) 2023; Epub ahead of print. [Crossref] [PubMed]
- Liu J, Duan P, Mummaneni PV, et al. Does transforaminal lumbar interbody fusion induce lordosis or kyphosis? Radiographic evaluation with a minimum 2-year follow-up. J Neurosurg Spine 2021;35:419-26. [Crossref] [PubMed]
- Alvi MA, Kurian SJ, Wahood W, et al. Assessing the Difference in Clinical and Radiologic Outcomes Between Expandable Cage and Nonexpandable Cage Among Patients Undergoing Minimally Invasive Transforaminal Interbody Fusion: A Systematic Review and Meta-Analysis. World Neurosurg 2019;127:596-606.e1. [Crossref] [PubMed]
- Lin GX, Kim JS, Kotheeranurak V, et al. Does the application of expandable cages in TLIF provide improved clinical and radiological results compared to static cages? A meta-analysis. Front Surg 2022;9:949938. [Crossref] [PubMed]
- Hong TH, Cho KJ, Kim YT, et al. Does Lordotic Angle of Cage Determine Lumbar Lordosis in Lumbar Interbody Fusion? Spine (Phila Pa 1976) 2017;42:E775-80. [Crossref] [PubMed]
- Lovecchio FC, Vaishnav AS, Steinhaus ME, et al. Does interbody cage lordosis impact actual segmental lordosis achieved in minimally invasive lumbar spine fusion? Neurosurg Focus 2020;49:E17. [Crossref] [PubMed]
- Tian H, Wu A, Guo M, et al. Adequate Restoration of Disc Height and Segmental Lordosis by Lumbar Interbody Fusion Decreases Adjacent Segment Degeneration. World Neurosurg 2018;118:e856-64. [Crossref] [PubMed]
- Chang CC, Chou D, Pennicooke B, et al. Long-term radiographic outcomes of expandable versus static cages in transforaminal lumbar interbody fusion. J Neurosurg Spine 2020;34:471-80. [Crossref] [PubMed]
- Choi WS, Kim JS, Hur JW, et al. Minimally Invasive Transforaminal Lumbar Interbody Fusion Using Banana-Shaped and Straight Cages: Radiological and Clinical Results from a Prospective Randomized Clinical Trial. Neurosurgery 2018;82:289-98. [Crossref] [PubMed]
- Massie LW, Zakaria HM, Schultz LR, et al. Assessment of radiographic and clinical outcomes of an articulating expandable interbody cage in minimally invasive transforaminal lumbar interbody fusion for spondylolisthesis. Neurosurg Focus 2018;44:E8. [Crossref] [PubMed]
- Steffen T, Tsantrizos A, Aebi M. Effect of implant design and endplate preparation on the compressive strength of interbody fusion constructs. Spine (Phila Pa 1976) 2000;25:1077-84. [Crossref] [PubMed]
- Briski DC, Goel VK, Waddell BS, et al. Does Spanning a Lateral Lumbar Interbody Cage Across the Vertebral Ring Apophysis Increase Loads Required for Failure and Mitigate Endplate Violation. Spine (Phila Pa 1976) 2017;42:E1158-64. [Crossref] [PubMed]
- Park DY, Heo DH. The Use of Dual Direction Expandable Titanium Cage With Biportal Endoscopic Transforaminal Lumbar Interbody Fusion: A Technical Consideration With Preliminary Results. Neurospine 2023;20:110-8. [Crossref] [PubMed]