The effect of expandable versus static lordotic interbody implants in minimally invasive spine surgery: patient reported outcomes, sagittal alignment, and restoration of disc height and foraminal height
Introduction
Though the population in the United States is aging, individuals are remaining more active and placing greater demands on their musculoskeletal system (1-5). As a result, a key healthcare problem is that pain and disability due to age-related spinal disorders will also increase (6-9). These patients are initially treated conservatively with interventions such as physical therapy, lifestyle modifications, and pharmacological therapies to manage their pain. However, when these treatments are no longer efficacious, they may need to resort to invasive surgical options such as spinal fusion. Specifically, standard open posterior approaches to the spine are associated with significant muscle morbidity, including atrophy, denervation, and scarring (10,11). Such complications can increase postoperative pain and hospital stays, with the possibility of developing chronic, long-term pain (12,13).
The use of minimally invasive surgery (MIS) techniques for spine surgery, such as posterior lumbar interbody fusion, provides an opportunity to treat these patients with less morbidity than traditional open surgery (14,15). Various MIS approaches are being developed for spinal deformities with encouraging preliminary results (16). For the lumbar spine, several authors have documented similar outcomes with decreased blood loss and hospital stay utilizing MIS versus open approaches (17-19). Posterior MIS approaches have been used as stand-alone instrumentation and as supplements to anterior column reconstruction. Several authors have shown similar surgical outcomes with decreased blood loss and infection rates in patients treated with minimally invasive posterior approaches (20-22). Additional MIS techniques, such as transforaminal lumbar endoscopic decompression procedures, have recently been shown to have excellent perioperative and long-term quality of life outcomes (23,24).
Interbody fusion cages were introduced to improve fusion rates and restore lumbar lordosis, intervertebral disc height, and foraminal height (25-29). Initial designs of interbody fusion cages, also known as static cages, were offered in multiple different sizes and shapes to account for variability in patients’ anatomy (29). Current drawbacks of static cage designs are related to implant subsidence issues and loss of lordosis, which can negatively impact patient outcomes, leading to the development of expandable cage devices (25,26,29-31). The advent of minimally invasive expandable interbody devices continues to improve on these clinical successes and medical economic advancements by incorporating design features to reduce additional surgical time, as well as steps designed to reduce iatrogenic impact to the neurological and bony anatomy (29). The expandable device is inserted through a minimally invasive approach while maximizing vertical height restoration for optimum anterior column support (29,32-36). Though expandable cages were developed to improve upon the limitations of static cages, additional clinical evidence in this area is needed (37-40). The purpose of this study was therefore to collect and compare multiple outcome metrics over a two-year postoperative period following transforaminal lumbar spinal fusion via MIS with either a static polyether-ether-ketone (PEEK) spacer or an expandable interbody spacer. We present the following article in accordance with the STROBE reporting checklist (available at https://jss.amegroups.com/article/view/10.21037/jss-22-55/rc).
Methods
Study objectives
The objectives of this study were to review and analyze retrospectively collected perioperative, radiographic, and clinical outcome data following treatment with either a static or minimally invasive expandable transforaminal lumbar interbody fusion (TLIF) device for the treatment of spondylolisthesis, degenerative disc disease, spinal stenosis, disc herniation, or degenerative scoliosis. The primary goals of this study were to evaluate radiographic and spinopelvic changes, patient-reported outcomes (PROs), and the incidence of non-union and revision surgery. The study was conducted in accordance with the Declaration of Helsinki (as revised in 2013). This study was approved by the WIRB-Copernicus Group (WCG) Institutional Review Board (IRB# 20212789) and individual consent for this retrospective analysis was waived.
Subjects
Inclusion criteria
- Between 18–90 years of age (inclusive and skeletally mature).
- A diagnosis of spondylolisthesis, degenerative disc disease, spinal stenosis, disc herniation, or degenerative scoliosis (any curve magnitude).
- Spinal fusion undertaken via the transforaminal lumbar minimally invasive approach, with supplemental posterior instrumentation where the physician had decided that use of a static PEEK or expandable spacer was in the best interests of the patient.
Exclusion criteria
- Involved in spinal litigation (e.g., workers’ compensation).
Study procedures
Subject data were obtained from a single physician’s retrospective patient pool who met the inclusion and exclusion criteria. Patients undergoing surgery using the static implants were chosen prior to the development of the expandable implant. The expandable implants were then used in the subsequent 50 patients. Patients were chosen without regard to outcomes. The study was carried out in accordance with the international standards on clinical trials: Real Decreto 223/2004, Declaration of Helsinki in its latest revised version, and Good Clinical Practice Regulations (International Conference for Harmonization).
Confirmation of study eligibility was made by the investigator utilizing the pre-operative images. At the time of the retrospective data collection, the identified subjects had the following documented if clinically relevant:
- Demographics (age, gender, smoking history);
- Physical exam;
- Diabetes mellitus, including fasting plasma glucose (FPG) measurement, oral glucose tolerance tests (OGTT), and standardized hemoglobin A1c (HbA1c) assays;
- History of hypertension;
- Pertinent imaging studies that were obtained as part of standard of care preoperative planning, including dynamic radiographs (anterior-posterior, flexion/extension laterals), magnetic resonance imaging (MRI), or computed tomography (CT) scans, if clinically relevant;
- Fusion criteria applied to all the patients in the study followed the Millman criteria for fusion, which is the industry and insurance standard, which defines instability and the need for fusion as having a spondylolisthesis and having 3 to 5 mm of translation noted on forward bending and backward extension laterals.
Operative suite reports were used as documentation for the surgical approach that was utilized, as well as posterior fixation selection. The patient’s spine level (i.e., L4-L5 or L5-S1) and implant height were also recorded.
Any complications during and after surgery were captured. Subjects were selected by the investigating surgeon who returned to see their study physician at 1 week, 6 weeks, 3 months, 6 months, 12 months, and 2 years were included in the analysis.
Study interventions
Retrospective data were included from patients who underwent minimally invasive spine surgery for the treatment of spondylolisthesis, degenerative disc disease, spinal stenosis, disc herniation, or degenerative scoliosis at L4-L5 or L5-S1. Subjects were divided into groups based on whether a static PEEK spacer or expandable interbody spacer was used. In both groups, a 50/50 mixture of autograft and allograft was used.
The ProLift® Expandable Spacer System (Life Spine Inc, Huntley, Illinois) is intended to serve as an intervertebral body fusion device (Figure 1). The implant is available in a range of sizes and footprints to suit the individual pathology and anatomical conditions of the patient. It is fabricated and manufactured from surgical titanium (6Al4V). The implant is hollow to permit packing with autogenous bone grafting to help promote intervertebral body fusion. The superior and inferior surfaces have teeth to assist in the interface with the vertebral endplates to prevent rotation and migration.
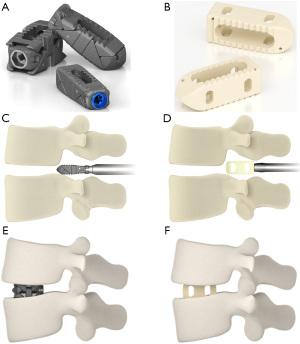
The static implant was a fixed PEEK 15-degree lordotic interbody implant manufactured by Precision Spine (Parsippany, New Jersey) with design feature of having a bullet tip and a hollow core for packing with autologous bone graft prior to insertion. The implant allows the placement of the implant via an insert and rotate mechanism of insertion which then allows proper seating of the implant on the endplates.
Outcome measures
The following outcomes were measured and analyzed:
- Intraoperative complications.
- Radiographic evidence of decompression, as assessed by the interbody height, foraminal height, segmental lordosis, and fusion rates (Figure 2).
- Image assessments were performed by a single surgeon with 34 years of experience.
- Fusion was assessed on anterior-posterior, lateral, and flexion-extension lateral radiographs. The definition of fusion was bridging bone anteriorly with a positive sentinel sign, bone noted in the implant and within the disc space, no lucent lines about the implants, and no evidence of subsidence. If needed, a CT scan (1 mm thin cut) was performed to confirm or deny pseudoarthrosis.
- Oswestry Disability Index (ODI).
- Visual Analogue Scale (VAS) for Back and Leg Pain.
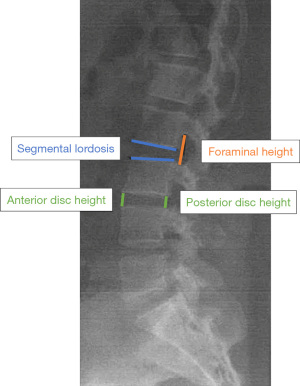
Statistical analysis
Baseline characteristics were summarized using descriptive statistics, where continuous data were reported as means with standard deviations, and categorical data were presented as counts and proportions. To compare the differences in the changes in radiographic outcomes and PROs between treatment groups, a two-sided Independent Samples t-test was conducted. A two-sided paired sample t-test was also conducted for within-group comparisons. A chi-squared test was conducted to compare the incidence of non-union and revision between treatment groups at 2 years, and relative risks (RR) were calculated. A P value of less than 0.05 was considered to be statistically significant. All statistical analyses were conducted using a statistical software package (R version 4.0.1). Subgroup analyses were also conducted based on patients’ spine level (i.e., L4-L5 or L5-S1), physical examination finding (i.e., back pain-positive or back pain-negative), and diagnoses for radiographic measures and PROs at final follow-up.
Results
Baseline characteristics
Baseline characteristics of the treatment groups are summarized in Table 1. Gender distribution, age, proportion of smokers, physical examination results, and spine level distribution (L4-L5 or L5-S1) were similar between the two groups. In this study, all smokers stopped smoking 6 weeks prior to the surgery, confirmed through preoperative carboxymethyl hemoglobin levels. In terms of comorbidities, a greater proportion of Expandable patients had hypertension or diabetes. The proportion of patients diagnosed with radiculopathy, facet arthropathy, or prior surgery was similar between groups; however, the proportion of patients diagnosed with spondylosis, spondylolisthesis, or spinal stenosis was relatively higher in the Static group, whereas the proportion of patients diagnosed with spinal instability or herniated nucleus pulposus was higher in the Expandable group. Additionally, the two groups have the same proportion of patients with an implant height of 10 mm; however, the Static group had more patients with an implant height of 8 and 12 mm, whereas the Expandable group had more patients with an implant height of 11, 13, and 14 mm. While implant heights varied per patient, there was no difference in implant footprints between groups or patients.
Table 1
Characteristics | Static (n=50) | Expandable (n=50) |
---|---|---|
Female, n [%] | 24 [48] | 26 [52] |
Age in years, mean (SD) | 56.9 (7.7) | 57.9 (7.3) |
Smoker, n [%] | 22 [44] | 25 [50] |
Hypertension, n [%] | 18 [36] | 25 [50] |
Diabetes mellitus, n [%] | 9 [18] | 14 [28] |
Physical examination, n [%] | ||
Back pain | 28 [56] | 32 [64] |
Radiculopathy | 50 [100] | 50 [100] |
Diagnosis, n [%] | ||
Spondylosis | 30 [60] | 24 [48] |
Spondylolisthesis | 35 [70] | 29 [58] |
Spinal instability | 29 [58] | 36 [72] |
Spinal stenosis | 29 [58] | 21 [42] |
Herniated nucleus pulposus | 16 [32] | 23 [46] |
Radiculopathy | 39 [78] | 38 [76] |
Facet arthropathy | 18 [36] | 21 [42] |
Prior surgery | 13 [26] | 10 [20] |
Spine level, n [%] | ||
L4-L5 | 19 [38] | 21 [42] |
L5-S1 | 31 [62] | 29 [58] |
Implant height, mm, n [%] | ||
8 | 14 [28] | 0 [0] |
10 | 18 [36] | 18 [36] |
11 | 1 [2] | 15 [30] |
12 | 17 [34] | 8 [16] |
13 | 0 [0] | 8 [16] |
14 | 0 [0] | 1 [2] |
SD, standard deviation.
Radiographic outcomes
Radiographic outcomes are summarized in Table 2. Changes in anterior disc height, posterior disc height, average disc height, foraminal height, and lordosis improved significantly more in the Expandable group versus the Static group postoperatively and at each follow-up visit up to two years (Figure 3; all P<0.001). In terms of the maintenance of postoperative radiographic improvement at two years, improvements were maintained across all radiographic measures within both groups (Table 3; Figure 4). Figure 5 shows the two-year radiographs of a patient implanted with the expandable device and another with the static device.
Table 2
Outcome | Static | Expandable | MD (95% CI) | P value |
---|---|---|---|---|
Change in anterior disc height (mm), mean (SD) | Baseline =10.7 (2.5) | Baseline =7.7 (0.7) | ||
Postoperative | 1.9 (0.3) | 7.9 (0.5) | 6 (5.9, 6.2) | <0.001 |
6 months | 1.7 (0.4) | 7.8 (0.5) | 6.1 (5.9, 6.3) | <0.001 |
1 year | 1.7 (0.4) | 7.8 (0.5) | 6.1 (5.9, 6.3) | <0.001 |
2 years | 1.9 (0.4) | 7.9 (0.5) | 6 (5.9, 6.2) | <0.001 |
Change in posterior disc height (mm), mean (SD) | Baseline =4.6 (1.3) | Baseline =2.9 (0.7) | ||
Postoperative | 2.5 (0.2) | 7.1 (0.4) | 4.6 (4.5, 4.8) | <0.001 |
6 months | 2.3 (0.2) | 7 (0.4) | 4.7 (4.5, 4.8) | <0.001 |
1 year | 2.3 (0.2) | 7 (0.4) | 4.7 (4.6, 4.8) | <0.001 |
2 years | 2.4 (0.2) | 7.1 (0.4) | 4.7 (4.5, 4.8) | <0.001 |
Change in average disc height (mm), mean (SD) | Baseline =7.7 (1.5) | Baseline =5.3 (0.5) | ||
Postoperative | 2.2 (0.7) | 7.5 (0.3) | 5.3 (5, 5.5) | <0.001 |
6 months | 2.1 (0.8) | 7.3 (NA) | 5.2 (5, 5.5) | <0.001 |
1 year | 2.1 (0.8) | 7.4 (0.4) | 5.3 (5, 5.5) | <0.001 |
2 years | 2.2 (0.7) | 7.5 (0.3) | 5.3 (5, 5.5) | <0.001 |
Change in foraminal height (mm), mean (SD) | Baseline =8.4 (1.0) | Baseline =11.6 (0.9) | ||
Postoperative | 1.9 (0.3) | 7.9 (0.3) | 6 (5.9, 6.1) | <0.001 |
6 months | 1.8 (0.4) | 7.7 (0.3) | 5.9 (5.7, 6) | <0.001 |
1 year | 1.8 (0.5) | 7.7 (0.4) | 5.9 (5.7, 6) | <0.001 |
2 years | 1.8 (0.5) | 7.8 (0.4) | 6 (5.8, 6.2) | <0.001 |
Change in lordosis in degrees, mean (SD) | Baseline =8.4 (1.1) | Baseline =6.2 (0.9) | ||
Postoperative | 2 (0.3) | 7.1 (2.1) | 5.1 (4.5, 5.7) | <0.001 |
6 months | 1.9 (0.5) | 7 (2.1) | 5.1 (4.5, 5.7) | <0.001 |
1 year | 1.9 (0.7) | 7 (2.1) | 5.1 (4.5, 5.8) | <0.001 |
2 years | 1.8 (0.9) | 7 (2.1) | 5.2 (4.5, 5.8) | <0.001 |
SD, standard deviation; NA, not available; MD, mean difference; CI, confidence interval.
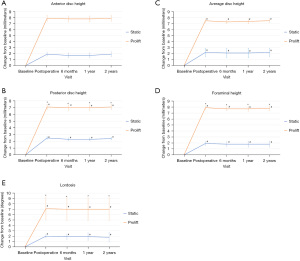
Table 3
Outcome by treatment group | Postoperative | 2 years | MD (95% CI) | P value |
---|---|---|---|---|
Static, mean (SD) | ||||
Anterior disc height, mm | 12.536 (2.481) | 12.526 (2.504) | −0.01 (0.01, −0.03) | 0.3222 |
Posterior disc height, mm | 7.068 (1.306) | 7.044 (1.339) | −0.024 (0.01, −0.058) | 0.1653 |
Average disc height, mm | 9.918 (1.511) | 9.9 (1.534) | −0.018 (0.007, −0.043) | 0.162 |
Foraminal height, mm | 10.318 (0.983) | 10.198 (1.097) | −0.12 (0.006, −0.246) | 0.0624 |
Lordosis, degrees | 10.444 (1.132) | 10.272 (1.232) | −0.172 (0.073, −0.417) | 0.165 |
Expandable, mean (SD) | ||||
Anterior disc height, mm | 15.646 (0.786) | 15.646 (0.786) | 0 (NA, NA) | NA |
Posterior disc height, mm | 10 (0.666) | 10 (0.666) | 0 (NA, NA) | NA |
Average disc height, mm | 12.832 (0.548) | 12.828 (0.547) | −0.004 (0.002, −0.01) | 0.1594 |
Foraminal height, mm | 19.476 (0.888) | 19.394 (1.044) | −0.082 (0.015, −0.179) | 0.0959 |
Lordosis, degrees | 13.268 (2.451) | 13.188 (2.513) | −0.08 (0.014, −0.174) | 0.0929 |
SD, standard deviation; MD, mean difference; CI, confidence interval; NA, not available.
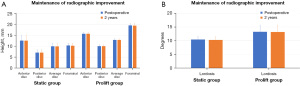
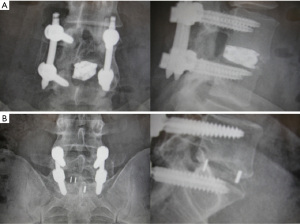
Clinical outcomes
Change from baseline scores in PRO measures are reported in Table 4. Improvements relative to baseline in both the ODI and VAS back pain were significantly more favorable in the Expandable group at all study visits (Figure 6; all P<0.001). The change in VAS leg pain was significantly more improved in the Expandable group at 2 years only (P=0.042).
Table 4
Outcome | Static | Expandable | MD (95% CI) | P value |
---|---|---|---|---|
Change in ODI scores, mean (SD) | Baseline =55.4 (3.5) | Baseline =53.2 (3.7) | ||
3 months | −13.9 (5.2) | −20.5 (5.0) | −6.6 (−4.6, −8.6) | <0.001 |
6 months | −20.7 (5.8) | −27 (3.8) | −6.3 (−4.3, −8.2) | <0.001 |
1 year | −19.7 (7.6) | −26.8 (4.8) | −7.1 (−4.6, −9.7) | <0.001 |
2 years | −18.9 (9.6) | −26.7 (5.5) | −7.8 (−4.6, −10.9) | <0.001 |
Change in VAS back pain scores, mean (SD) | Baseline =73.9 (3.8) | Baseline =72.1 (6.9) | ||
3 months | −21.3 (7.5) | −33.1 (8.7) | −11.8 (−8.6, −15) | <0.001 |
6 months | −36.4 (6.5) | −43.5 (6.0) | −7.1 (−4.6, −9.6) | <0.001 |
1 year | −35.6 (7.5) | −43.8 (4.9) | −8.2 (−5.7, −10.7) | <0.001 |
2 years | −34.1 (10.8) | −43.6 (5.7) | −9.5 (−6.1, −13) | <0.001 |
Change in VAS leg pain scores, mean (SD) | Baseline =79.9 (6.0) | Baseline =81.8 (7.2) | ||
3 months | −71 (12.7) | −74.4 (8.8) | −3.4 (0.9, −7.7) | 0.1239 |
6 months | −76.4 (8.9) | −78.8 (9.3) | −2.4 (1.2, −6) | 0.1908 |
1 year | −74 (14.5) | −78.9 (12.6) | −4.9 (0.5, −10.3) | 0.0751 |
2 years | −74.7 (15.1) | −80.1 (10.6) | −5.4 (−0.2, −10.6) | 0.0415 |
ODI, Oswestry Disability Index; SD, standard deviation; VAS, visual analogue scale; MD, mean difference; CI, confidence interval.
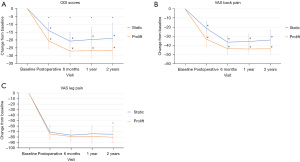
Safety outcomes
No intraoperative complications occurred in either treatment group. At the 2-year follow-up, Expandable cage-treated patients had a lower incidence of non-union (6% vs. 12% for Static) and revision surgery (4% vs. 8% for Static), though the differences between groups were not statistically significant (Table 5). In the Static group, revisions were due to lucent lines and slight subsidence of the implants and persistent pain. In the Expandable cage group, two patients had subsidence due to end plate violation of the implants that necessitated revision. Posterior hardware failure was not noted in either group. No other adverse events were documented over the 2-year follow up period.
Table 5
Outcome | Static, n [%] | Expandable, n [%] | RR (95% CI) | P value |
---|---|---|---|---|
Non-union | 6 [12] | 3 [6] | 0.5 (0.13, 1.89) | 0.487 |
Revision | 4 [8] | 2 [4] | 0.5 (0.1, 2.61) | 0.6777 |
CI, confidence interval; RR, relative risk. RR <1.0 favors the Expandable group.
Subgroup analyses
The results of all subgroup analyses for each radiographic and PRO at 2 years are available in the Supplementary. Similar results were observed, with the Expandable group demonstrating statistically significant improvements in all outcome measures [anterior disc height (Figure S1), posterior disc height (Figure S2), average disc height (Figure S3), foraminal height (Figure S4), lordosis (Figure S5), ODI score (Figure S6), VAS back pain (Figure S7)] except in VAS leg pain scores, which was only statistically significant in the subgroup of patients with spondylolisthesis (P=0.044) (Figure S8).
Discussion
The objective of this study was to compare multiple radiographic and PROs following transforaminal lumbar spinal fusion via MIS between a static PEEK spacer and an expandable interbody spacer up to 2 years following surgery. In terms of radiographic measures, the Expandable group demonstrated statistically significant improvements relative to Static group in anterior disc height, posterior disc height, average disc height, foraminal height, and lordosis from the postoperative visit and each subsequent visit up to 2 years. Additionally, the radiographic improvement achieved postoperatively was maintained throughout the 2 years. With regards to PROs, Expandable cage-treated patients had statistically significant improvements compared to the Static group in ODI and VAS back pain scores as early as 3 months postoperatively, which was sustained up to the 2-year visit. VAS leg pain scores were only statistically significant, in favor of the Expandable group, at the final follow-up. Patient diagnoses may have differed between groups, with the proportion of patients diagnosed with spondylosis, spondylolisthesis, or spinal stenosis being relatively higher in the Static group, and the proportion diagnosed with spinal instability or herniated nucleus pulposus being higher in the Expandable group; however, subgroup analyses revealed similar findings across these different diagnoses. Lastly, though not statistically significant, patients treated with the expandable device also had a lower incidence of both non-union and revision surgery (RR of 0.5 for both).
The use of expandable versus static cages in lumbar spinal fusion has been previously investigated in the literature. In a biomechanical study on eight cadaveric lumbar specimens, an expandable interbody cage demonstrated significantly greater foraminal height, anterior disc height, and posterior disc height at L4-L5 than static cages, though stability, stiffness, and segmental lordosis were similar between treatments (41). Clinical investigations comparing the two devices are limited and predominantly lower quality evidence. In a meta-analysis of expandable versus static cages among patients undergoing minimally invasive transforaminal interbody fusion, Alvi et al. compared both clinical and radiological outcomes between groups (25). They included 12 studies (706 patients) and concluded that there may not be a significant difference between these two groups; however, their results were not based on a meta-analysis of direct head-to-head comparisons (i.e., their conclusions were based on indirect evidence only), and they graded their confidence in the effect estimates across outcomes as low or very low for this reason.
Primary studies directly comparing the two interventions have also been conducted. In a 2017 retrospective study by Hawasli et al. on 45 patients, an expandable cage [mean follow-up =7.1 months (range, 0.9 to 19.8)] led to a greater and more sustained restoration of disc height, foraminal height, and segmental lordosis, and also improved ODI scores compared to a static device [mean follow-up = 14.6 months (range, 0.9 to 26)] (32). Yee et al. conducted a retrospective review of 89 patients who underwent TLIF with either an expandable or static cage, which revealed no significant differences between groups in terms of segmental and lumbar lordosis at the 1-year follow-up (42). Another retrospective cohort by Khechen et al. that included 60 patients showed significantly greater improvements in disc height and foraminal height with an expandable device, but no differences between groups in segmental lordosis or PROs (ODI, VAS back pain, and VAS leg pain) over 6 months (37). Kremer et al. also conducted a retrospective analysis of 99 patients and found that, at both 3 months and final follow-up (average of 67 months for the Static group and 43 months for the Expandable group), patients treated with an expandable cage had significantly more favorable ODI scores and greater foraminal height, but improvements in VAS pain and disc height were not significantly different between groups (38). Another retrospective cohort by Li et al. on 62 patients demonstrated significantly greater improvements in PROs (VAS back and leg pain, and ODI) with an expandable cage versus a static cage by two years, but disc height improvement was significantly greater in the Static group, with no significant differences in foraminal height or segmental lordosis (43). In a study by Vaishnav et al. on 171 patients retrospectively reviewed following TLIF, expandable cages showed more favorable results in restoring posterior disc height and maintaining lordosis in the immediate postoperative period (44). Chang et al. conducted a 10-year retrospective review on 178 patients who underwent TLIF and also found that an expandable cage provided a greater improvement in disc height restoration, but also resulted in a higher incidence of cage subsidence compared to a static cage (45).
In contrast, Frisch et al., in an observational study with a two year follow-up, found that both static and expandable cages significantly improved clinical and radiographic outcomes in patients who underwent a lateral lumbar interbody fusion (LLIF), with no significant difference between treatments, but that the static cage exhibited a significantly higher incidence of implant subsidence (46). The study by Li et al. also showed a greater incidence of implant subsidence in the Static group (43). Overall, these studies provide conflicting results on both radiographic and clinical outcomes between expandable and static cages; however, generally, they show that expandable cages demonstrate no significant difference or an improvement in outcomes versus static cages. Additionally, none of these prior investigations were specifically on the ProLift expandable system, with this study being the first that directly compared this device to a static cage. While the ProLift device is also made with a titanium coating, which helps promote osseointegration (47-50), the prior investigations utilized a variety of different expandable cages with different surface textures and morphologies, which may have led to the conflicting reported results. Hence differences in device characteristics such as this must be considered when comparing between the various expandable cage manufacturers seen across these studies.
In the current study, patients treated with a static cage achieved an anterior disc height of 12.54 mm (L4-L5: 12.20 mm; L5-S1: 12.70 mm), posterior disc height of 7.07 mm (L4-L5: 6.97 mm; L5-S1: 7.13 mm), and average disc height of 9.92 mm (L4-L5: 9.87 mm; L5-S1: 9.95 mm) postoperatively. In expandable-treated patients, these values were 15.65 mm (L4-L5: 15.60 mm; L5-S1: 15.70 mm), 10.00 mm (L4-L5: 10.10 mm; L5-S1: 9.96 mm), and 12.83 mm (L4-L5: 12.90 mm; L5-S1: 12.80 mm), respectively. These measures were maintained over the two-year follow-up period in both groups. Prior studies have measured these radiographic parameters in normal (i.e., heathy) lumbar intervertebral discs. The anterior disc height has ranged from 10.6 to 18.1 mm in the L4-L5 region, and from 9.9 to 18.7 mm in the L5-S1 region (51-53). The posterior disc height has ranged from 6.2 to 10.1 mm in the L4-L5 region, and from 5.2 to 8.5 mm in the L5-S1 region (51-53). The average disc height has ranged from 8.4 to 12.5 mm in the L4-L5 region, and from 7.6 to 10.5 mm in the L5-S1 region (51,52). The expandable device demonstrates disc height values more comparable to the upper end of these ranges for normal lumbar intervertebral discs.
The correlation between radiographic and clinical outcomes has also been studied. In a retrospective cohort of adults with chronic low back pain treated with a 6-week protocol of nonsurgical spinal decompression, Apfel et al. found that reductions in pain scores significantly correlated with increases in disc height, concluding that pain reduction may be mediated, at least in part, through the restoration of disc height (54). The study by Hawasli et al. determined that there was a significant correlation between improvements in ODI scores and segmental lordosis, and ODI scores and disc height, but the correlation with foraminal height was not statistically significant (32). Additionally, Tian et al. found that the restoration of disc height and segmental lordosis, though not foraminal height, significantly decreases the risk of adjacent segment degeneration (55). In the current study, the expandable device demonstrated greater improvements versus the static cage in the same outcome measures used in these previous studies, further supporting the notion that there is a correlation between these radiographic parameters and clinical outcomes.
A limitation of the current study is that it was a retrospective review; however, the data were collected in a manner that allowed for analyses at multiple study visits over two years with no patients missing any outcome data. Patients were not randomized to their assigned intervention, meaning that there was no treatment allocation concealment and outcomes assessment was unblinded, including both radiographic measurements and PROs. A sample size calculation was not performed a priori; therefore, it is unclear if the statistical analysis was adequately powered prior to conducting the study. Funding was provided by the manufacturer of one of the devices studied and is therefore subject to biases. However, patients were chosen without regard to their outcomes, and the results of this study are in line with other, similar studies. Additionally, this study only looked at one brand of expandable cage, and one brand of static cage and thus the findings may not be generalizable to other brands of device. Another limitation related to the generalizability of these findings is that only patients who had surgery at either the L4-L5 or L5-S1 region, meaning that the results of this study cannot be extrapolated to those who require fusion at other lumbar regions. A final potential limitation of this study is the variety of diagnoses studied. However, studying multiple pathologies shows the flexibility and efficacy of the expandable TLIF device.
A strength of this study was that it was a single center and single surgeon study, limiting the impact of the variation in healthcare practices across centers and surgeon expertise bias on patient outcomes; however, this may also compromise the generalizability of these findings. As previously stated, there was no missing data, and the analyses were based on complete outcome data from all eligible patients. Additionally, both radiographic parameters and PROs were evaluated in this study, providing both an objective and subjective (from the patient’s perspective) assessment of the patient’s progression following treatment. The study sample included patients who required lumbar fusion for various spinal indications and, via subgroup analyses by diagnosis, it was found that the results of the overall sample were similar across these different conditions.
Future research in this area is warranted. Prospective studies, preferably randomized controlled trials, with larger sample sizes and long-term patient follow-up are needed to generate higher quality evidence on this topic and more accurately establish the comparative effects between expandable and static cages. Such studies should then be used to inform health economic analyses to determine the potential impact of expandable cages on the financial burden currently placed on the healthcare system and on patients, and their impact on patient quality of life. Future research in this area should focus on prospective studies (e.g., randomized controlled trials) with larger sample sizes and long-term patient follow-up. Health economic evaluations should then be conducted to determine the potential socioeconomic impact of expandable cages on patients and the healthcare system.
Conclusions
In patients who underwent transforaminal lumbar spinal fusion via MIS, the expandable cage demonstrated significantly improved radiographic and PROs compared to a static cage over two years. Subgroup analyses also revealed that results were similar across patients treated at different spine levels (L4-L5 or L5-S1) and across different diagnoses.
Acknowledgments
Funding: None.
Footnote
Reporting Checklist: The authors have completed the STROBE reporting checklist. Available at https://jss.amegroups.com/article/view/10.21037/jss-22-55/rc
Data Sharing Statement: Available at https://jss.amegroups.com/article/view/10.21037/jss-22-55/dss
Peer Review File: Available at https://jss.amegroups.com/article/view/10.21037/jss-22-55/prf
Conflicts of Interest: All authors have completed the ICMJE uniform disclosure form (available at https://jss.amegroups.com/article/view/10.21037/jss-22-55/coif). DWK receives royalties, consulting fees, or other support from the following entities: Medicrea, Precision Spine, NVision Spine, Surgalign, SeaSpine, Spinal Elements, Orthofix/Stryker, Arthrex Spine, and CoreLink; DWK also has patents planned, issued, or pending for Modular Pedicle Screw Design System, SI joint implant design, and 3D printed PEEK interbody implants. EIW, LCS, and CV have received payment for independent medical writing and data analysis from Life Spine. The other author has no conflicts of interest to declare.
Ethical Statement: The authors are accountable for all aspects of the work in ensuring that questions related to the accuracy or integrity of any part of the work are appropriately investigated and resolved. The study was conducted in accordance with the Declaration of Helsinki (as revised in 2013). This study was approved by the WIRB-Copernicus Group (WCG) Institutional Review Board (IRB# 20212789) and individual consent for this retrospective analysis was waived.
Open Access Statement: This is an Open Access article distributed in accordance with the Creative Commons Attribution-NonCommercial-NoDerivs 4.0 International License (CC BY-NC-ND 4.0), which permits the non-commercial replication and distribution of the article with the strict proviso that no changes or edits are made and the original work is properly cited (including links to both the formal publication through the relevant DOI and the license). See: https://creativecommons.org/licenses/by-nc-nd/4.0/.
References
- Pallin DJ, Espinola JA, Camargo CA Jr. US population aging and demand for inpatient services. J Hosp Med 2014;9:193-6. [Crossref] [PubMed]
- White MS, Burns C, Conlon HA. The Impact of an Aging Population in the Workplace. Workplace Health Saf 2018;66:493-8. [Crossref] [PubMed]
- De Biasi A, Wolfe M, Carmody J, et al. Creating an Age-Friendly Public Health System. Innov Aging 2020;4:igz044. [Crossref] [PubMed]
- Dogra S, Dunstan DW, Sugiyama T, et al. Active Aging and Public Health: Evidence, Implications, and Opportunities. Annu Rev Public Health 2022;43:439-59. [Crossref] [PubMed]
- U.S. Census Bureau. Life Expectancy at Birth. 2022. Available online: https://www.census.gov/data/
- Machino M, Nakashima H, Ito K, et al. Age-related degenerative changes and sex-specific differences in osseous anatomy and intervertebral disc height of the thoracolumbar spine. J Clin Neurosci 2021;90:317-24. [Crossref] [PubMed]
- Kim ES, Tkatch R, Martin D, et al. Resilient Aging: Psychological Well-Being and Social Well-Being as Targets for the Promotion of Healthy Aging. Gerontol Geriatr Med 2021;7:23337214211002951. [Crossref] [PubMed]
- Roberts S, Colombier P, Sowman A, et al. Ageing in the musculoskeletal system. Acta Orthop 2016;87:15-25. [Crossref] [PubMed]
- Vo NV, Hartman RA, Patil PR, et al. Molecular mechanisms of biological aging in intervertebral discs. J Orthop Res 2016;34:1289-306. [Crossref] [PubMed]
- Wocial K, Feldman BA, Mruk B, et al. Imaging features of the aging spine. Pol J Radiol 2021;86:e380-6. [Crossref] [PubMed]
- Kawaguchi Y, Matsui H, Gejo R, et al. Preventive measures of back muscle injury after posterior lumbar spine surgery in rats. Spine (Phila Pa 1976) 1998;23:2282-7; discussion 2288. [Crossref] [PubMed]
- Kanaan SF, Melton BL, Waitman LR, et al. The effect of age and gender on acute postoperative pain and function following lumbar spine surgeries. Physiother Res Int 2021;26:e1888. [Crossref] [PubMed]
- Kim DY, Lee SH, Chung SK, et al. Comparison of multifidus muscle atrophy and trunk extension muscle strength: percutaneous versus open pedicle screw fixation. Spine (Phila Pa 1976) 2005;30:123-9.
- Defino HLA, Costa HRT, Nunes AA, et al. Open versus minimally invasive percutaneous surgery for surgical treatment of thoracolumbar spine fractures- a multicenter randomized controlled trial: study protocol. BMC Musculoskelet Disord 2019;20:397. [Crossref] [PubMed]
- Oppenheimer JH, DeCastro I, McDonnell DE. Minimally invasive spine technology and minimally invasive spine surgery: a historical review. Neurosurg Focus 2009;27:E9. [Crossref] [PubMed]
- Anand N, Baron EM, Thaiyananthan G, et al. Minimally invasive multilevel percutaneous correction and fusion for adult lumbar degenerative scoliosis: a technique and feasibility study. J Spinal Disord Tech 2008;21:459-67. [Crossref] [PubMed]
- Dhall SS, Wang MY, Mummaneni PV. Clinical and radiographic comparison of mini-open transforaminal lumbar interbody fusion with open transforaminal lumbar interbody fusion in 42 patients with long-term follow-up. J Neurosurg Spine 2008;9:560-5. [Crossref] [PubMed]
- Foley KT, Lefkowitz MA. Advances in minimally invasive spine surgery. Clin Neurosurg 2002;49:499-517.
- Park P, Upadhyaya C, Garton HJ, et al. The impact of minimally invasive spine surgery on perioperative complications in overweight or obese patients. Neurosurgery 2008;62:693-9; discussion 693-9. [Crossref] [PubMed]
- Korovessis P, Hadjipavlou A, Repantis T. Minimal invasive short posterior instrumentation plus balloon kyphoplasty with calcium phosphate for burst and severe compression lumbar fractures. Spine (Phila Pa 1976) 2008;33:658-67. [Crossref] [PubMed]
- Rampersaud YR, Annand N, Dekutoski MB. Use of minimally invasive surgical techniques in the management of thoracolumbar trauma: current concepts. Spine (Phila Pa 1976) 2006;31:S96-102; discussion S104. [Crossref] [PubMed]
- Sclafani JA, Raiszadeh K, Raiszadeh R, et al. Validation and analysis of a multi-site MIS Prospective Registry through sub-analysis of an MIS TLIF Subgroup. Int J Spine Surg 2014. doi:
10.14444/1004 . eCollection 2014. - Nellensteijn J, Ostelo R, Bartels R, et al. Transforaminal endoscopic surgery for symptomatic lumbar disc herniations: a systematic review of the literature. Eur Spine J 2010;19:181-204. [Crossref] [PubMed]
- Sclafani JA, Raiszadeh K, Laich D, et al. Outcome Measures of an Intracanal, Endoscopic Transforaminal Decompression Technique: Initial Findings from the MIS Prospective Registry. Int J Spine Surg 2015;9:69. [Crossref] [PubMed]
- Alvi MA, Kurian SJ, Wahood W, et al. Assessing the Difference in Clinical and Radiologic Outcomes Between Expandable Cage and Nonexpandable Cage Among Patients Undergoing Minimally Invasive Transforaminal Interbody Fusion: A Systematic Review and Meta-Analysis. World Neurosurg 2019;127:596-606.e1. [Crossref] [PubMed]
- Emstad E, Del Monaco DC, Fielding LC, et al. The VariLift(®) Interbody Fusion System: expandable, standalone interbody fusion. Med Devices (Auckl) 2015;8:219-30. [Crossref] [PubMed]
- Fujimori T, Le H, Schairer WW, et al. Does Transforaminal Lumbar Interbody Fusion Have Advantages over Posterolateral Lumbar Fusion for Degenerative Spondylolisthesis? Global Spine J 2015;5:102-9. [Crossref] [PubMed]
- Humphreys SC, Hodges SD, Patwardhan AG, et al. Comparison of posterior and transforaminal approaches to lumbar interbody fusion. Spine (Phila Pa 1976) 2001;26:567-71. [Crossref] [PubMed]
- Lewandrowski KU, Ferrara L, Cheng B. Expandable Interbody Fusion Cages: An Editorial on the Surgeon's Perspective on Recent Technological Advances and Their Biomechanical Implications. Int J Spine Surg 2020;14:S56-62. [Crossref] [PubMed]
- McAfee PC, DeVine JG, Chaput CD, et al. The indications for interbody fusion cages in the treatment of spondylolisthesis: analysis of 120 cases. Spine (Phila Pa 1976) 2005;30:S60-5. [Crossref] [PubMed]
- Zhang J, Pan A, Zhou L, et al. Comparison of unilateral pedicle screw fixation and interbody fusion with PEEK cage vs. standalone expandable fusion cage for the treatment of unilateral lumbar disc herniation. Arch Med Sci 2018;14:1432-8. [Crossref] [PubMed]
- Hawasli AH, Khalifeh JM, Chatrath A, et al. Minimally invasive transforaminal lumbar interbody fusion with expandable versus static interbody devices: radiographic assessment of sagittal segmental and pelvic parameters. Neurosurg Focus 2017;43:E10. [Crossref] [PubMed]
- Kim CW, Doerr TM, Luna IY, et al. Minimally Invasive Transforaminal Lumbar Interbody Fusion Using Expandable Technology: A Clinical and Radiographic Analysis of 50 Patients. World Neurosurg 2016;90:228-35. [Crossref] [PubMed]
- Li XC, Huang CM, Zhong CF, et al. Minimally invasive procedure reduces adjacent segment degeneration and disease: New benefit-based global meta-analysis. PLoS One 2017;12:e0171546. [Crossref] [PubMed]
- Parker SL, Mendenhall SK, Shau DN, et al. Minimally invasive versus open transforaminal lumbar interbody fusion for degenerative spondylolisthesis: comparative effectiveness and cost-utility analysis. World Neurosurg 2014;82:230-8. [Crossref] [PubMed]
- Patel AA, Zfass-Mendez M, Lebwohl NH, et al. Minimally Invasive Versus Open Lumbar Fusion: A Comparison of Blood Loss, Surgical Complications, and Hospital Course. Iowa Orthop J 2015;35:130-4.
- Khechen B, Haws BE, Patel DV, et al. Static Versus Expandable Devices Provide Similar Clinical Outcomes Following Minimally Invasive Transforaminal Lumbar Interbody Fusion. HSS J 2020;16:46-53. [Crossref] [PubMed]
- Kremer MA, Alferink J, Wynsma S, et al. Expandable spacers provide better functional outcomes than static spacers in minimally invasive transforaminal lumbar interbody fusion. J Spine Surg 2019;5:315-9. [Crossref] [PubMed]
- Soriano-Baron H, Newcomb AGUS, Malhotra D, et al. Biomechanical Analysis of an Expandable Lumbar Interbody Spacer. World Neurosurg 2018;114:e616-23. [Crossref] [PubMed]
- Verma R, Virk S, Qureshi S. Interbody Fusions in the Lumbar Spine: A Review. HSS J 2020;16:162-7. [Crossref] [PubMed]
- Godzik J, Lehrman JN, Newcomb AGUS, et al. Tailoring selection of transforaminal interbody spacers based on biomechanical characteristics and surgical goals: evaluation of an expandable spacer. J Neurosurg Spine 2019; Epub ahead of print. [Crossref]
- Yee TJ, Joseph JR, Terman SW, et al. Expandable vs Static Cages in Transforaminal Lumbar Interbody Fusion: Radiographic Comparison of Segmental and Lumbar Sagittal Angles. Neurosurgery 2017;81:69-74. [Crossref] [PubMed]
- Li YM, Frisch RF, Huang Z, et al. Comparative Effectiveness of Expandable Versus Static Interbody Spacers via MIS LLIF: A 2-Year Radiographic and Clinical Outcomes Study. Global Spine J 2020;10:998-1005. [Crossref] [PubMed]
- Vaishnav AS, Saville P, McAnany S, et al. Retrospective Review of Immediate Restoration of Lordosis in Single-Level Minimally Invasive Transforaminal Lumbar Interbody Fusion: A Comparison of Static and Expandable Interbody Cages. Oper Neurosurg (Hagerstown) 2020;18:518-23. [Crossref] [PubMed]
- Chang CC, Chou D, Pennicooke B, et al. Long-term radiographic outcomes of expandable versus static cages in transforaminal lumbar interbody fusion. J Neurosurg Spine 2020; Epub ahead of print. [Crossref]
- Frisch RF, Luna IY, Brooks DM, et al. Clinical and radiographic analysis of expandable versus static lateral lumbar interbody fusion devices with two-year follow-up. J Spine Surg 2018;4:62-71. [Crossref] [PubMed]
- Assem Y, Mobbs RJ, Pelletier MH, et al. Radiological and clinical outcomes of novel Ti/PEEK combined spinal fusion cages: a systematic review and preclinical evaluation. Eur Spine J 2017;26:593-605. [Crossref] [PubMed]
- Hasegawa T, Ushirozako H, Shigeto E, et al. The Titanium-coated PEEK Cage Maintains Better Bone Fusion With the Endplate Than the PEEK Cage 6 Months After PLIF Surgery: A Multicenter, Prospective, Randomized Study. Spine (Phila Pa 1976) 2020;45:E892-902. [Crossref] [PubMed]
- Massie LW, Zakaria HM, Schultz LR, et al. Assessment of radiographic and clinical outcomes of an articulating expandable interbody cage in minimally invasive transforaminal lumbar interbody fusion for spondylolisthesis. Neurosurg Focus 2018;44:E8. [Crossref] [PubMed]
- Park PJ, Lehman RA. Optimizing the Spinal Interbody Implant: Current Advances in Material Modification and Surface Treatment Technologies. Curr Rev Musculoskelet Med 2020;13:688-95. [Crossref] [PubMed]
- Chow DHK, Yuen EMK, Xiao L, et al. Mechanical effects of traction on lumbar intervertebral discs: A magnetic resonance imaging study. Musculoskelet Sci Pract 2017;29:78-83. [Crossref] [PubMed]
- Hioki A, Miyamoto K, Shimizu K, et al. Test-retest repeatability of lumbar sagittal alignment and disc height measurements with or without axial loading: a computed tomography study. J Spinal Disord Tech 2011;24:93-8. [Crossref] [PubMed]
- Mirab SMH, Barbarestani M, Tabatabaei SM, et al. Measuring Dimensions of Lumbar Intervertebral Discs in Normal Subjects. Anat Sci J 2018;15:3-8.
- Apfel CC, Cakmakkaya OS, Martin W, et al. Restoration of disk height through non-surgical spinal decompression is associated with decreased discogenic low back pain: a retrospective cohort study. BMC Musculoskelet Disord 2010;11:155. [Crossref] [PubMed]
- Tian H, Wu A, Guo M, et al. Adequate Restoration of Disc Height and Segmental Lordosis by Lumbar Interbody Fusion Decreases Adjacent Segment Degeneration. World Neurosurg 2018;118:e856-64. [Crossref] [PubMed]