Assessing in vivo flexion-extension quality of motion after cervical disc arthroplasty: a pilot study
Highlight box
Key findings
• C4-C5 level gives rise to the greatest mean segmental motion contribution during the flexion-extension arc of motion, even after C5-C6 or C6-C7 cervical disc arthroplasty.
• The average segmental motion fractions at C5-C6 and C6-C7 index levels did not show statistical difference compared to the same levels without arthroplasty.
• The highest average motion fractions at C5-C6 and C6-C7 index levels were observed at the two endpoints of flexion-extension.
• The post-arthroplasty shift of centre of location to the superior endplate of the caudal vertebrae is similar to the findings in asymptomatic subjects.
What is known and what is new?
• The study demonstrated physiologic quality of motion at the index segment and harmony among its neighbouring segments after cervical disc arthroplasty, without gearshift-like intermittent locking of the prosthesis during the arc of motion.
What is the implication, and what should change now?
• This pilot study demonstrated the feasibility of investigating cervical disc prostheses using the novel concept of segmental motion fraction as a motion-quality metric. Further long-term studies with larger sample size are required to draw solid conclusions.
Introduction
Historically, anterior cervical discectomy and fusion has been employed as a surgical option to treat cervical spondylosis and neural compression, to restore physiological cervical alignment and to promote bony arthrodesis. One of the inherent issues with cervical fusion is the potential risk of subsequent adjacent segment diseases. Over the past decade, cervical disc arthroplasty has been increasingly adopted as an alternative to address the shortcomings of cervical arthrodesis. In contrast to traditional cervical fusion, cervical disc arthroplasty serves to preserve the segmental motion, avoiding the need for fusion and hence reducing the risk of accelerated degeneration at the adjacent levels. Cervical disc arthroplasty also avoids the need for bone grafting and eliminates the risk of pseudoarthrosis.
Previous studies of in vivo motion assessment after cervical disc arthroplasty have been limited to measuring the range of motion from static lateral radiographs taken at the extremes of full flexion and extension (1-3). Range of motion is important, but it fails to characterise the mid-range motion where the majority of daily living activities take place. The limitations of this quantitative motion assessment have been addressed by Boselie et al. The team previously published a study protocol describing the assessment of the dynamic process of cervical spine motion using the sequence of segmental contributions (4). It is the quality of motion between the flexion and extension endpoints that is more important as this occurs on a day-to-day basis and is an important factor in placing stress on the adjacent segments and facet joints. Intermittent locking of a prosthesis over a portion of the arc of motion can result in non-physiologic kinematics, leading to excessive wear of the prosthesis and stress-shielding at the adjacent segments (5).
The present pilot study serves to assess the quality of index segment motion in relation to the whole cervical spine using dynamic fluoroscopic images obtained over the full range of flexion-extension motion following cervical disc arthroplasty using a M6-C artificial disc (Orthofix Medical Inc., Lewisville, TX, USA). Motion segments with these implanted devices have been demonstrated in a cadaveric study to function in harmony with other segments of the cervical spine as before cervical disc arthroplasty, with smooth sequence of movements across the arc of motion without gearshift-like intermittent locking of the prosthesis. Excellent clinical outcomes following cervical disc arthroplasty have been demonstrated through multiple clinical studies (6-10). Segmental contributions to the global cervical spine motion in healthy adult subjects have been shown to vary during different motion ranges of flexion and extension (11). The highest average contribution is typically contributed by the mid-cervical spine, with successively reduced contributions from the adjacent levels during flexion-extension motion. A study on the ProDisc-C prosthesis (Synthes Spine, West Chester, PA, USA) has shown maintenance of relative contributions from the index operative level to the total cervical range of motion. This was statistically equivalent from baseline to 24 months of follow-up, in contrast to the comparison group with cervical arthrodesis (12). However, in vivo segmental motion contribution following an M6-C cervical disc arthroplasty has not previously been studied.
The notion of instantaneous centre of rotation was first described by Penning in 1988 (13). A recent study in asymptomatic subjects with a mean age of 46 years old shows that the average superior-inferior instantaneous centre of rotation moves progressively more superior from C2-C3 motion segment to C6-C7 motion segment; and the average anterior-posterior location of the instantaneous centre of rotation path is posterior to the geometric centre of the caudal vertebral body (14). Various studies using this kinematic quality parameter has been performed to assess the quality of motion after cervical disc arthroplasty, with variable results in preserving the physiological location of pre-operative centre of rotation (15-19). Nonetheless, the centre of rotation at the index level after cervical disc arthroplasty with an M6-C prosthesis has not been investigated in the past.
This is a unique study investigating the quality of in vivo kinematics of implanted cervical disc prostheses using fluoroscopic images. In this study, we aimed to assess: (I) segmental and global range of cervical spine motions from C2 to C7; (II) the relative contribution by individual motion segments to the total cervical spine motion, termed segmental motion fraction; (III) its variation through the arc of flexion-extension motion between index and adjacent cranial and caudal segments (if available); (IV) centre of rotation. We present the following article in accordance with the STROBE reporting checklist (available at https://jss.amegroups.com/article/view/10.21037/jss-22-62/rc).
Methods
Patient population
The study was conducted in accordance with the Declaration of Helsinki (as revised in 2013). The study was approved by the Tasmania Health and Medical Human Research Ethics Committee of the University of Tasmania (No. 20264) and informed consent was provided by all individual participants. Subjects older than 18 years of age and had a single-level C5-C6 or C6-C7 cervical disc arthroplasty performed by the senior author (N Thani) in 2019 and 2020 were recruited consecutively. The minimum duration between the arthroplasty surgery and the recording of the fluoroscopic images was 3 months for all the participants. Patients with a previous history of cervical fusion or posterior cervical operation, and pregnant women were excluded from the study.
Radiographic analysis
Eligible subjects underwent dynamic fluoroscopic imaging of the entire cervical spine to evaluate the range of motion and the kinematics of the prosthesis. All participants were pain-free on the day of imaging. In standing position, the subjects were instructed to continuously move their head and neck through the entire range of flexion-extension at a constant rate over a period of 10 seconds, with 15 frames captured per second. A static image with the subject looking straight ahead with the head in neutral position was also collected. The average dose of radiation exposure from the lateral fluoroscopy was predicted to be in the range of 10 to 15 µGy·m2. Each subject was allocated a unique subject identification number so that they could be de-identified prior to data analysis. Recorded dynamic fluoroscopic images were stored in an anonymous and confidential manner.
Data analysis was performed using custom digitised software (Quantitative Motion Analysis, Medical Metrics Inc., Houston, TX, USA). Anatomical identifications based on bony landmarks were digitised using the software for radiographic analysis. The image study encompassed the anterior and posterior corners of the superior and inferior endplates from C2 to C7 vertebrae. This validated radiographic motion analysis software uses advanced pattern recognition algorithms to generate accurate measurements of intervertebral rotation, translation and change in disc height measurement error of no more than 0.5 degrees and 0.5 mm. The reproducibility of the measurements has also been validated in various studies including in the cervical spine (20-23). The fluoroscopic images were subsampled (every 10th frame were tracked) to obtain 15 frames for motion analysis. The frame numbers were assigned such that Frame 1 corresponds to full extension, Frame 8 corresponds to neutral, and Frame 15 corresponds to full flexion. The intervertebral flexion-extension angle in each frame of the dynamic fluoroscopy was normalised to the static neutral image for each subject. The size of the implants was used for calibration purposes. For distance-based analysis, we reported the data normalized to the superior enplate of the caudal vertebra.
Data analysis
The fluoroscopic images were analysed to calculate segmental motion fractions as the ratios of angular motion contributions made by individual segments to the motion of the C2-C7 spine at each of the intermediate image collected over the flexion-extension arc of motion. For each cervical spine, we calculated the mean values of segmental contributions to the total C2-C7 motion, averaged over the segmental arc of motion after disc arthroplasty. We arbitrarily defined global cervical range of motion from C2 to C7 in each patient as 100%. We also assessed the variation of each segment’s motion contribution from its mean over the arc of motion. The variation from the mean contribution was assessed using the peak deviation from the mean segmental motion fraction.
We have also assessed the centre of rotation at the index level using the fluoroscopic flexion-extension images. Movement of a cervical spine segment involves angular and translational motions of one vertebra relative to the other. In this study, the centre of rotation was determined by the amount of translation for each degree of angular motion using the Cartesian coordinate system as the point about which the superior vertebra rotates relative to the inferior vertebra. The reference point is located at the midpoint of the superior endplate of the inferior vertebra (Figure 1). The x-axis is parallel to the superior endplate in the anterior-posterior direction. Positive x-values indicate the centre of rotation is anterior to the centre of the endplate. The y-axis is orthogonal to the superior endplate in the cranial-caudal direction. Positive y-values indicate the centre of rotation is below the superior endplate. The centre of rotation is calculated using 15 representative frames from full extension to full flexion. The measurement is dependent on the presence of sufficient angular motion between frames in order for it to be reported reliably. The angular motion threshold at which the centre of rotation was calculated was 3 degrees. The shift in centres of rotation in superior-inferior and anterior-posterior directions were plotted on the coordinate system in Figure 2 for C2-C3 to C6-C7 motion segments.
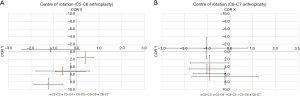
Statistical analysis
Continuous variables were presented as mean ± standard deviation. Individual two-tailed t-tests were used to assess for clinically-significant differences in the average segmental motion fractions for all cervical spine motion segments, comparing C5-C6 disc arthroplasty versus C6-C7 disc arthroplasty patients. Statistical significance was assessed at P values <0.05.
Results
Ten participants were enrolled, including four males and six females with an average age of 43.8 years old (Table 1). The data were collected over a period of 2 months in October to December 2020. The average timing of fluoroscopy was 16.2 months after the surgery (median 12 months). Surgical indications were cervical spondylotic radiculopathy secondary to disc herniation or foraminal stenosis. The motion segments adjacent to the index level (C4-C5 and C6-C7 levels for C5-C6 arthroplasty; C5-C6 level for C6-C7 arthroplasty) showed either Kellgren grade 1 (minimal) or 2 (mild) spondylosis in all subjects in this study. Three subjects had C5-C6 cervical disc arthroplasty and seven had C6-C7 cervical arthroplasty. Based on previous studies, insertion of an appropriately-sized artificial cervical disc prosthesis is essential to avoid over-distraction of the disc space with associated reduction in range of motion and increased facet joint pressure (24,25). In this study, all implanted devices were 6 mm in size. On the other hand, footprint mismatch has been known to cause subsidence, heterotopic ossification, and device failure due to uneven load distribution (26,27). An implant with the largest footprint was chosen for individual patients to provide maximal coverage of the endplates. In four subjects, a large footprint implant was chosen. A large, long footprint prosthesis was inserted for six patients. Subject No. 2 was excluded from the data analysis due to poor visibility of C2, C3 and C7 levels during the motion analysis.
Table 1
Variables | C5-C6 | C6-C7 | P values |
---|---|---|---|
Age (years) | 46.0±13.5 | 41.8±10.2 | 0.62 |
Gender | |||
Male | 0 | 3 | |
Female | 3 | 3 | |
Follow-up (months) | 17.7±13.1 | 16.8±15.4 | 0.94 |
Data are presented as mean ± standard deviation.
The C2-C7 cervical spine had an average flexion-extension range of 66.7 degrees (Table 2). For the group of subjects who underwent C5-C6 disc arthroplasty, the contribution of the index segment was 18.9%±2.1% when averaged over the entire flexion-extension arc of motion, with a peak contribution of 24.4%. The mean contribution of the segments above and below the index segment were 24.7%±5.9% and 21.5%±5.1% respectively, with corresponding peak values of 33.0% and 29.6%. On the other hand, the average segmental motion fraction for subjects who went C6-C7 disc arthroplasty was 15.5%±7.4%, with a peak contribution of 25.5%. The mean contribution from the adjacent C5-C6 level was 18.8%±3.2%, with a peak value of 26.5%. The differences in average segmental motion fractions at the C5-C6 and C6-C7 index levels did not show statistical significance compared to the same levels without arthroplasty (P=0.98 and 0.25, respectively) (Table 3).
Table 2
Patients | C2-C7 FE ROM (degrees) | C2-C3 (degrees) | C3-C4 (degrees) | C4-C5 (degrees) | C5-C6 (degrees) | C6-C7 (degrees) |
---|---|---|---|---|---|---|
1 | 74.4 | 13.7 | 18.6 | 24.9 | 10.6 | 6.4 |
3 | 67.8 | 7.6 | 12.6 | 14.4 | 14.4 | 18.8 |
4 | 61.8 | 8.3 | 16.0 | 16.3 | 13.2 | 8.0 |
5 | 59.8 | 9.7 | 15.1 | 17.4 | 9.6 | 7.9 |
6 | 77.9 | 12.6 | 16.5 | 19.0 | 17.8 | 12.0 |
7 | 57.4 | 5.0 | 11.7 | 15.8 | 12.1 | 12.4 |
8 | 58.2 | 11.0 | 11.9 | 13.4 | 10.7 | 11.4 |
9 | 69.1 | 10.7 | 10.5 | 18.4 | 14.2 | 15.4 |
10 | 73.6 | 9.1 | 17.3 | 16.5 | 14.2 | 16.6 |
Average, mean ± SD | 66.7±7.7 | 9.7±2.6 | 14.5±2.9 | 17.3±3.3 | 12.9±2.5 | 12.1±4.2 |
SD, standard deviation; FE, flexion-extension; ROM, range of motion.
Table 3
Spine segment | C5-C6 disc arthroplasty | C6-C7 disc arthroplasty | Difference in average contribution | ||||
---|---|---|---|---|---|---|---|
Average contribution (%) | Peak contribution (%) | Average contribution (%) | Peak contribution (%) | P values | |||
C2-C3 | 14.7±3.7 | 23.3 | 15.7±5.1 | 23.4 | 0.77 | ||
C3-C4 | 20.4±1.4 | 31.5 | 24.1±4.2 | 33.8 | 0.19 | ||
C4-C5 | 24.7±5.9 | 33.0 | 25.8±4.1 | 35.2 | 0.76 | ||
C5-C6 | 18.9±2.1 | 24.4 | 18.8±3.2 | 26.5 | 0.98 | ||
C6-C7 | 21.5±5.1 | 29.6 | 15.5±7.4 | 25.5 | 0.25 |
Data are presented as mean ± standard deviation.
Motion fraction (Figure 3) at index level for three patients with C5-C6 cervical arthroplasty showed the highest average values of 20.6% and 21.4% at the extreme of extension and flexion, respectively. The lowest value is 14.8% at Frame 9. Six patients with C6-C7 disc arthroplasty showed the highest mean motion fractions at the two endpoints, measuring 16.8% and 16.9%, respectively. For these patients, the lowest C6-C7 motion fraction was observed during Frame 10.
The mean cranial-caudal location of the centre of rotation progressively moves more superior from the C2-C3 to C6-C7 motion segment (Figure 2 and Table 4). This remains true when the centre of rotation is calculated from full extension to neutral position, and from the neutral frame to full flexion. The average anterior-posterior location of the centre of location remains posterior to the centre of the superior endplate from full extension to neutral and remains anterior to the midpoint from neutral to full flexion. However, when this is analysed in individual arthroplasty groups, the centre of rotation at C6-C7 level did not change significantly between operated and non-operated cases but has shifted anteriorly at C5-C6 level in the operated cases.
Table 4
Level | COR X (1:15) (mm) | COR Y (1:15) (mm) | COR X (1:8) (mm) | COR Y (1:8) (mm) | COR X (8:15) (mm) | COR Y (8:15) (mm) |
---|---|---|---|---|---|---|
C2-C3 | −0.6±1.3 | 7.3±2.1 | −1.7±1.0 | 6.9±1.9 | 0.5±2.0 | 8.2±2.9 |
C3-C4 | −0.8±0.8 | 5.6±1.5 | −1.7±0.9 | 5.3±1.9 | 0.3±1.9 | 6.3±2.1 |
C4-C5 | −0.9±0.8 | 4.8±1.4 | −1.5±0.8 | 4.0±1.7 | 0.1±1.7 | 5.8±2.9 |
C5-C6 | −0.6±1.0 | 2.3±1.9 | −1.9±2.2 | 2.8±2.7 | 0.5±1.1 | 2.0±3.5 |
C6-C7 | −1.1±1.6 | −0.8±2.3 | −2.0±1.6 | −0.2±2.4 | 0.1±1.4 | −1.3±3.7 |
Data are presented as mean ± standard deviation. COR, centre of rotation.
Discussion
Following traditional anterior cervical discectomy and fusion, loss of motion at the index level is compensated by increased segmental contribution from the adjacent levels, as demonstrated by reports comparing arthrodesis patients to asymptomatic control subjects (12,28). Cervical disc arthroplasty has been proposed to have the benefit of preserving physiologic motions and load sharing at the treated level to reduce the risk of adjacent segment degeneration. Traditional total disc arthroplasty prostheses consist of a sliding, rotational joint without inherent stiffness or resemblance to a normal viscoelastic intervertebral disc structure (29). M6-C disc prosthesis incorporates a compressible core made of a viscoelastic polymer surrounded by woven artificial annulus, providing built-in resistance to angular and translational motions. In this novel in vivo study of motion-quality analysis, we quantified the segmental motion fraction for each motion segment and the variations throughout the entire arc of flexion-extension after cervical disc arthroplasty. We also demonstrated the change of centre of rotation (COR) between the two endpoints.
The study subjects are representative of the typical population being offered cervical disc arthroplasty, with a mean age of 43.8 years old. Following cervical disc arthroplasty at C5-C6 or C6-C7 level, the patients in our study maintain harmonious cervical spine motions, with the greatest mean segmental motion contribution from the C4-C5 level during both flexion and extension of the cervical spine. These findings are in line with previous studies performed in asymptomatic control subjects without a history of previous operation (28,30). The average segmental motion contributions decrease progressively at the adjacent cranial and caudal motion segments (from C4-C5 to C2-3 and from C4-C5 to C6-C7), as reflected in Figure 4 showing the relationship between segmental and global range of cervical spine motions. Of note, the three patients who had cervical disc arthroplasty at the C5-C6 level had reversal of these segmental motion contributions between the C5-C6 and C6-C7 levels, with an average difference of 1.9 degrees.
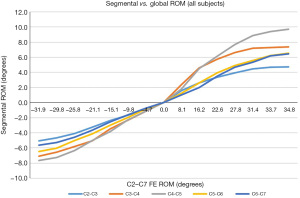
The M6-C artificial disc has previously been shown to maintain normal range of flexion-extension motion in a cadaveric study (31). In a laboratory study investigating applied moment versus segmental angular motion, disc arthroplasty using the prostheses resulted in a small variability in segmental stiffness over the arc of flexion-extension motion and relatively small peak values of motion fraction around the mean (5). The implanted C5-C6 and C6-C7 segments showed small variations in peak segmental motion fractions. This is likely secondary to the intrinsic stiffness of the viscoelastic core and the woven annular design, offering unique internal resistance mechanisms to angular and translational motions, allowing the implanted segments to maintain physiologic range of motion while conferring stability. This is distinct from the Mobi-C device which shows large deviations in motion contribution over the arc of motion despite maintaining similar average motion contributions to pre-implantation values (5). The substantial variability in segmental motion fraction can result in hypermobility, disrupting the harmonious load-sharing with the adjacent segments. The relatively small peak segmental motion fractions of the device can potentially reduce the risk of adjacent segment disease and facet joint loading. In fact, the peak segmental motion fractions for C5-C6 and C6-C7 index levels found in this study were less than those demonstrated in a cadaveric study. Overall, the current study demonstrates that the cervical disc prostheses restored physiologic quality of motion following arthroplasty, such that implanted levels continued to function in harmony with the other segments of the cervical spine.
This analysis confirms that the COR moves closer to the superior endplate of caudal vertebra from C2-C3 to C6-C7 level, similar to findings in asymptomatic subjects without previous operations (14,32,33). Thus for the same degree of movement, a vertebra at the higher cervical segments experiences greater translational motion compared to the lower cervical segments. At C6-C7 level, the mean location of the COR lies at the level of the superior endplate of C7 vertebra. The average anterior-posterior location of the COR is posterior to the geometric centre of the inferior vertebral endplate. Nonetheless, subgroup analysis showed that the centre of rotation at C5-C6 level has shifted anteriorly in the operated cases, compared to the non-operated cases. This may be confounded by the small sample size in this group.
A prosthesis with flexible biomechanical properties can contribute to near-physiological shift of COR and motion pattern, with subsequent impact on clinical outcomes. A systematic review has recently been performed to investigate the shift of COR after cervical arthroplasty (34). It has been found that this is closely related to the type of prostheses used. Traditional artificial prostheses with a ball-and-socket design constrain the COR to the centre of the radius of curvature of the prosthesis. Hence, precise intra-operative placement of the devices with the centre of the ball to match the COR location of a healthy segment is required if they are to mimic normal cervical kinematics (35). A non-constrained cervical disc prosthesis permits independent translation along all three axes in addition to rotation, allowing six degrees-of-freedom and hence able to reproduce the COR in healthy segments. Choosing a cervical disc prosthesis which mimics in vivo cervical spine movements, especially the COR, is important as an abnormal motion path can alter the adjacent segmental loading, leading to accelerated degeneration. A potential flaw in the current literature is that most studies focus on comparing the shift of COR before and after operation. However, the cervical spine has already degenerated prior to the operations. As a result, the location of pre-operative COR may not necessarily reflect that of a healthy cervical spine. This issue is further investigated by a recent study comparing COR between healthy volunteers and patients with a single-level cervical disc prothesis (16). The study suggests that pre-operative COR should not be used as a reference because it is not congruent with the COR found in healthy subjects due to motion pattern changes secondary to degenerative diseases.
Limitations
This study has a few limitations that merit consideration. Firstly, this study has a small sample size with only ten participants, but it serves as a pilot study to assess the feasibility of this novel motion-quality metric. Secondly, cervical spine motions are not limited to flexion-extension. Other movements such as lateral bending, axial rotation, and axial compression are not assessed to verify the controlled range of motion in all six degrees of freedom. However, axial rotation in the cervical spine is always coupled with lateral flexion (36) and it is not feasible to assess these coupled motions using two-dimensional imaging such as uniplanar fluoroscopy. In addition, patient efforts cannot be standardised between subjects. In vitro studies are useful because testing conditions such as applied forces can be fixed for all specimens. During in vivo studies, individual variables such as patient efforts and body habitus can alter the range of cervical spine motion. On the other hand, testing conditions of in vitro studies may not accurately reflect applied in vivo forces and motions in real life. Nonetheless, the segmental motion fraction is unlikely to be affected as it represents a percentage of contribution of a motion segment towards the motion of the entire cervical spine.
We acknowledge that successful preservation of range and quality of motion at an average of 16.2 months after the surgery constitutes a relatively short period of follow-up. An additional 5 to 10 years of follow-up, at a minimum, is necessary to evaluate the performance of this cervical disc arthroplasty device. Lastly, it would have been interesting to perform the above fluoroscopic imaging prior to the cervical arthroplasty operation for direct comparison with the post-operative findings, or to compare the findings to another artificial cervical disc device.
Conclusions
Our results showed physiologic motion quality at the index segment and harmony among neighbouring segments following cervical disc arthroplasty using a M6-C device, without gearshift-like intermittent locking of the prosthesis during the arc of flexion-extension motion. The implanted levels showed small variability in segmental motion fractions over the arc of flexion-extension motion. The study also showed that the flexible, non-constrained properties of the prosthesis allow near-physiological shift of COR and motion pattern. This pilot study demonstrated the feasibility of investigating cervical disc prostheses using the novel concept of segmental motion fraction as a motion-quality metric. Further long-term studies with larger sample size are required to draw solid conclusions.
Acknowledgments
Funding: None.
Footnote
Reporting Checklist: The authors have completed the STROBE reporting checklist. Available at https://jss.amegroups.com/article/view/10.21037/jss-22-62/rc
Data Sharing Statement: Available at https://jss.amegroups.com/article/view/10.21037/jss-22-62/dss
Peer Review File: Available at https://jss.amegroups.com/article/view/10.21037/jss-22-62/prf
Conflicts of Interest: All authors have completed the ICMJE uniform disclosure form (available at https://jss.amegroups.com/article/view/10.21037/jss-22-62/coif). The authors have no conflicts of interest to declare.
Ethical Statement: The authors are accountable for all aspects of the work in ensuring that questions related to the accuracy or integrity of any part of the work are appropriately investigated and resolved. The study was conducted in accordance with the Declaration of Helsinki (as revised in 2013). The study was approved by the Tasmania Health and Medical Human Research Ethics Committee of the University of Tasmania (No. 20264) and informed consent was provided by all individual participants.
Open Access Statement: This is an Open Access article distributed in accordance with the Creative Commons Attribution-NonCommercial-NoDerivs 4.0 International License (CC BY-NC-ND 4.0), which permits the non-commercial replication and distribution of the article with the strict proviso that no changes or edits are made and the original work is properly cited (including links to both the formal publication through the relevant DOI and the license). See: https://creativecommons.org/licenses/by-nc-nd/4.0/.
References
- Pham M, Phan K, Teng I, et al. Comparative Study Between M6-C and Mobi-C Cervical Artificial Disc Replacement: Biomechanical Outcomes and Comparison with Normative Data. Orthop Surg 2018;10:84-8. [Crossref] [PubMed]
- Kim SW, Paik SH, Castro PA, et al. Analysis of factors that may influence range of motion after cervical disc arthroplasty. Spine J 2010;10:683-8. [Crossref] [PubMed]
- Chen Y, Yuan W, Wu X, et al. The effect of range of motion after single-level discover cervical artificial disk replacement. J Spinal Disord Tech 2013;26:E158-62. [Crossref] [PubMed]
- Boselie TF, van Mameren H, de Bie RA, et al. Cervical spine kinematics after anterior cervical discectomy with or without implantation of a mobile cervical disc prosthesis; an RCT. BMC Musculoskelet Disord 2015;16:34. [Crossref] [PubMed]
- Patwardhan AG, Havey RM. Prosthesis design influences segmental contribution to total cervical motion after cervical disc arthroplasty. Eur Spine J 2020;29:2713-21. [Crossref] [PubMed]
- Lauryssen C, Coric D, Dimmig T, et al. Cervical total disc replacement using a novel compressible prosthesis: Results from a prospective Food and Drug Administration-regulated feasibility study with 24-month follow-up. Int J Spine Surg 2012;6:71-7. [Crossref] [PubMed]
- Thomas S, Willems K, Van den Daelen L, et al. The M6-C Cervical Disk Prosthesis: First Clinical Experience in 33 Patients. Clin Spine Surg 2016;29:E182-7. [Crossref] [PubMed]
- Reyes-Sanchez A, Miramontes V, Olivarez LM, et al. Initial clinical experience with a next-generation artificial disc for the treatment of symptomatic degenerative cervical radiculopathy. SAS J 2010;4:9-15. [Crossref] [PubMed]
- Phillips FM, Coric D, Sasso R, et al. Prospective, multicenter clinical trial comparing M6-C compressible six degrees of freedom cervical disc with anterior cervical discectomy and fusion for the treatment of single-level degenerative cervical radiculopathy: 2-year results of an FDA investigational device exemption study. Spine J 2021;21:239-52. [Crossref] [PubMed]
- Hui N, Phan K, Kerferd J, et al. Comparison of M6-C and Mobi-C cervical total disc replacement for cervical degenerative disc disease in adults. J Spine Surg 2019;5:393-403. [Crossref] [PubMed]
- Wu SK, Kuo LC, Lan HC, et al. Segmental percentage contributions of cervical spine during different motion ranges of flexion and extension. J Spinal Disord Tech 2010;23:278-84. [Crossref] [PubMed]
- Auerbach JD, Anakwenze OA, Milby AH, et al. Segmental contribution toward total cervical range of motion: a comparison of cervical disc arthroplasty and fusion. Spine (Phila Pa 1976) 2011;36:E1593-9. [Crossref] [PubMed]
- Penning L. Differences in anatomy, motion, development and aging of the upper and lower cervical disk segments. Clin Biomech (Bristol, Avon) 1988;3:37-47. [Crossref] [PubMed]
- Anderst W, Baillargeon E, Donaldson W, et al. Motion path of the instant center of rotation in the cervical spine during in vivo dynamic flexion-extension: implications for artificial disc design and evaluation of motion quality after arthrodesis. Spine (Phila Pa 1976) 2013;38:E594-601. [Crossref] [PubMed]
- Kowalczyk I, Lazaro BC, Fink M, et al. Analysis of in vivo kinematics of 3 different cervical devices: Bryan disc, ProDisc-C, and Prestige LP disc. J Neurosurg Spine 2011;15:630-5. [Crossref] [PubMed]
- Muhlbauer M, Tomasch E, Sinz W, et al. In cervical arthroplasty, only prosthesis with flexible biomechanical properties should be used for achieving a near-physiological motion pattern. J Orthop Surg Res 2020;15:391. [Crossref] [PubMed]
- Koller H, Meier O, Zenner J, et al. In vivo analysis of cervical kinematics after implantation of a minimally constrained cervical artificial disc replacement. Eur Spine J 2013;22:747-58. [Crossref] [PubMed]
- Guo Z, Cui W, Sang DC, et al. Clinical Relevance of Cervical Kinematic Quality Parameters in Planar Movement. Orthop Surg 2019;11:167-75. [Crossref] [PubMed]
- Powell JW, Sasso RC, Metcalf NH, et al. Quality of spinal motion with cervical disk arthroplasty: computer-aided radiographic analysis. J Spinal Disord Tech 2010;23:89-95. [Crossref] [PubMed]
- Reitman CA, Hipp JA, Nguyen L, et al. Changes in segmental intervertebral motion adjacent to cervical arthrodesis: a prospective study. Spine (Phila Pa 1976) 2004;29:E221-6. [Crossref] [PubMed]
- Sears WR, Duggal N, Sekhon LH, et al. Segmental malalignment with the Bryan cervical disc prosthesis--contributing factors. J Spinal Disord Tech 2007;20:111-7. [Crossref] [PubMed]
- Ghiselli G, Wharton N, Hipp JA, et al. Prospective analysis of imaging prediction of pseudarthrosis after anterior cervical discectomy and fusion: computed tomography versus flexion-extension motion analysis with intraoperative correlation. Spine (Phila Pa 1976) 2011;36:463-8. [Crossref] [PubMed]
- Taylor M, Hipp JA, Gertzbein SD, et al. Observer agreement in assessing flexion-extension X-rays of the cervical spine, with and without the use of quantitative measurements of intervertebral motion. Spine J 2007;7:654-8. [Crossref] [PubMed]
- Guyer RD, Coric D, Nunley PD, et al. Cervical Total Disk Replacement: Available Implant Size Matters. Clin Spine Surg 2022;35:166-9. [Crossref] [PubMed]
- Wang XF, Meng Y, Liu H, et al. The impact of different artificial disc heights during total cervical disc replacement: an in vitro biomechanical study. J Orthop Surg Res 2021;16:12. [Crossref] [PubMed]
- Thaler M, Hartmann S, Gstöttner M, et al. Footprint mismatch in total cervical disc arthroplasty. Eur Spine J 2013;22:759-65. [Crossref] [PubMed]
- Dong L, Tan MS, Yan QH, et al. Footprint mismatch of cervical disc prostheses with Chinese cervical anatomic dimensions. Chin Med J (Engl) 2015;128:197-202. [Crossref] [PubMed]
- Anderst WJ, Donaldson WF 3rd, Lee JY, et al. Cervical motion segment percent contributions to flexion-extension during continuous functional movement in control subjects and arthrodesis patients. Spine (Phila Pa 1976) 2013;38:E533-9. [Crossref] [PubMed]
- Sears WR, McCombe PF, Sasso RC. editors. Kinematics of cervical and lumbar total disc replacement. Seminars in Spine Surgery. Elsevier; 2006.
- Holmes A, Wang C, Han ZH, et al. The range and nature of flexion-extension motion in the cervical spine. Spine (Phila Pa 1976) 1994;19:2505-10. [Crossref] [PubMed]
- Patwardhan AG, Tzermiadianos MN, Tsitsopoulos PP, et al. Primary and coupled motions after cervical total disc replacement using a compressible six-degree-of-freedom prosthesis. Eur Spine J 2012;21:S618-29. [Crossref] [PubMed]
- Liu B, Liu Z, VanHoof T, et al. Kinematic study of the relation between the instantaneous center of rotation and degenerative changes in the cervical intervertebral disc. Eur Spine J 2014;23:2307-13. [Crossref] [PubMed]
- Amevo B, Worth D, Bogduk N. Instantaneous axes of rotation of the typical cervical motion segments: a study in normal volunteers. Clin Biomech (Bristol, Avon) 1991;6:111-7. [Crossref] [PubMed]
- Sang H, Cui W, Sang D, et al. How Center of Rotation Changes and What Affects These After Cervical Arthroplasty: A Systematic Review and Meta-analysis. World Neurosurg 2020;135:e702-9. [Crossref] [PubMed]
- Pickett GE, Rouleau JP, Duggal N. Kinematic analysis of the cervical spine following implantation of an artificial cervical disc. Spine (Phila Pa 1976) 2005;30:1949-54. [Crossref] [PubMed]
- Bogduk N, Mercer S. Biomechanics of the cervical spine. I: Normal kinematics. Clin Biomech (Bristol, Avon) 2000;15:633-48. [Crossref] [PubMed]